ABSTRACT
The structural, pasting, and dynamic rheological properties of starches isolated from six Indian wheat (Triticum aestivum L.) cultivars were evaluated. The amylose content of starches ranged between 18.35–20.58%; subsequently, these were normal-type starches. The light transmittance of gelatinized starch pastes decreased with increase in storage periods at 4°C. Scanning electron micrographs revealed bimodal size distribution of starch granules, with smaller B- and larger A-type. X-ray diffraction (XRD) pattern revealed typical A-type crystalline starch, with the highest crystalline index (CI) for cv.C-306. Fourier transform infrared (FTIR) spectroscopy was performed to understand the chemical bonding and short-range molecular order of starches. The highest 1047/1022 absorbance ratio (0.8666) for cv.C-306 justified its more crystalline nature. Peak viscosity (PV), trough viscosity (TV), and final viscosity (FV) were the highest for cv.WH-147 starch (1602, 1542, and 2123 cP, respectively). Peak G’, peak G”, and breakdown in G’ during heating were in the range of 5052–9067, 563–1463, and 909–3567 Pa, respectively. The power law model agreed well with the steady shear properties of starch pastes. Non-Newtonian fluid behaviour of starch was observed with n value ranging between 0.22 and 0.32. PV, FV, TV, and setback viscosity (SV) were significantly correlated to each other. Peak G’ exhibited negative correlation with TG’ (temperature at peak G’), light transmittance after 96 and 120h storage periods, and positively correlated with breakdown in G’. A significant positive correlation was observed between peak G” and tanδ during heating. Complex viscosity (η*) was also found positively correlated to G’.
Introduction
Wheat (Triticum aestivum L.) serves as one of the most important food crops because it is an important source of starch. Its grain quality depends on the starch characteristics and protein present. In 2014, approximately 729 metric tons of wheat was produced all over the world, with India producing about 94.48 metric tons.[Citation1] India ranks second after China in wheat-producing countries, followed by the Russian Federation.[Citation1] A huge amount of wheat grains is wasted every year in India due to insects, rodents, and climatic factors, so by converting them into starch on a large scale, this wastage may be reduced.
Starch is the major form and predominant source of stored carbohydrate present in cereal grains and it has attracted wide attention in relation to its physicochemical and structural properties.[Citation2] The mature endosperms of wheat, triticale (wheat-rye hybrid), and barley contain two types of starch granules: large, disc-shaped A-granules and small, spherical B-granules.[Citation3] Using confocal laser scanning microscopy, Kim and Huber[Citation4] observed channels throughout the wheat starch granules, which facilitate transfer of some molecules into the granule matrix. The pasting properties of wheat starch revealed that A-type granule starch showed higher peak, breakdown, final, and setback viscosities than B-type granules.[Citation5] The temperature and/or time during the heating of starch dispersions may be limiting factors for the pasting and swelling properties of starch granules. Rheological studies help to know about the molecular structure or distribution of the molecular components of foods and also the prediction and description of structural modifications of foods during manufacturing processes.[Citation6] Thus, the food rheology helps in providing important information for product development, quality control, sensory evaluation, and process designing. Crystalline index (CI) is a semiquantitative evaluation of long-range order within starch, determined using the ratio of diffraction intensities given by crystalline (ordered pattern) parts relative to the total diffraction pattern. X-ray diffractogaphs of starches from waxy and normal wheat cultivars showed typical A-type diffraction patterns.[Citation7] Fourier transform infrared (FTIR) spectroscopy is performed to understand the location and nature of chemical bonds that already existed, newly formed ones, and those broken between different atoms of starch amylose and amylopectin fraction. This technique also helps one to know the short-range molecular order of starches, from which crystallinity and the double helices structure can be estimated.
The aim of the present study was to investigate the interrelationships between the different physicochemical, pasting, rheological, and crystalline properties of wheat starches. By knowing the relationship between the properties studied, we can alter the quality of wheat starch to a desirable extent, which can be beneficial for the final product formed from it.
