ABSTRACT
Volatile compounds in the three Longjing tea were analyzed by gas chromatography–mass spectrometry with method of solid phase microextraction–gas chromatography. Furthermore, aroma-active compounds of three Longjing tea were analyzed by gas chromatography–olfactometry and odor activity value. However, the kinds of aroma-active compounds identified from those two methods were different. The results showed that dihydroactinidiolide (aroma intensity = 9.1–9.2), (Z)-nerolidol (aroma intensity = 8.4–8.8), benzyl alcohol (aroma intensity = 8.1–8.4), and epoxylinalool (aroma intensity = 8.1–8.5) presented the highest aroma intensity in three green tea samples. While geraniol (422.4–2508.3), epoxylinalool (601.5–1033.9), (Z)-nerolidol (235.5–559.3), 2-ethyl-3,5-dimethylpyrazine (328.0–420.6), β-ionone (227.7–387.3) presented higher odor activity values than other compounds. Thus, this study uses recombination aroma models to identify selected important aroma-active compounds from gas chromatography–olfactometry and odor activity value and verifies their effectiveness to identify the aroma profile characterization of three Longjing tea samples using electronic nose analysis and sensory evaluation. The radar plot of electronic nose analysis tallied with sensory evaluation indicated that the odor activity value–based aroma models were more similar to the original Longjing tea aroma than gas chromatography–olfactometry–based models.
Introduction
Nowadays, tea (Camellia sinensis, Theaceae) is one of the most popular consumed beverages in the world which ranks at a level of being the second nonalcoholic drink after that of water.[Citation1] Tea comprises complicated chemical composition that varies with various parameters, such as variety, plucking season, soil, fertilization, climate, and post-harvested treatment.[Citation2] Longjing tea, also known as West-lake dragon-well tea, is a type of flatten-shaped Chinese green tea. It is regarded as the national tea in China and is renowned for its high quality, long history, and rich cultural connotations. The volatile compounds in Longjing tea have been identified by many researchers.[Citation3–Citation5] Although more than 600 volatile compounds have been identified through extensive research on green tea,[Citation6] few systematic investigations have been made to verify of the active-aroma compounds in Longjing tea. Gas chromatography–olfactometry and odor activity value (OAV) were extensively employed to investigate the aroma-active compounds of foods.[Citation7–Citation10] However, the effectiveness of those two methods to identify the key aroma-active compounds was different due to the difference of thresholds in air and water. Thus, the objectives of the present study were (a) to identify the aroma-active compounds in Longjing tea by OAV and GC-O, and (b) to compare their effectiveness to identify the aroma profile in Longjing tea by electronic nose analysis (ENA) and sensory analysis.
Materials and methods
Chemicals and materials
Pure authentic standards of dimethyl sulfide, 2,3-butanedione, 2,3-pentanedione, hexanal, 2-methyl-2-butenal, 4-methyl-3-penten-2-one, 1-penten-3-ol, β-myrcene, heptanal, 1-pentanol, 2-methylpyrazine, 3-hydroxy-2-butanone, 2,5-dimethylpyrazine, 2,6-dimethylpyrazine, 2-ethylpyrazine, 6-methyl-5-hepten-2-one, 1-hexanol, 2-ethyl-5-methylpyrazine, nonanal, trimethylpyrazine, 2-ethyl-3,5-dimethylpyrazine, linalool oxide, 2-ethyl hexanol, (E,E)-2,4-heptadienal, 2-acetylfuran, benzaldehyde, linalool, 1-octanol, 5-methyl-2-furfural, 2-acetyl pyridine, hotrienol, β-cyclocitral, safranal, 3-hexenyl hexanoate, furfurol, α-terpineol, methyl salicylate, geraniol, benzyl alcohol, phenylethyl alcohol, β-ionone, (Z)-jasmone, maltol, 2-acetylpyrrole, phenol, (Z)-nerolidol, p-cresol, cedrenol, 3-hexen-1-ol benzoate, 2-methoxy-4-vinylphenol, α-cadinol, methyl anthranilate, methyl jasmonate, dihydroactinidiolide, 4-vinylphenol, indole, coumarin were purchased from Adamas-beta (Shanghai, China). Standard linear alkanes (C6–C30) were purchased from Sigma-Aldrich Corporation (Shanghai, China). Dichloromethane and anhydrous sodium sulfate were purchased from Sinopharm Chemical Reagent Co. Ltd. (Shanghai, China). 1,3-Dichlorobenzene was used as internal standard, which was purchased from Shanghai Anpu Chemical Reagent Co. Ltd.
