ABSTRACT
Polyphenol oxidase (PPO) enzyme was extracted from Prunus spinosa L. subsp. dasyphylla plum, partially purified by acetone precipitation, and its biochemical properties were investigated. Different substrates (p-coumaric acid, L-tyrosine, p-hydroxyphenyl propionic acid (PHPPA), catechol, 4-methylcatechol (4-MTC), hydrocaffeic acid, gallic acid, quercetin, catechin, and epicatechin) were analysed to determine their affinities with Prunus spinosa PPO (PsPPO). The substrate specificity was in the following order: 4-MTC > catechol > hydrocaffeic acid > catechin > epicatechin. 4-MTC was the most suitable substrate (Km = 0.97 mM and Vmax = 4753 U/mg protein). The optimal pH values were 7.0 for 4-MTC and catechol, 5.0 for catechin and epicatechin, and 4.0–6.0 for hydrocaffeic acid. Optimal temperatures were 40°C for 4-MTC, 30°C for catechol, and 60°C for catechin, epicatechin, and hydrocaffeic acid. In the inhibition tests, the most potent inhibitor was found to be sodium metabisulphite (IC50 = 0.01 mM), followed by ascorbic acid, thiourea, benzoic acid, L-cysteine, and sodium azide. Approximately 80 and 75% of the diphenolase activity was conserved at pH 5.0 and 7.0, respectively, at 4°C after 7 d incubation. Moreover, native polyacrylamide gel electrophoresis (Native-PAGE) of the enriched extract revealed the presence of at least six bands with PPO activity, suggesting the existence of different PPO isoforms. However, the oxidation of diphenol related to browning significantly, so the data obtained in this research provided a basis for the prevention of enzymatic browning of Prunus spinosa during processing. High-performance liquid chromatography (HPLC) revealed the plum extract contained protocatechuic acid, p-OH benzoic acid, vanillic acid, syringic acid, epicatechin, p-coumaric acid, and luteolin.
Introduction
Polyphenol oxidase (PPO) is a common copper-containing enzyme with a dinuclear copper centre that catalyses two distinct reactions involving molecular oxygen, namely the o-hydroxylation of monophenols to o-diphenols (cresolase activity; E.C. 1.14.18.1) and the oxidation of o-diphenols to o-quinones (catecholase activity; E.C. 1.10.3.2).[Citation1,Citation2] The resulting o-quinones easily polymerise or react with a variety of cellular constituents, such as amino acids and proteins, to form complex brown, red, or black pigments. These reactions also produce undesirable browning in products during food processing, or in post-harvest of plant products, resulting in a decrease in their sensory properties and nutritional and economic value.[Citation1,Citation2,Citation3] Many researchers have characterized or purified PPO from various fruits and vegetables, such as Stanley plum,[Citation4] medlar fruits,[Citation5] mulberry fruit,[Citation6] persimmon (Diospyros kaki L.),[Citation3,Citation7] mamey,[Citation8] mango,[Citation9] wolf’s apple,[Citation10] Yomra apple (Malus communis L.),[Citation11] Ataulfo mango,[Citation12] chestnut kernel,[Citation13] Hemşin apple,[Citation14] Pyrus elaeagnifolia,[Citation15] Turkish Alyanak apricot (Prunus armenica L.),[Citation16] cape gooseberry,[Citation17]and Diospyros lotus.[Citation18]
Prunus spinosa subsp. dasyphylla, commonly known as “guvem” in Turkish, is a wild plum species of the Rosaceae family that is grown in various regions in Turkey (particularly in central, north, west, and south Anatolia) and bears a red-black-bluish, soft and edible fruit on ripening. Ethnobotanical studies have reported that the fruit of P. spinosa subsp. dasyphylla has been used as an astringent, diuretic, purgative, and decoction in the treatment of cardiac diseases in the European part of Turkey.[Citation19,Citation20]
Plant polyphenols are a large and diverse group of secondary metabolites commonly found in our diet, particularly in fruits, cereals, vegetables, and beverages. Various classes of polyphenols exist based on their structural characteristics. One of the main classes are the phenolic compounds, such as phenolic acids, flavonoids, procyanidins, and anthocyanins. P. spinosa L. extracts are reported to contain a high antioxidant capacity and high phenolic content; however, only a limited number of the phenolic compounds have been identified.[Citation19] P. spinosa subsp. dasyphylla is widely consumed as fruit, jam, syrup, or marmalade, produced from the whole fruit after deseeding. Enzymatic browning catalyzed by PPO also occurs in P. spinosa L. Forestalling of enzymatic browning requires a detailed study of the PPO biochemical properties, which can help reveal suitable methods to control PPO activity and the adverse browning associated with its activity. The aims of this research were to (1) biochemically characterise the PPO of Prunus spinosa by determining several selected substrates’ specificity, (2) determine their enzyme kinetic parameters, (3) determine the effects of pH and temperature on the enzyme activity in order to find the optimal ranges of work, (4) determine the inhibitory effect of some common PPO inhibitors and metal ions on the enzyme activity, (5) determine the thermal and pH stability of the enzyme, and (6) determine the phenolic compounds in the Prunus spinosa plum.