Materials and methods
Materials
Six wheat cultivars, i.e. PBW-502 and PBW-343 from Punjab, India, and cultivars PBW-373, C-306, WH-147, and WH-1025 from Haryana, India, were procured for isolation of starch. Starch was isolated from these cultivars using the method described by Wolf.[Citation8]
Amylose content, swelling power, and solubility
Amylose content was determined using the method described by Williams et al.[Citation9] using a UV-Vis spectrophotometer (Shimadzu, UV-1800, Japan). Swelling power and solubility were determined by following the procedure described by Leach et al.[Citation10]
Light transmittance
Light transmittance of starch pastes was measured following the method described by Perera and Hoover[Citation11] using a UV-Vis spectrophotometer (Shimadzu, UV-1800, Japan).
Scanning electron microscopy
Scanning electron micrographs were obtained at 1000 × (1000 times magnified) with a scanning electron microscope (Model EVO-LS10 ZEISS, Oberkochen, Germany) by following the method described by Wani et al.[Citation12]
X-ray diffraction
X-ray diffractograms of starch samples were recorded using an analytical X-ray diffractometer (Rigaku MiniFlex, Japan) having a CuKα source, with a wavelength of λ = 1.54 Å operating at 45 kV and 40 mA. X-ray diffractograms were obtained at 25°C in the 2θ angle range of 4–30° with a step size of 0.02 and 10 sec scan speed. The MS excel data was exported to OriginPro 8E (OriginLab, USA) software for graph creation. CI was calculated by the peak height method developed by Segal et al.[Citation13] and d-spacing values were calculated according to the method described by Zhang et al.[Citation14] using Eq. (1):
where n = 1 and λ = 1.54 Å.
FTIR spectroscopy
The FTIR spectrum was recorded on a Fourier transform spectrophotometer (PerkinElmer FTIR-C92035, USA) in the range 400–4000 cm−1 using KBr as the medium. The MS excel data was exported to OriginPro, 8E (OriginLab, USA) software for graph creation. The absorbance ratios at 1047/1022 and 1022/995 cm−1 were obtained from the deconvoluted FTIR spectra from wave numbers 800 to 1200 cm−1.
Pasting properties
The pasting properties of the starches were measured using a starch cell of Modular Compact Rheometer (Anton Paar, MCR-52, Austria) using the method described by Kaur and Singh.[Citation15]
Dynamic rheological properties
Dynamic rheological properties were determined with the method described by Sandhu et al.[Citation16] Steady shear properties were determined using the method described by Park et al.[Citation17] with slight modifications. To describe the variation in the rheological properties of the samples under steady shear, the data were fitted to the well-known power law (Eqn. 2).
where σ is the shear stress (Pa), γ is the shear rate (s−1), K is the consistency index (Pa.sn), and n is the flow behaviour index (dimensionless), which was obtained from the linear regression of the square roots of shear rate–shear stress data.
Statistical analysis
The data reported in all tables are averages of triplicate observations. The averages, one- and two-way ANOVA, Least Significant Difference (LSD), and Honest Significant Difference (HSD) (Tukey’s test) were computed to measure variations in the observations with the help of MS-excel and Minitab 14. Correlations among different parameters were also computed with the help of MS-excel. The superscripts *, **, and *** (values given in text and data not shown in the form of correlation table) represent the level of significance at p ≤ 0.05, 0.01, and 0.001, respectively.
Results and discussion
Physicochemical properties
The physicochemical properties of starches from different wheat cultivars are shown in . The amylose content of starches ranged from 18.35 to 20.58%, and the lowest and the highest powers were shown by cv.C-306 and cv.PBW-502, respectively. The amounts of amylose and amylopectin starch components have a profound effect on the various properties of starch materials such as swelling power and microscopic properties.[Citation18] The cv.WH-147 showed the lowest and cv.PBW-502 had the highest swelling power. Starch gelatinization process breaks down the intermolecular bonds of starch molecules in the presence of water and heat, thus subsequently engaging more water to the hydrogen bonding sites (hydroxyl hydrogen and oxygen).[Citation19] The solubility of different starches ranged from 6.48 to 11.98%. Solubility is the amount of starch leached out into the supernatant in swelling power determination.[Citation20] Kaur et al.[Citation21] also observed the swelling power and solubility of 9.8 g/g and 12.9%, respectively, for starch from an Indian wheat cultivar.
Table 1. Amylose content, swelling power, and solubility of starches from different wheat cultivars.