A total of three samples of Longjing tea were analyzed in the present study. One (Y1) was collected from local market in Hangzhou on March 26, 2016, before Qingming Festival (April 4). Other two samples were collected in Qiantang (Y2) and Yuezhou (Y3) on April 2, 2017 and April 4, 2017, respectively. The tea samples were considered as first grade with one bud and one leaf exhibition. The appearance of tea samples was flat and bright. Then, the samples were processed in local factory with traditional technology. All samples were kept in their own packages with aluminum foil material and stored in a refrigerator (4°C) until analyzed.
Sample preparation and solid-phase micro-extraction absorption of aroma compounds
Thirty grams of Longjing tea were placed in a pre-warmed tea pot and 800 mL of 80°C distilled water was added. The tea was brewed for 8 min. Then, the tea was poured through a porcelain strainer into a pre-warmed porcelain bowl. In order to minimize the loss of aroma compounds, the tea infusion (5 g) and 0.1 g of 1,3-dichlorobenzene (100 mg/kg) was placed in a 20 mL vial, which was sealed with Teflon® covers and equilibrated at 80°C on a water bath with shaking at regular intervals while being exposed to solid-phase micro-extraction (SPME) fiber in the sample headspace. After reaching equilibrium, the SPME fiber was withdrawn and directly introduced to the GC injector at 250°C for 3 min. The conditions of SPME fibers (65 μm PDMS/DVB, 80 μm PA, 100 μm PDMS, 75 μm CAR/PDMS, and 50/30 μm CAR-PDMS-DVB) and extraction times (5, 10, 15, 20, 25, 30 min) were evaluated.
Calibration of standard curves
Natural products contained a large number of substrates, including polysaccharide, protein, starch, phenol, and other macromolecular compounds: these substrates, with their space structure, were prone to interact with volatile compounds by physico-chemical action, which affected the release of compounds in natural products.[Citation11,Citation12] Thus, a specific matrix must be prepared to improve the accuracy of assessed concentrations of volatile compounds in natural products. To obtain a matrix similar to that of tea infusion, model solution was prepared containing 40 mg/g caffeine, 40 mg/g (+)-catechin (C), 10 mg/g (–)-epicatechin (EC), 30 mg/g (–)-epicatechin gallate (ECG), 10 mg/g (–)-epigallocatechin (EGC), 70 mg/g (–)-epigallocatechin gallate (EGCG), 4 mg/g His, 10 mg/g Glu, 5 mg/g Val, and 0.5 mg/g Val in Milli-Q deionized water.[Citation13,Citation14] A standard stock solution was prepared, using all of the volatile compounds, in Milli-Q deionized water. The standard stock solution was diluted in the following ratios: 1:5, 1:10, 1:20, 1:30, 1:40, and 1:50, respectively. Then, 0.1 g of 1,3-dichlorobenzene (100 mg/kg) was added, as an internal standard, to 5 g of model solution in a 20 mL vial, which was extracted by SPME. The optimal conditions were employed, as was identical to the tea infusions. The calibration curves were established to calculate the concentrations of volatile compounds in model solution. Each point was repeated six times.
GC-O analysis of aroma compounds
The GC separation consisted of an Agilent 7890A chromatograph equipped with a flame ionization detector (FID) and an ODP-2 Olfactory Detector Port (Gerstel, Mulheim an der Ruhr, Germany). This system allowed us to simultaneously obtain an FID signal for the quantification, and the odor characteristics of each compound were detected by sniffing port. GC effluent was split 1:1 among the FID and sniffing port. Samples were separated on the HP-5MS (60 m length × 0.25 mm i.d. × 0.25 μm thickness; J&W Scientific, Folsom, CA, USA) columns. The flow rate of carrier gas (nitrogen) was 2 mL/min; the oven temperature was first increased from 40°C (6 min), ramped at 3°C/min to 100°C, and then ramped at 5°C/min to 230°C (10 min); the injector and FID detector temperatures were set at 250 and 280°C, respectively. A glass sniffing port was heated by a ribbon heater (250°C). Moist air was pumped into the sniffing port at 50 mL/min to quickly remove the odorant eluted from sniffing port. The hydrogen, air, and nitrogen (makeup) flow rates were 30, 400, and 25 mL/min, respectively. A panel of three assessors evaluated the effluents. All the assessors were previously trained to recognize, describe, and discriminate different aroma compounds. Each of them completed 150 h of specific training with tea infusions. Then, the assessors responded and recorded the retention time, aroma intensity (AI), and descriptor of the aroma compounds. The odor intensities were rated on the following scale from 0 (undetected), 1 (weak), 5 (moderate), 8 (strong), 10 (extreme strong); half values were allowed. The experiment was replicated in triplicate by each assessor. Finally, the AI was averaged.