Materials and methods
Material and chemicals
P. spinosa L. subsp. dasyphylla plum was collected directly from Gümüşhane province, Turkey, at an altitude of 1300 m, in 2015 November and was stored at −30°C until used. All chemicals were of reagent grade and purchased from Sigma Chemical Co. (St. Louis, MO, USA).
Preparation of crude enzyme extract and acetone precipitation
The crude enzyme extract was prepared as reported in the literature.[Citation5,Citation15,Citation18,Citation21] P. spinosa plums (30 g) were placed in a Dewar flask under liquid nitrogen for 15 min to disrupt the cell membranes, and then homogenized in a blender in 100 mL of acetate buffer (pH 7.0, 50 mM) containing 2 mM ethylenediaminetetraacetic acid (EDTA), 1 mM MgCl2, 1 mM phenylmethylsulphonyl fluoride (PMSF), and 6% w/v Triton X-114 for 20 min. After that, the homogenate was filtered using three layers of cheesecloth and the filtrate was centrifuged at 15,000 rpm, 4°C for 30 min. An equal volume of cold acetone (−30°C) was added to the supernatant and the mixture was incubated overnight at 4°C for protein precipitation. After centrifugation at 10,000 rpm, 4°C for 20 min to remove the acetone, the precipitate was redissolved in an appropriate volume of 50 mM phosphate buffer (pH 7.0). This solution was used as the crude enzyme extract and characterised for its continued PPO activity during storage at 4°C for 6 weeks.
PPO activity assay
Phenolic compounds are the primary substrates of PPO. PPO activity was determined using p-coumaric acid, L-tyrosine, and p-hydroxyphenyl propionic acid (PHPPA) as the monophenolic substrates; catechol, 4-MTC, and hydrocaffeic acid as the diphenolic substrates; gallic acid as a triphenolic substrate; and quercetin, catechin, and epicatechin as pentaphenolic substrates. The enzyme activity was assayed using a 1 mL sample cuvette containing 100 µL substrate (stock 100 mM), an equal volume of 10 mM 3-methyl-2-benzothiazolinone hydrazone (MBTH) and 20 µL dimethylformamide (DMF), and diluted to 980 µL with 50 mM phosphate buffer (pH 7.0). Subsequently, 20 µL of crude enzyme extract was added. The increase in absorbance was measured at 494 nm for 4-MTC and at 500 nm for the remaining substrates, in a UV/visible spectrophotometer (1601UV, Shimadzu, Australia).[Citation22] The reference cuvette included all the reactants, except for the enzyme. One unit of PPO activity was defined as the amount of enzyme causing a 0.1 increase in absorbance per minute, in 1 mL of the reaction mixture.[Citation10]
Protein determination
The soluble protein content was determined according to the Lowry method using bovine serum albumin as the standard.[Citation23]
Native polyacrylamide gel electrophoresis
Non-denaturing polyacrylamide gel electrophoresis (PAGE) was performed according to Laemmli,[Citation24] by using 10% separating gel. After running the gel, it was incubated in 24 mM L-3,4-dihydroxyphenylalanine (L-DOPA) in 0.1 M phosphate buffer (pH 7.0) at 40°C for 1 h. Then, an appropriate amount of 1 mM ascorbic acid solution was added to the gel to detect the isoenzyme bands.
Substrate specificity and enzyme kinetics
The stock solutions (100 mM) of catechol, 4-MTC, catechin, epicatechin, hydrocaffeic acid, p-coumaric acid, L-tyrosine, PHPPA, gallic acid, and quercetin were used to monitor P. spinosa PPO (PsPPO) activity. The rate of the reaction was based on the increase in absorbance at the absorption maxima of the corresponding quinone products for each substrate.