The light transmittance of starch pastes decreased with storage periods at 4°C from 0 to 120 h for different cultivars (). The results revealed significant (p ≤ 0.05) difference in light transmittance among starch pastes from different cultivars. Light transmittance of starch pastes ranged between 11.30–15.13% and 1.27–2.04% at 0 and 120 h of storage periods, respectively. Light transmittance after 48, 72, 96, and 120 h of storage at 4°C showed positive correlations with swelling power (r = 0.93**, 0.90*, 0.83*, and 0.82*, respectively). Starch paste from cv.WH-147 showed the lowest and that from cv.PBW-502 had the highest light transmittance for all storage periods studied except for 0 h. The period of storage showed a positive relationship among each other for light transmittance. The decrease in light transmittance values with increase in storage time may be due to starch retrogradation and subsequently the re-association of broken bonds in the ordered structure of starch.[Citation12] Perera and Hoover[Citation11] reported that amylose and amylopectin leached out due to swelling in granules and their chain lengths, ultimately leading to increased turbidity and decreased light transmittance in starch gels during refrigerated storage.
Table 2. Light transmittance (%) property of starches isolated from different wheat cultivars.
Scanning electron microscopy
SEM helps to understand the relationship between granules morphology and starch genotype.[Citation22] The scanning electron micrographs revealed three distinct category sizes of starch granules in wheat cultivars, i.e. small, medium, and large (). These sizes of small, medium, and large granules were in the range of 5.9–8.2 µm, 14.6–20.9 µm, and 27.7–31.0 µm, respectively (data not shown). These are classified into two category sizes, granule size in the ranges 5.9–14.6 µm (B-type) and 14.6–31.0 µm (A-type). Generally wheat contains bimodal size distribution of starch granule populations and has two types of size groups, i.e. small granules (below 10–14 µm) and large granules (above 10–14 µm to 36 µm). Starch granules from cv.PBW-343 showed smaller-sized granules, whereas those from cv.WH-147 had larger-sized granules. The larger A-type wheat starch granules were generally disc like or lenticular and the smaller B-type granules were spherical and somewhat polygonal in shape. Similar spherical and irregular shapes for B-type starch granules were also observed by Zhang et al.[Citation14] SEM also revealed that some starch granules were not intact and they were broken into half or more divisions. The damaged starch amount depends on the severity of grinding and the hardness of wheat grains.[Citation23]
X-ray diffraction
Starch granule is a semicrystalline system, containing crystalline and amorphous regions. For a specific type of starch, a specific XRD pattern was obtained and each type of crystalline structure of starch has its own XRD pattern (). The crystalline parts of starch always show sharp peaks, whereas the amorphous parts have dispersive peaks.[Citation24] All starches under study had a typical A-type crystalline structure with the main diffraction peaks at around 15, 17, 18, 20, and 23° (2θ) angle. However, in PBW-373, WH-1025, and PBW-343 cultivars, some peaks were absent. Diffraction spacing (d-spacing) values for the peaks obtained at different 2θ diffraction angles (°) and CI are reported in . The CI of starches from different cultivars ranged from 26.61 to 31.37%; cv.PBW-373 exhibited the lowest and cv.C-306 showed the highest value. In case of cv.PBW-373 peaks at around 15°, 17°; for cv.WH-1025 peaks at around 20°; and for cv.PBW 343 peaks at around 18° at 2θ were lacking, and hence there exists no d-spacing value, which supports the lower CI values for starches from these cultivars. The results were in line with those reported by Zhang et al.[Citation14] The d-spacing values observed on different peaks ranging from 5.91 to 3.86 Å also indicate the crystallinity of starches.
Table 3. Structural characteristics of different wheat cultivar starches as determined by XRD and FTIR.