GC-MS analysis of aroma compounds
The GC-MS system consisted of an Agilent 5975 mass selective detector (MSD) coupled to an Agilent 7890 chromatograph fitted with a HP-5MS column (60 m length × 0.25 mm i.d. × 0.25 μm thickness; J&W Scientific). The oven and injector temperatures were same with those of GC-O analysis as described previously. Helium was used as carrier gas at the flow rate of 1 mL/min. The mass detector was operated in scan mode. The mass scan range was m/z 30 to 450. The mass spectrometer was operated in electron impact (EI) mode with an ionization voltage of 70 eV. The temperatures of quadrupole and ion source were 150°C and 230°C, respectively. The transfer line temperature was 280°C. The oven temperature was identical to the GC-O. Identification was carried out by comparison of mass spectra with a NIST11a.L and wiley7n.l library match (Agilent Technologies, Inc.), retention indices (on two columns of different polarities), and the availability of standard compounds. The retention indices were determined via sample injection with a homologous series of alkanes (C6–C30) (Sigma-Aldrich).
Calculation of odor activity values
The OAV of a compound was calculated by dividing the calculated concentrations with literature sensory thresholds in water, which was obtained from the literature.
Aroma recombination of Longjing tea
In order to compare the effectiveness of OAV and GC-O in characterizing the tea aroma, six aroma models were prepared by blending volatile compounds in their natural concentrations shown in . Those models consisted of odorants with AI > 4 and OAV > 10, respectively.
Table 1. Mean concentration (μg/kg) of volatile compounds detected in three Longjing tea samples.
Electronic nose analysis of recombination models
FOX 4000 nose from Alpha-MOS (Toulouse, France) coupled with 18 metal oxide sensors fitted with a headspace auto-sampler HS100 was used. All sensors were divided into three clusters (T, P, and LY) based on different oxides. The electronic nose was used to tentatively estimate the aroma profile similarity between two reconstituted models and the original odor concentrates of Longjing tea, considering that it was powerful for providing an odor print of the sample by mimicking the human olfactory perception. The procedures are as follows: 1 g of each aroma synthetic solution and the original concentrates were prepared in a 10 mL glass vial and capped with a Teflon/silicon rubber cap. Four copies of vials were orderly placed in the automatic sampler in the headspace system. Each vial was incubated at 80°C for 20 min under agitation (500 rpm). The injected volume was 250 µL. The parameters were optimized in detail and each analysis was repeated four times.
Sensory evaluation of recombination models
Aroma profile characterization for six reconstituted models and the original odor concentrates were performed by 10 experienced sensory panelists. Before the quantitative descriptive analysis, 10 g of original tea infusion was put in a 20 mL volume of white china cup covered with a plastic Petri dish and was served to a panelist in laboratory at room temperature (25°C). And panelists had discussed aroma compositions of samples through three preliminary sessions (each spent 3 h), until all of them had agreed to use them as the attributes. Then, a total of five aroma attributes including “green,” “floral,” “fruity,” “nutty,” and “smoky” were established. The score criterion of sensory evaluation was in accordance with the GC-O. These five aroma attributes were defined as following aromas: hexanal for “green” note, phenylethyl alcohol for “floral” note, maltol for “sweet” note, 2-ethyl-3,5-dimethylpyrazine for “nutty” note, and p-cresol for “smoky” note. After the training period, each of 10 g for the aroma profiles of reconstituted models and the original odor concentrates was introduced by the panelists. Each analysis was repeated three times.