The kinetics of P. spinosa diphenolase activity for 4-MTC (0.05–0.80 mM), catechol (0.10–1.50 mM), catechin (1.25–15.00 mM), epicatechin (0.31–10.00 mM), and hydrocaffeic acid (0.15–5.00 mM) substrates were analysed as 1/specific activity (1/V) versus 1/substrate concentration (1/[S]). The Michaelis–Menten constants (Km) and maximum velocity (Vmax) were obtained from the Lineweaver–Burk plots.[Citation25]
pH optimum and stability
The activity of PsPPO was determined as a function of pH, using 4-MTC, catechol, hydrocaffeic acid, catechin, and epicatechin as substrates (100 mM stock solutions). HAc-NaAc (pH 4.0 and 5.0), phosphate (pH 6.0 and 7.0), Tris–HCl (pH 8.0 and 9.0), and glycine-NaOH (pH 10.0) buffers were used.
For determining the PsPPO pH stability, 0.010 mL of crude enzyme solution was added to 0.990 mL buffer solution (pH 4–7, which covered the optimum pH values for 4-MTC, catechol, hydrocaffeic acid, catechin, and epicatechin substrates) and stored at 4°C for 24 d. At the end of each storage period, the diphenolase activity was assayed according to the percentage of residual PPO activity at the optimum pH by mixing 0.1 mL of 100 mM 4-MTC as substrate, 0.1 mL of 10 mM MBTH, and 0.02 mL dimethylformamide with the incubated crude enzyme solution.[Citation5,Citation21,Citation26]
Thermal activity and stability
The optimum temperature for diphenolase activity was measured at 10°C increments from 10 to 80°C by using 4-MTC, catechol, hydrocaffeic acid, catechin, and epicatechin as substrates. To determine the thermal stability of PsPPO, Eppendorf tubes containing the enzyme solution in 50 mM phosphate buffer (pH 7.0) were incubated in a thermoblock at 30, 40m and 60°C for 60 min, rapidly cooled in an ice bath for 5 min, and then brought to 25°C. The enzyme activity was subsequently assayed under the standard assay conditions in the presence of 4-MTC as the substrate. The percentage of residual PsPPO activity was calculated by comparison with the unincubated enzyme.[Citation15,Citation18]
Effect of inhibitors and metal ions
The inhibitory effects of ascorbic acid (12.50–200 µM), sodium azide (6.25–200 mM), benzoic acid (1.56–50 mM), sodium metabisulphite (3.13–50 µM), thiourea (0.78–25 mM), and cysteine (6.25–200 mM) on diphenolase activity were screened in the presence of 4-MTC as the substrate. The concentration of inhibitor causing 50% inhibition of PPO activity (IC50) was determined from a plot of the percentage of the remaining activity against the inhibitor concentration.
PsPPO activity was measured in the presence (1, 5 and 10 mM final concentrations) of various ionic compounds (Na+, K+, Ba2+, Cd2+, Mg2+, Mn2+, Hg2+, Ni2+, Zn2+, Ca2+, Co2+, and Al3+) under standard assay conditions, with 4-MTC as the substrate. The percentage of the remaining activity was expressed by comparison against a standard assay mixture with no metal ion added using the following equation: inhibition (%) = ((A0−Ai)/A0) × 100, where A0 is the initial PsPPO activity (no metal ion) and Ai is PsPPO activity with the metal ion.[Citation15,Citation21]
Preparation of the P. spinosa L. extract
P. spinosa L. extraction was performed by placing 2 g of the sample in a Falcon tube with 25 mL methanol (99%), which was then stirred using a shaker (Heidolph Promax 2020, Schwabach, Germany) at room temperature for 24 h, and then sonicated for 3 h with a sonicator (Elma® Transsonic Digital, Germany). The suspensions were filtered through filter paper (Whatman) and concentrated in a rotary evaporator (IKA-Werke, Staufen-Germany) under reduced pressure at 40°C. The residue was then resuspended in a minimal volume of methanol and stored at 4°C until use.