FTIR spectroscopy
The FTIR patterns of starches from different cultivars were studied to understand their molecular nature (). The characteristic broad bands at 3392.3–3416.2 cm−1 and 2930.2–2931.3 cm−1 are attributed to the vibration of O-H stretching due to hydrogen-bonded hydroxyl groups and the C-H deformation of the glucose unit, respectively. The absorbance at 1638.9–1644.1 cm−1 was due to the bending vibration of O-H of water absorbed in the amorphous regions of starch. Three characteristic peaks were assigned to the vibration of C-O stretching observed between 1019–1020, 1080.1–1080.5, and 1155.6–1156.7 cm−1. The peaks at 1019–1020 and 1080.1–1080.5 cm−1 were attributed to the vibration of C-H and C-O-H bending, respectively. No clear differences were observed between starches from different wheat cultivars. Further bends at 439.2–440.4, 526.3–528.1, 575.4–575.8, 708.1–709.4, 764.1–764.9, 860.9–861.5, and 930.1–930.5 cm−1 were attributed to skeletal vibrations of the pyranoid ring. Similar results were reiterated by Smrckova et al.[Citation25] and Wang et al.[Citation26] The peak around 1015 cm−1 was due to the C–O of C–O–C in polysaccharide; the peaks close to 1081 and 1160 cm−1 were associated with anhydro-glucose ring C–O stretch; the peak near 930 cm−1 was assigned to the skeletal mode vibration of α-(1–4) glycosidic linkage[Citation27,Citation28]; and the peak near 860 cm−1 was because of the C–H and CH2 deformations.[Citation28] To investigate the short-range molecular order of starches from different cultivars, deconvoluted FTIR spectra from wave numbers 800–1200 cm−1 are depicted in . The absorbance ratios of 1047/1022 and 1022/995 cm−1 ranged from 0.8312 to 0.8666 and 1.1115 to 1.1913, respectively (), where cv.C-306 showed the highest 1047/1022 cm−1 absorbance ratio, while cv.PBW-343 had the highest 1022/995 cm−1 absorbance ratio. The absorbance ratios of 1047/1022 and 1022/995 cm−1 are the indexes of the short-range order of double helices.[Citation29,Citation30] FTIR showed sensitive changes in structure at the molecular level and the IR bands at 1047 and 1022 cm−1. These are associated with the ordered and amorphous structure of starch, also reflected from the significant positive correlation (r = 0.86*) between CI and the 1047/1022 absorbance ratio. The 1022/995 absorbance ratio showed a negative correlation (r = −0.87*) with d-spacing values of XRD patterns at about 23° at the 2θ scale.
Pasting properties
Pasting properties of starches from different cultivars are presented in . Peak viscosity (PV) of starches ranged from 1180 to 1602 cP: cv.WH-147 showed the highest and cv.PBW-343 had the lowest value. The increase in viscosity is due to granule swelling. The amylose leaching, amylose lipid complex formation, and granule swelling affect the hot paste viscosity.[Citation31] Trough viscosity (TV) of starches varied from 1102 to 1542 cP; cv.WH-147 had the highest value and showed a positive correlation with PV (r = 0.93**). TV indicates a decrease in the viscosity of starch after PV due to the rupturing of swollen granules at high temperature.[Citation32] Breakdown viscosity (BV) (difference between PV and TV) of the studied starches ranged from 60 to 194 cP. It is attributed to the dissociation of gelatinized starch granule during continuous stirring and heating.[Citation33] BV of starches showed stability of pastes. BV was highly correlated (r = 0.98**) with the d-spacing values of XRD patterns at about 23° at the 2θ scale. Setback viscosity (SV) showed the retrogradation capacity of starch and varied from 344 to 618 cP. During the cooling of starch paste, leached amylose molecules are rapidly associated and the resultant amylose junction areas were responsible for SV.[Citation34] A rearrangement reaction between amylose and amylopectin occurred as the temperature decreased from 95 to 50°C; subsequently, the viscosity increased again to a final viscosity (FV), and the increment value was called SV.[Citation35] The FV of starches varied from 1468 to 2123 cP; the highest and lowest values were observed for WH-147 and PBW-343 cultivars, respectively. FV was positively correlated with large A-type granule size, peak, trough, and SV (r = 0.82*, 0.96**, 0.91*, and 0.86*, respectively). The FV of starches is influenced by the aggregation of amylose molecules[Citation36], whereas SV is the measure of gelling ability or the retrogradation tendency of starch.[Citation37] The pasting temperature of starches was between 84.8 and 87.8°C, where cv.C-306 showed the highest value; subsequently, its starch granules had higher resistance to swelling and rupture.