Statistical analysis
The volatile compounds were submitted to variance analysis (ANOVA). Duncan’s multiple-range tests were applied to determine significant differences between the samples. All the analyses were carried out employing the XLSTAT, version 7.5 (Addinsoft, New York, NY, USA).
Results and discussion
Extraction of the volatile components by SPME
Because the extraction process was immediately conducted after the brewing, so the extraction temperature was set at 80°C, which was identical to the temperature of tea brewing. Thus, the conditions of fiber and extraction time were evaluated on the basis of the total of adsorbing capacity of aroma compounds in Longjing tea.
Five fibers, coated with 65 μm PDMS/DVB, 80 μm PA, 100 μm PDMS, 75 μm CAR/PDMS, and 50/30 μm CAR-PDMS-DVB, were assessed for the extraction of the aroma compounds. From , the total abundance of aroma compounds greatly varied with different fibers. Among those fibers, the 50/30 μm CAR-PDMS-DVB fiber extracted greatest aroma compounds, while the 100 μm PDMS presented least adsorbing capacity of aroma compounds. The reason might be that the distribution coefficient of two phase between coating and sample exist in the process of extraction. The adsorbing capacity depends on the characteristics of the coating material. Thus, the 50/30 μm CAR-PDMS-DVB fiber is selected in this experiment.
Figure 1. (a) Five fibers, coated with 65 μm PDMS/DVB, 80 μm PA, 100 μm PDMS, 75 μm CAR/PDMS, and 50/30 μm CAR-PDMS-DVB, were assessed for the extraction of the aroma compounds. (b) Six different extraction times were evaluated in the experiment.
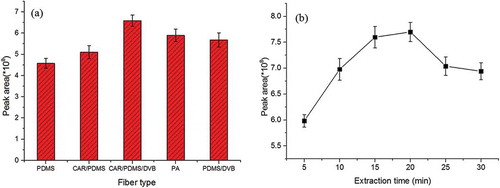
From , the total abundance of aroma compounds greatly varied with different extraction times. As the extension of time, the adsorbing capacity of aroma compounds increased gradually. The adsorption quantity reached maximum value when the extraction time was 20 min. After that, the adsorption quantity dropped. The reason might be that the aroma compounds gradually desorbed from the fiber with the extension of extraction time. Thus, the sample was extracted for 20 min.
Concentration and OAV of aroma compounds in Longjing tea
The total ion chromatograms (TIC) of aroma compounds of three Longjing tea samples are presented in . The numbers (1–53) in the peak represent the identified aroma compounds in . As shown in , the statistical analysis demonstrated that the most of aroma compounds identified in three Longjing tea samples present significant differences.
Figure 2. Total ion chromatogram (TIC) of three Longjing tea samples. The numbers (1–53) in the peak refer to the identified aroma compounds in .
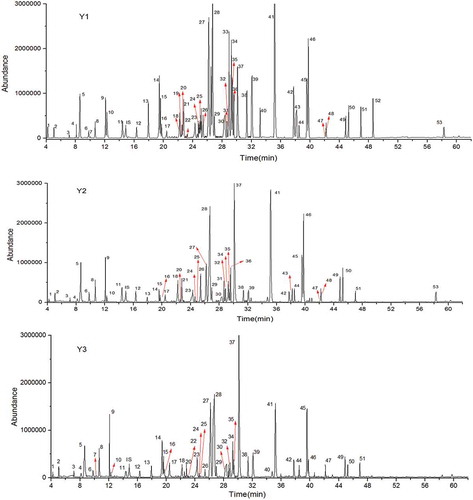
The aroma compounds of three green tea samples mainly consisted of 2-acetylpyrrole (15318.2–35838.9 µg/kg), geraniol (16896.7–35012.4 µg/kg), phenylethyl alcohol (30386.5–46075.0 µg/kg), benzyl alcohol (9070.6–26983.3 µg/kg), linalool (5300–22914.0 µg/kg), and 4-vinylphenol (2802.4–3673.7 µg/kg). Specifically, 2-acetylpyrrole possessed a “nutty, herbal” aroma as reported in other teas.[Citation15] Geraniol was found in green tea by other researchers and was a colorless, oily liquid with a “floral, rose-like” aroma.[Citation16] 4-Vinylphenol contributed to the “smoky” odor as well as other phenolics such as phenol, p-cresol, and 2-methoxy-4-vinylphenol.[Citation17] Phenylethyl alcohol had been reported to have a “floral, rose-like” odor and was detected in many green tea samples.[Citation18] Dimethyl sulfide, which had been described as “cabbage,” “garlic,” or “rotten egg-like” off-flavors at high concentration, also considered as a positive impression to tea at low concentration.[Citation19] Their abundant concentration in Longjing teas could be formed during spreading of fresh young shoots before processing, in which hydrolysis of their glyocosides and primeverosides by β-glucosidase primeverosidase occurs intensively.[Citation20]