High-performance Liquid Chromatography Analysis of the Phenolic Compounds
Approximately 2.5 g of the sample was placed into a falcon tube (100 mL), to which 50 mL 99% methanol was then added. The mixture was continuously stirred with a shaker (HeidolphPromax 2020, Schwabach, Germany) at room temperature for 24 h, and then sonicated for 4 h with an ultrasonicator (ultrasonic Elma Scmidbauer GmbH) Germany. Particles were removed using Whatman filter paper and concentrated in a rotary evaporator (IKA-Werke, Staufen, Germany) at 40°C. The residue was redissolved in methanol to a known final concentration and kept at 4°C until use for phenolic compound analysis.
Samples were prepared for high-performance liquid chromatography (HPLC) analysis by dissolving the residue in 10 mL acidified distilled water (pH 2). The sample was then extracted three times with 15 mL of diethyl ether:ethyl acetate mixtures (1:1 v/v). The organic solvents were subsequently removed using a rotary evaporator. The final residue was redissolved in methanol and completed to a final volume of 2 mL with methanol, before HPLC analysis.
The methanolic extract was evaporated until dryness with a rotary evaporator at 40°C. The residue was dissolved in 15 mL acidified distilled water (pH 2). Liquid–liquid extraction was carried out with 5 × 3 mL diethyl ether and 5 × 3 mL ethyl acetate, consecutively. Fourteen phenolic standards were analysed using an HPLC system (Elite LaChrom Hitachi, Japan) equipped with a reverse-phase C18 column (150 × 4.6 mm, 5 μm; Fortis) maintained at 30°C. Acetonitrile, water, and acetic acid were used for the mobile phase by applying the programmed gradient. The mobile phase consisted of (A) 2% acetic acid in water and (B) acetonitrile:water (70:30). The sample injection volume was 25 μL and the flow rate was 0.75 mL/min. The programmed solvent used began with a linear gradient held at 95% A for 3 min, decreasing to 80% A at 10 min, 60% A at 20 min, 20% A at 30 min, and finally 95% A at 50 min.[Citation27]
HPLC analysis of the phenolic compounds method validation
The method described in this study was validated according to the method described by Ribani.[Citation28]The validation characteristics evaluated were selectivity, accuracy, precision, linearity, robustness, and limits of detection and quantification. The validation characteristics evaluated were selectivity, accuracy, precision, linearity, robustness, and limits of detection and quantification. Standard solutions of the phenolics were prepared for calibration of the device. The standard solutions were filtered at 0.45 μm and collected in vials. These standard solutions were read in the UV on an HPLC device, and calibration graphs were generated according to their arrival times. Linearity was determined by calculation of the regression plots using the least squares method and was expressed as the determination coefficient (R2). Concentrations of all compounds in the bee product samples were calculated based on peak area ratios.
Calibration generated at the linear measurement range was calculated from the equation data from the graph and using the formulas given below for limit of detection (LOD) and limit of quantification (LOQ). LOD was calculated using the following formula:
where SD is the standard deviation of the response and m is the slope of the calibration curve.[Citation28] LOQ was established using the following formula:
LOD and LOQ were experimentally verified by injection of phenolic compounds at the LOD and LOQ concentrations as follows:
where SD is the standard deviation at the lowest level of the calibration curve, and m is slope of the calibration curve. Precision was estimated by evaluating intra-batch precision (repeatability) and inter-batch precision (preparation process, repeatability). The precision of the method (within-day variations of replicate determinations) was checked by injecting reference compounds six times at the LOD and LOQ levels. The area values were recorded, and the dimethylformamide (DMF) was calculated ().
Table 1. RP-HPLC-UV validation parameters of the phenolic compounds in P. spinosa L.
Results and discussion
The results obtained in the present study revealed that PsPPO displaying diphenolase activity with broad substrate specificity, and good pH and thermal stabilities, was successfully biochemically characterized.
Native PAGE
Under native PAGE, six isoenzyme bands were detected by in-gel enzymatic activity staining with L-DOPA (). Various numbers of PPO isoenzyme bands obtained from diverse sources are reported in the literature. For instance, two isoenzymes have been detected in wild pear (P. elaeagnifolia),[Citation15] and an additional third and fourth PPO isoenzyme have been reported in Turkish Alyanak apricot (P. armenica L.)[Citation16] and persimmon (D. kaki L.),[Citation7] respectively.