Rheological properties
Dynamic rheological properties
The rheological properties of different starches during heating are shown in . In and the changes in elastic or storage modulus (peak Gʹ) and viscous or loss modulus (peak Gʹʹ) of starches during heating are shown. Peak Gʹ values were much higher than peak Gʹʹ values for all starches under study. Peak Gʹ varied from 5052 to 9067 Pa (Pascal) for different cultivar starches. Peak Gʹʹ ranged between 563 and 1463 Pa, where cv.PBW-343 showed the lowest value and cv.PBW-502 had the highest value. Peak Gʹ was negatively correlated with light transmittance after 96 and 120 h of storage duration (r = −0.83* and −0.82*, respectively). Peak Gʹ is the measure of stored energy in the material and recovered from it per cycle whereas peak Gʺ is the measure of energy dissipated or lost per cycle during sinusoidal deformation.[Citation38] Breakdown in Gʹ ranged from 909 to 3567 Pa; the highest was for cv.PBW-373 and the lowest value was observed for cv.PBW-502. Breakdown in Gʹ exhibited significant positive correlations with peak Gʹ (r = 0.92**). The ratio of the energy lost to the energy stored for each cycle can be defined by tan δ, which is another parameter indicating the physical behaviour of a system. tan δ varied from 0.10 to 0.29 for different starches, where the highest tan δ was observed for cv.PBW-502. tan δ approaches unity when the degree of elastic and viscous behaviour of a material is same. Lower values of tan δ (<1) indicate more elastic material with solid-like behaviour, while higher values of tan δ (>1) describe the viscous behaviour and the material is more like a liquid in nature.[Citation39] tan δ showed positive correlation with peak Gʹʹ (r = 0.85*). TGʹ varied from 77.57 to 85.06°C; cv.PBW-502 had the highest value and was negatively correlated with peak Gʹ (r = −0.93**). It can also be observed from that Gʹ of the starches progressively increased to a maximum (peak Gʹ) at various temperatures (TGʹ) and then decreased with continued heating. Similar findings were observed for Gʺ with a predominance of Gʹ over Gʺ, indicating viscoelastic behaviour (). Many applications of polymers depend on their viscoelastic properties. The swelling of starch granules to fill all available volume of the system is related to the formation of the three-dimensional network of swollen granules, which occurs due to the intergranular contact required for the initial increase in Gʹ.[Citation40]
Table 4. Dynamic rheological properties of starches isolated from different wheat cultivars.
Figure 5. (A): Changes in G’ for wheat cultivar starches during heating. (B): Changes in G” for starches during heating. Here: (A) C-306, (B) PBW-373, (C) WH-147, (D) WH-1025, (E) PBW-343, and (F) PBW-502.
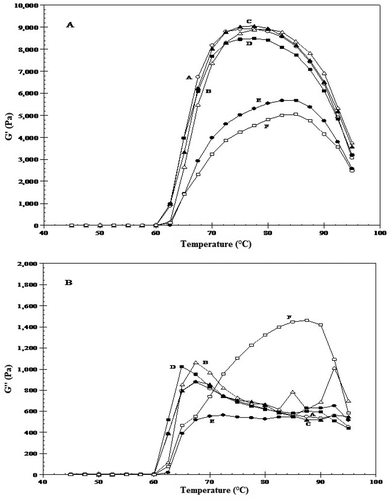
The frequency dependencies of the storage modulus (Gʹ) and loss modulus (Gʹʹ) provide significantly valuable information about the gel structure.[Citation41] In addition to Gʹ and Gʹʹ, the loss tangent (Gʹʹ/Gʹ) was observed to reflect the dynamic elastic nature of gels, indicating the relative measure of the associated energy loss versus the energy stored per deformation cycle.[Citation42] The Gʹ value of starches during frequency sweep test measured at 6.28 rad/sec varied from 5685 to 7370 Pa; the highest and lowest values were observed for cv.PBW-373 and cv.C-306, respectively (, , , and ). Gʹ exhibited a positive correlation (r = 0.81*) with solubility and negatively correlated with CI and the 1047/1022 cm−1 absorbance ratio (r = −0.82* and −0.89*, respectively). The Gʹ value of all starches under study showed a higher value compared to the Gʹʹ value; this denotes that these starches were elastic in nature. Gʹʹ varied from 290 to 360 Pa, where cv.WH-147 reported the lowest and cv.PBW-373 showed the highest value. Gʹʹ was positively correlated with swelling power and solubility (r = 0.82* and 0.81*, respectively).The tan δ values were 0.05 for all, except cv.C-306. Complex viscosity (η*) ranged from 906 to 1174 Pa s, where C-306 and PBW-373 cultivars showed the lowest and highest values, respectively. Complex viscosity (η*) was positively correlated with Gʹ and solubility (r = 1.00*** and 0.81*, respectively). Complex viscosity (η*) also exhibited a negative correlation with CI (r = −0.82*).