Moreover, 30 compounds that had the high OAVs (>10) are displayed in . Specifically, geraniol (422.4–2508.3), epoxylinalool (601.5–1033.9), (Z)-nerolidol (235.5–559.3), 2-ethyl-3,5-dimethylpyrazine (328.0–420.7), β-ionone (227.7–387.3) presented highest OAVs (>100) in three green tea samples. Due to the unavailability of odor threshold data in the literature, the OAVs of 4-methyl-3-penten-2-one, (Z)-jasmone, (Z)-3-hexenyl benzoate, (Z)-3-hexenyl hexanoate, α-cadinol, and dihydroactinidiolide were not determined. Among those 30 compounds, geraniol showed the highest OAV, and it thus contributed significantly to the characteristic “floral” aroma in green tea. Epoxylinalool had rarely been reported in the literature and contributed to the “sweet” and “woody” odor.[Citation15] (Z)-nerolidol was responsible for rose and apple aromas with high OAV in samples. Therefore, (Z)-nerolidol might be a major contributor of the characteristic floral aroma note in the green tea investigated.
Table 2. GC-O and OAV of identified aroma-active compounds in Longjing tea samples.
In addition, two phenol compounds (4-vinylphenol, 2-methoxy-4-vinylphenol) were found in Longjin tea. Those compounds were always considered to impart smoky aroma by GC-O analysis. However, the OAVs of those two compounds varied greatly in green tea. The OAVs of 4-vinylphenol found in sample were high enough to be considered representative of an active compound, suggesting that smoky characteristics might contribute greatly to the aroma of green tea. On the contrary, the OAVs of 2-methoxy-4-vinylphenol were only 1.3 and 2.4 in the green tea. Thus, this compound might contribute little to green tea.
Pyrazine compounds were considered as another important compounds in tea, especially in green tea. In this study, a total of seven compounds (2-methylpyrazine, 2,5-dimethylpyrazine, 2,6-dimethylpyrazine, 2-ethylpyrazine, 2-ethyl-5-methylpyrazine, 2,3,5-trimethylpyrazine, 2-ethyl-3,5-dimethylpyrazine) were identified. Among those compounds, 2,5-dimethylpyrazine (3385.9–8943.5 µg/kg), 2-ethyl-5-methylpyrazine (2329.2–4974.4 µg/kg), and 2-methylpyrazine (1296.1–6435.5 µg/kg) presented relatively high amounts in study. Moreover, pyrazine compounds with high OAVs were 2-ethyl-3,5-dimethylpyrazine (OAV = 328.0–420.7) and 2-ethyl-5-methylpyrazine (OAV = 23.3–49.7). Pyrazine compounds were typically associated with roast aromas, and might form in the Maillard reaction occurring during manufacturing processes. A previous study found that these compounds were derived from amino acids and sugars.[Citation21] β-Ionone, which might be responsible for floral and woody odor, exhibited a high amount (1594.1–2710.7 μg/kg) and OAV (227.7–387.3) in tea. The mechanism of forming β-ionone was the oxidation and degradation of beta-carotene, which was caused by the enzymatic oxidation of catechins.[Citation22] β-Ionone might contribute to the floral and woody sensory properties of Longjin tea.
GC-O results for Longjing tea
The GC-O was performed to categorize the compounds according to their odor potency. As shown in , the compounds having the highest AIs were dihydroactinidiolide (AI = 9.1–9.2) and (Z)-nerolidol (AI = 8.4–8.8). (Z)-nerolidol was a volatile compound that gives a “floral” aroma. It existed in teas and could be considered as one of the key odorants for the high-quality flavor of tea.[Citation18] Dihydroactinidiolide, which existed in teas, contributed to the “roasted” aroma.[Citation15]On the other hand, geraniol, 2-ethylpyrazine, linalool, epoxylinalool, methyl salicylate, and benzyl alcohol also presented high AIs in all of aroma-active compounds. 2-Ethylpyrazine and other pyrazines, exhibiting “peanut,” “sweet,” and “nutty” aromas, were generally formed through the Maillard reaction or microbial metabolism.[Citation21] In general, many compounds (such as epoxylinalool, (Z)-nerolidol, and benzyl alcohol) both had high OAVs (>100) and AIs (>8), which confirmed the positive relationship between AI and OAV.[Citation23] Otherwise, compounds (such as 2-ethyl-3,5-dimethylpyrazine, geraniol, methyl jasmonate) that had high AIs but a relatively low OAVs, could be related to the difference of thresholds in air and water. In other words, AI depended on odor threshold in air, whereas the calculated OAVs depended on odor threshold in water, which was a better indication of the perceived aroma, taking the matrix into account.[Citation24]
Aroma recombination of Longjing tea
In order to verify the contribution of aroma-active compounds to the aroma profile of three different Longjing tea samples, synthetic blends were prepared based on the aforementioned findings. Six models were prepared according to the results shown in . That was to say, models were consisted of odorants with AI > 4 and OAV > 10, respectively (). Radar plots, obtained by ENA, were particularly suitable for illustrating relationships and trends concisely.[Citation25]
Table 3. Models consisting aroma compounds with AI > 4 and OAV > 10.
As for aroma radar plot of two aroma models (), the value corresponding to sensors LY2/G, LY2/AA, LY2/GH, and LY2/gCTL were rated slightly higher than that in the original odor concentrates of the Longjing tea, whereas the value corresponding to sensors T30/1, P10/2, T70/2, PA/2, P30/1, P40/2, and P30/2 were just the opposite, and the value corresponding to other sensors were with no obvious difference. Overall for the examined attributes, the aromas of two models were found to be comparable to the original odor concentrates of Longjing tea, as model 2 (OAV-based aroma model) was more similar to the original odor concentrates of Longjing tea. Similar conclusions were obtained from other models for Y2 and Y3 samples. These results tentatively indicated that compared with GC-O, OAV was a more reliable method in evaluating the key aroma of Longjing tea.
Figure 3. Radar plot of electronic nose analysis data for the original odor concentrates of three Longjing tea samples and different aroma synthetic solutions based on data from gas chromatography–olfactometry (GC-O) and odor activity value (OAV) as well as the value corresponding to sensors between each aroma recombination model and the original model.
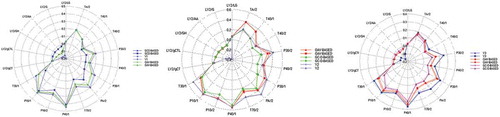
Moreover, the results of sensory test of differences between each model and the original Longjing tea were obtained to provide a graphic representation of the aroma profiles. And their difference and similarities are easily identified in . As shown in , for model 1 (GC-O-based model), “green” attribute was rated significantly lower than that in the original odor concentrates of Longjing tea, whereas “smoky” attribute was just the opposite; for model 2 (OAV-based model), “floral,” “sweet,” “smoky,” and “nutty” attributes were rated slightly higher than those in the original odor concentrates of Longjing tea, while “green” attribute was just the opposite. Similar results were also obtained from other models for Y2 and Y3 samples (). In other words, the OAV-based aroma model was more similar to the original Longjing tea aroma than that of GC-O-based model. The reasons might be that GC-O is affected by vigilance, adaptation, breathing, training, and individual sensitivity.[Citation26] However, it was necessary to investigate more samples of Longjing tea to confirm this hypothesis, since there were many grades and producing areas of Longjing tea ().
Conclusion
The aroma-active compounds in three Longjing tea samples were identified by GC-MS, GC-O, and OAV. The results showed that dihydroactinidiolide (AI = 9.1–9.2), (Z)-nerolidol (AI = 8.4–8.8), benzyl alcohol (AI = 8.1–8.4), and epoxylinalool (AI = 8.1–8.5) presented the highest AI in three green tea samples. While geraniol (422.4–2508.3), epoxylinalool (601.5–1033.9), (Z)-nerolidol (235.5–559.3), 2-ethyl-3,5-dimethylpyrazine (328.0–420.6), β-ionone (227.7–387.3) presented higher OAVs than other compounds. Moreover, the ENA and sensory evaluation were employed to verify and compare the effectiveness of identifying the profile characterization of three Longjing tea samples from GC-O-based and OAV-based aroma models. The results demonstrated that OAV-based aroma models were more similar to the original Longjing tea aroma than GC-O-based models.