Steady shear rheological properties
shows the steady shear values of different starches at 25°C. The experimental data of flow behaviour for different starch pastes were fitted to the power law and the consistency coefficient (K), flow behaviour indices (n), and coefficients of determination (R2) were evaluated. K ranged between 33.44 and 62.03 Pa s, where cv.C-306 showed the lowest and cv.PBW-502 had the highest value. The power law accurately described the flow behaviour of starch pastes. K showed viscousness, n, and R2 values for each flow curve. The n value for different starches varied from 0.22 to 0.32, and hence the starch paste formed show thinning behaviour upon shearing and is a non-Newtonian fluid. If n = 1 the paste shows Newtonian fluid behaviour and if n > 1, the paste would be described as having a thickening behaviour. All the R2 values for the regression equations were above 0.98, so they revealed best fit of power law results. K from the power law model can also be used as a criterion of viscosity.[Citation43] Okechukwu and Rao[Citation44] reported that shear-thickening or shear-thinning behaviour is related to the rupture of swollen granules.
Table 5. The power law function parameters of different wheat cultivar starches.
Conclusions
The correlation analysis provided valuable information about the mechanisms contributing to the functional, morphological, crystalline, pasting, and dynamic rheological properties of wheat starches. Significant correlations among the rheological and structural properties of different starches were observed. Cv.C-306 showed the lowest amylose content and solubility, but had the highest CI, 1047/1022 cm−1 absorbance ratio, d-spacing values for all its peaks at 2θ diffraction angles, and pasting temperature. Cv.WH-147 exhibited the highest PV, TV, and FV and the lowest BV and pasting temperature. The wheat cultivars chosen for study of their starch properties are mostly grown in the North Indian region. Study of the physicochemical, granule morphology, crystalline, gelatinization, and retrogradation properties strongly influences the quality of wheat flour products. Subsequently it is very important to investigate various properties (physicochemical, structural, pasting, and rheological) of these starches with their relationships, so that applications in food industries are enhanced to a large extent, to design better processing procedures of various food products. Further research, however, needs to be performed on the modification of these starches so that they may be utilized for suitable applications in the food industry.
References
- FAO, 2014. Food and Agriculture Organization of the United Nations. http://faostat.fao.org (accessed November, 2016).
- Van Hung, P.; N. Morita, Physicochemical Properties of Hydroxypropylated and Cross-linked Starches from A-type and B-type Wheat Starch granules. Carbohydrate Polymers 2005, 59, 239–246.
- Ao, Z.; Jane, J. Characterization and Modeling of the A- and B-granule Starches of Wheat, Triticale, and Barley. Carbohydrate Polymers 2007, 67, 46–55.
- Kim, H.S.; Huber, K.C. Channels within Soft Wheat Starch A- and B-type Granules. Journal of Cereal Science 2008, 48, 159–172.
- Shinde, S.V.; Nelson, J.E.; Huber, K.C. Soft Wheat Starch Pasting Behaviour in Relation to A- and B-type Granule Content and Composition. Cereal Chemistry 2003, 80, 91–98.
- Koocheki, A.; Razavi, S.M. Effect of Concentration and Temperature on Flow Properties of Alyssum homolocarpum Seed Gum Solutions: Assessment of Time Dependency and Thixotropy. Food Biophysics 2009, 4, 353–364.
- Hayakawa, K.; Tanaka, T.; Nakamura, S.; Endo, T.; Hoshino, T. Quality Characteristics of Waxy Hexaploid Wheat (Triticum aestivum L.): Properties of Starch Gelatinization and Retrogradation. Cereal Chemistry 1997, 74, 576–580.
- Wolf, M.J. Wheat Starch Isolation. In Methods in Carbohydrates Chemistry; Wolform, R.L.; Eds.; Academic press: New York, 1964; 4–6.
- Williams, P.C.; Kuzina, F.D.; Hlynka, I. A Rapid Colorimetric Procedure for Estimating the Amylose Content of Starches and Flours. Cereal Chemistry 1970, 47, 411-–420.
- Leach, H.W.; Mc Cown, L.D.; Schoch, T.J. Structure of the Starch Granule I. Swelling and Solubility Patterns of Various Starches and Flours. Cereal Chemistry 1959, 36, 535–545.
- Perera, C.; Hoover, R. Influence of Hydroxypropylation on Retrogradation Properties of Native, Defatted and Heat-Moisture Treated Potato Starches. Food Chemistry 1999, 64, 361–375.
- Wani, I.A.; Sogi, D.S.; Wani, A.A.; Gill, B.S.; Shivhare, U.S. Physico-chemical Properties of Starches from Indian Kidney Bean (Phaseolus vulgaris) Cultivars. International Journal of Food Science and Technology 2010, 45, 2176–2185.
- Segal, L.; Creely, J.J.; Martin, A.E.; Conrad, C.M. An Empirical Method for Estimating the Degree of Crystallinity of Native Cellulose using the X-ray Diffractometer. Textile Research Journal 1962, 29, 786–794.
- Zhang, B.; Li, X.; Liu, J.; Xie, F.; Chen, L. Supramolecular Structure of A- and B-type Granules of Wheat Starch. Food Hydrocolloids 2013, 31, 68–73.
- Kaur, M.; Singh, S. Physicochemical, Morphological, Pasting and Rheological Properties of Tamarind (Tamarindus indica L.) Kernel Starch. International Journal of Food Properties 2016, 19, 2432–2442.
- Sandhu, K.S.; Singh, N.; Kaur, M. Characteristics of Different Corn Types and their Grain Fractions: Physicochemical, Thermal, Morphological, and Rheological Properties of Starches. Journal of Food Engineering 2004, 64(1), 119–127.
- Park, S.; Chung, M.G.; Yoo, B. Effect of Octenylsuccinylation on Rheological Properties of Corn Starch Pastes. Starch/Stärke 2004, 56, 399406.
- Hermansson, A.; Svegmark, K. Developments in the Understanding of Starch Functionality. Trends in Food Science and Technology 1996, 7, 345–353.
- Sobkowska, E.; Skrobia, I. Wspołczesna Wiedza o Węglowodanach (Contemporary Knowledge on Carbohydrates). In Poznan (in Polish); Gawecki, J.; Ed.; Wyd, Warszawa: AR, 2001.
- Singh, N.; Singh, J.; Kaur, L.; Sodhi, N.S.; Gill, B.S. Morphological, Thermal and Rheological Properties of Starches from Different Botanical Sources. Food Chemistry 2003, 81, 219–231.
- Kaur, M.; Oberoi, D.P.S.; Sogi, D.S.; Gill, B.S. Physicochemical, Morphological and Pasting Properties of Acid Treated Starches from Different Botanical Sources. Journal of Food Science and Technology 2011, 48, 460–465.
- Fannon, J.E.; Hauber, R.J.; BeMiller, J.N. Surface Pores of Starch Granules. Cereal Chemistry 1992a. 69, 284–288.
- Hoseney, R.C. Principle of Cereal Science and Technology. American Association of Cereal Chemists; AACC, St. Paul, MN, 1994.
- Gernat, C.; Tadosta, S.; Damaschun, G. Supramolecular Structure of Legume Starches Revealed by X-Ray Scattering. Starch/Stärke 1990, 42(5), 175–178.
- Smrckova, P.; Horsky, J.; Sarka, E.; Kolacek, J.; Netopilik, M.; Walterova, Z.; Krulis, Z.; Synytsya, A.; Hruskova, K. Hydrolysis of Wheat B-starch and Characterisation of Acetylated Maltodextrin. Carbohydrate Polymers 2013, 98, 43–49.
- Wang, S.; Wang, J.; Zhang, W.; Li, C.; Yu, J.; Wang, S. Molecular Order and Functional Properties of Starches from Three Waxy Wheat Varieties Grown in China. Food Chemistry 2015, 181, 43–50.
- Wang, J.; Su, L.; Wang, S. Physicochemical Properties of Octenyl Succinic Anhydride-Modified Potato Starch with Different Degrees of Substitution. Journal of the Science of Food and Agriculture 2010, 90(3), 424–429.