Funding
This research was funded by Development of Electronic Cigarette with Chinese-style Cigarettes Flavor (number 2015CP06), Natural Science Foundation of Shanghai Institute of Technology (number YYY-11067), National Key Research and Development Program Nanotechnology Specific Project (number 2016YFA0200300), National Natural Science Foundation of China (number 21476140), and Shanghai Engineering Technology Research Center of Fragrance and Flavor (number 15DZ2280100).
Additional information
Funding
References
- Wang, Y.; Chen, J.; Zhang, D.; Zhang, Y.; Wen, Y.; Li, L.; Zheng, L. Tumoricidal Effects of a Selenium (Se)-Polysaccharide from Ziyang Green Tea on Human Osteosarcoma U-2 OS Cells. Carbohydrate Polymers 2013, 98, 1186−1190.
- Unachukwu, U.J.; Ahmed, S.; Kavalier, A.; Lyles, J.T.; Kennelly, E.J. White and Green Teas (Camellia sinensis var. sinensis): Variation in Phenolic, Methylxanthine, and Antioxidant Profiles. Journal of Food Science 2010, 75, 541−548.
- Wang, L.; Wei, K.; Cheng, H.; He, W.; Li, X.; Gong, W. Geographical Tracing of Xihu Longjing Tea using High Performance Liquid Chromatography. Food Chemistry 2014, 146, 98−103.
- Yu, H.; Wang, J. Discrimination of LongJing Green-tea Grade by Electronic Nose. Sensors and Actuators B-Chemical 2007, 122, 134−140.
- Dai, Y.; Xu, H. Analysis on Aromatic Components of Longjing Tea using SPME-GC/MS Methods. Journal of Tea (China) 2008, 34, 85−88.
- Lee, J.; Chambers, D.H.; Chambers, E.; Adhikari, K.; Yoon, Y. Volatile Aroma Compounds in Various Brewed Green Teas. Molecules 2013, 18, 10024−10041.
- Zhu, J.C.; Chen, F.; Wang, L.Y.; Niu, Y.W.; Chen, H.X.; Wang, H.L.; Xiao, Z.B. Characterization of the Key Aroma Volatile Compounds in Cranberry (vaccinium macrocarpon ait.) using Gas Chromatography-olfactometry (GC-O) and Odor Activity Value (OAV). Journal of Agricultural and Food Chemistry 2016, 64, 4990−4999.
- Zhu, J.C.; Chen, F.; Wang, L.Y.; Niu, Y.W.; Yu, D.; Shu, C.; Chen, H.X.; Wang, H.L.; Xiao, Z.B. Comparison of Aroma-active Volatiles in Oolong Tea Infusions using GC-olfactometry, GC-FPD, and GC-MS. Journal of Agricultural and Food Chemistry 2015, 63, 7499−7510.
- Pang, X.; Chen, D.; Hu, X.; Zhang, Y.; Wu, J. Verification of Aroma Profiles of Jiashi Muskmelon Juice Characterized by Odor Activity Value and Gas Chromatography-olfactometry-detection Frequency Analysis: Aroma Reconstitution Experiments and Omission Tests. Journal of Agricultural and Food Chemistry 2010, 60, 10426−10432.
- Buttara, M.; Intarapichet, K.O.; Cadwallader K.R. Characterization of Potent Odorants in Thai Chempedak Fruit (Artocarpus integer Merr.), an Exotic Fruit of Southeast Asia. Food Research International 2014, 66, 388−395.
- Mitropoulou, A.; Hatzidimitriou, E.; Paraskevopoulou A. Aroma Release of a Model Wine Solution as Influenced by the Presence of Non-volatile Components. Effect of Commercial Tannin Extracts, Polysaccharides and Artificial Saliva. Food Research International 2011, 44, 1561–1570.
- Saenz-Navajas, M.P.; Campo, E.; Cullere, L.; Fernandez-Zurbano, P.; Valentin, D.; Ferreira, V. Effects of the Nonvolatile Matrix on the Aroma Perception of Wine. Journal of Agricultural and Food Chemistry 2010, 58, 5574–5585.
- Chen, X.Q.; Ye, Y.; Chen, H.; Yi, J.F.; Feng, C.H.; Sun, C. Analysis on the Main Biochemical Components in Xihu Longjing Tea Poduced from Various Species of Tea Plant in Xihu Area of Origin. Science Technology of Food Industry (China) 2008, 02, 280−281.
- Yin, J.F.; Xu, Y.Q.; Yuan, H.B.; Yu, S.P.; Wei, K.K.; Chen, J.X.; Wang, F.; Wu, R.M. Dynamic Change of Main Biochemical Components of Premium Green Tea Fresh Leaves during Spreading. Journal of Tea Science(China) 2009, 29, 102−110.
- Joshi, R.; Gulati, A. Fractionation and Identification of Minor and Aroma-active Constituents in Kangra Orthodox Black Tea. Food Chemistry 2015, 167, 290−298.
- Zhu, M.; Li, E.; He, H. Determination of Volatile Chemical Constitutes in Tea by Simultaneous Distillation Extraction, Vacuum Hydrodistillation and Thermal Desorption. Chromatographia 2008, 68, 603−610.
- Chen, S.; Xu, Y.; Qian, M.C. Aroma Characterization of Chinese Rice Wine by Gas Chromatography-olfactometry, Chemical Quantitative Analysis, and Aroma Reconstitution. Journal of Agricultural and Food Chemistry 2013, 61, 11295−11302.
- Qin, Z.; Pang, X.; Chen, D.; Cheng, H.; Hu, X.; Wu, J. Evaluation of Chinese tea by the Electronic Nose and Gas Chromatography–Mass Spectrometry: Correlation with Sensory Properties and Classification According to Grade Level. Food Research International 2013, 53, 864−874.
- Isogai, A.; Utsunomiya, H.; Kanda, R.; Iwata, H. Changes in the Aroma Compounds of Sake during Aging. Journal of Agricultural and Food Chemistry 2005, 53, 4118−4123.
- Guo, W.; Yamauchi, K.; Watanabe, N.; Usui, T.; Luo, S.; Sakata K. A primeverosidase as a Main Glycosidase Concerned with the Alcoholic Aroma Formation in Tea Leaves. Bioscience Biotechnology and Biochemistry 1995, 59, 962−964.
- Amrani-Hemaimi, M.; Cerny, C.; Fay, L.B. Mechanisms of Formation of Alkylpyrazines in the Maillard Reaction. Journal of Agricultural and Food Chemistry 1995, 43, 2818−2822.
- Tan, Y.P.; Huang, J.A.; Liu, Z.H. Advances in Green Tea Aroma Components and their Variation during Tea Processing. Tea Communication 2006, 33, 35−38.
- Grosch, W. Detection of Potent Odorants in Foods by Aroma Extract Dilution Analysis. Trends Food Science and Technology 1993, 4, 68−73.
- Hausch, B.J.; Lorjaroenphon, Y.; Cadwallader K.R. Flavor Chemistry of Lemon-lime Carbonated Beverages. Journal of Agricultural and Food Chemistry 2015, 63, 112−119.
- Haddi, Z.; Amari, A.; Alami, H.; El Bari, N.; Llobet, E.; Bouchikhi, B. A Portable Electronic Nose System for the Identification of Cannabis-based Drugs. Sensors and Actuators B-Chemical 2011, 155, 456−463.
- Hanaoka, K.; Vallet, N.; Giampaoli, P.; Heyd, B.; MacLeod, P. Possible Influence of Breathing on Detection Frequency and Intensity Rating in Gas Chromatography-olfactometry. Food Chemistry 2001, 72, 97−103.
- Van Gemert, L.J. Complilations of Odour Threshold Values in Air, Water and Other Media; Van Setten Kwadraat: Houten, The Netherlands, 2003.
- Zhu, J.C.; Chen, F.; Wang, L.Y.; Niu, Y.W.; Shu, C.; Chen, H.X.; Xiao, Z.B. Comparison of Aroma-active Compounds and Sensory Characteristics of Durian (Durio zibethinus L.) Wines using Strains of Saccharomyces Cerevisiae with Odor Activity Values and Partial Least-squares Regression. Journal of Agricultural and Food Chemistry 2015, 63, 1939−1947.