- Miao, M.; Li, R.; Jiang, B.; Cui, S.W.; Zhang, T.; Jin, Z. Structure and Physicochemical Properties of Octenyl Succinic Esters of Sugary Maize Soluble Starch and Waxy Maize Starch. Food Chemistry 2014, 151, 154–160.
- Sevenou, O.; Hill, S.E.; Farhat, I.A.; Mitchell, J.R. Organisation of the External Region of the Starch Granule as Determined by Infrared Spectroscopy. International Journal of Biological Macromolecules 2002, 31, 79–85.
- Shingel, K.I. Determination of Structural Peculiarities of Dexran, Pullulan and Irradiated Pullulan by Fourier-transform IR Spectroscopy. Carbohydrate Research 2002, 337, 1445–1451.
- Lu, S.; Chem, L.N.; Lii, C.Y. Correlations between the Five Structures, Physicochemical Preparation and Retrogradation of Amylopectins from Taiwan rice Varieties. Cereal Chemistry 1997, 74, 34–39.
- Andrabi, S.N.; Wani, I.A.; Gani, A.; Hamdani, A.M.; Masoodi, F.A. Comparative Study of Physico-Chemical and Functional Properties of Starch Extracted from Two Kidney Bean (Phaseolus vulgaris L.) and Green Gram Cultivars (Vigna radiata L.) Grown in India. Starch/Stärke 2015, 67, 1–11.
- Whistler, R.L.; BeMiller, J.N. Carbohydrate Chemistry for Food Scientists. American Association of Cereal Chemists; Eagan Press: St. Paul, MN, 1997; 117–151.
- Jane, J.; Chen, Y.Y.; Lee, L.F.; McPherson, A.E.; Wong, K.S.; Radosavljevic, M.; Kasemsuwan, T. Effects of Amylopectin Branch Chain Length and Amylose Content on the Gelatinization and Pasting Properties of Starch. Cereal Chemistry 1999, 76, 629–637.
- Chen, H.M.; Fu, X.; Luo, Z.G. Effect of Gum Arabic on Freeze-thaw Stability, Pasting and Rheological Properties of Tapioca Starch and its Derivatives. Food Hydrocolloids 2015, 51, 355–360.
- Blazek, J.; Copeland, L. Pasting and Swelling Properties of Wheat Flour and Starch in Relation to Amylose Content. Carbohydrate Polymers 2008, 71, 380–387.
- Shelton, D.R.; Lee, W.J. Food Science and Technology. In Cereal Carbohydrates; Kulp, K., J.G. Ponte; Eds.; CRC Press: Boca Raton, USA, 2000; 385–416.
- Ferry, J.D. Viscoelastic Properties of Polymers; (3rd ed.); John Wiley and Sons: New York, 1980; Chapter 2; 41–42.
- Lii, C.Y.; Chang, S.M.; Young, Y.L. Investigation of the Physical and Chemical Properties of Banana Starches. Journal of Food Science 1982, 47, 1493–1497.
- Eliasson, A.C. Viscoelastic Behaviour during the Gelatinization of Starch. I. Comparison of Wheat, Maize, Potato and Waxy-barley Starches. Journal of Texture Studies 1986, 17, 253–265.
- Kaur, L.; Singh, J.; Singh, H.; McCarthy, O.J. Starch-cassia Gum Interactions: A Microstructure-Rheology study. Food Chemistry 2008, 111, 1-–10.
- Toker, O.S.; Dogan, M.; Caniyilmaz, E.; Ersoz, N.B.; Kaya, Y. The Effects of Different Gums and their Interactions on the Rheological Properties of a Dairy Dessert: A Mixture Design Approach. Food and Bioprocess Technology 2013, 6, 896–908.
- Sikora, M.; Kowalski, S.; Tomasik, P.; Sady, M. Rheological and Sensory Properties of Dessert Sauces Thickened by Starch–Xanthan Gum Combinations. Journal of Food Engineering 2007, 79(4), 1144–1151.
- Okechukwu, P.E.; Rao, M.A. Influence of Granule Size on Viscosity of Corn Starch Suspension. Journal of Texture Studies 1995, 26, 501–516.