Substrate specificity and enzyme kinetics
The substrate specificity of PsPPO at pH 7.0, room temperature, was investigated spectrophotometrically, using 10 commercial-grade substrates, namely catechol, 4-MTC, catechin, epicatechin, hydrocaffeic acid, p-coumaric acid, L-tyrosine, PHPPA, gallic acid, and quercetin. The calculated PPO activity using 4-MTC, catechol, hydrocaffeic acid, catechin, and epicatechin substrates at 100 mM decreased in the following order: 3795 > 2240 > 1840 > 1133 > 908 U/mg protein (). Therefore, it can be summarized that PsPPOs have substrate binding sites with high affinity for hydrocaffeic acid and catechol, while 4-MTC is the best substrate for PsPPOs. The PsPPO showed no or little activity towards p-coumaric acid, PHPPA, and L-tyrosine. These results suggested that PPOs in P. spinosa plums lack monophenolase (cresolase) activity. Catechol and 4-MTC are common substrates for determining the activity of PPO isolated from different food and plant sources.[Citation7,Citation10,Citation13–Citation16,Citation18,Citation29]
Table 2. Substrate specificity and activity (U/mg protein) of P. spinosa polyphenol oxidase.
The Michaelis–Menten constant (Km) and maximum reaction velocity (Vmax) values for the diphenolase activity of PsPPO were determined using five substrates (4-MTC, catechol, hydrocaffeic acid, catechin, and epicatechin) at seven different concentrations and under optimum analysis conditions. The Lineweaver–Burk double reciprocal plots of 1/V and 1/[S] concentration (, –) confirmed that the maximum catalytic efficiency and substrate affinity were achieved in the presence of 4-MTC, followed by catechol, hydrocaffeic acid, catechin, and then epicatechin (). Using 4-MTC, catechol, hydrocaffeic acid, catechin, and epicatechin as substrates, the Km and Vmax values were calculated as 0.97, 2.35, 2.49, 4.26, and 6.04 mM; 4753, 3964, 7628, 1686, and 2093 U/mg protein, respectively, under optimum reaction conditions (, –). The obtained Km values for 4-MTC and epicatechin were 0.97 and 6.04, respectively (an increase of 6.2-fold). The isoenzymes exhibited different kinetic properties. The Km value can change depending on the structure of the enzyme, the number of enzyme isoforms, pH, temperature, and ionic strength.[Citation13,Citation14,Citation16,Citation29]
Table 3. Some biochemical parameters of P. spinosa polyphenol oxidase towards various substrates.
Figure 2. The Michaelis–Menten equation of diphenolase activity of P. spinosa for various substrates. (A) 4-methylcatechol (0.05–0.80 mM), (B) catechol (0.10–1.50 mM), (C) hydrocaffeic acid (0.15–5.00 mM), (D) catechin (1.25–15.00 mM), and (E) epicatechin (0.31–10.00 mM) substrates were analysed as 1/specific activity (1/V) versus 1/substrate concentration (1/[S]). The Michaelis–Menten constants (Km) and maximum velocity (Vmax) were obtained from the Lineweaver–Burk plots.
![Figure 2. The Michaelis–Menten equation of diphenolase activity of P. spinosa for various substrates. (A) 4-methylcatechol (0.05–0.80 mM), (B) catechol (0.10–1.50 mM), (C) hydrocaffeic acid (0.15–5.00 mM), (D) catechin (1.25–15.00 mM), and (E) epicatechin (0.31–10.00 mM) substrates were analysed as 1/specific activity (1/V) versus 1/substrate concentration (1/[S]). The Michaelis–Menten constants (Km) and maximum velocity (Vmax) were obtained from the Lineweaver–Burk plots.](/cms/asset/348fd330-60d6-47f1-9017-ddc68d2386e4/ljfp_a_1343349_f0002_b.gif)
The respective Km and Vmax values obtained using 4-MTC as the substrate were previously reported as 3.57 mM and 4781 U/mg protein for P. elaeagnifolia,[Citation15] 3.88 mM and 38.51 UPPO/mL/min for Cape gooseberry,[Citation17] 3.8 mM and 1250 U/mg protein for D. lotus,[Citation18] 0.15 mM and 3.01 U/mL for Wolf’s apple,[Citation10] 3.69 mM and 0.24 IU/mL for Ataulfo mango,[Citation12] and 94 mM and 1.3 mmol/L for chestnut kernel.[Citation13] The corresponding values using catechol as the substrate were expressed as 6.47 mM and 3.42 U/mL for Wolf’s apple,[Citation10] 5.7 mM and 88 µM/min for medlar fruits,[Citation5] 3.40 mM and 333.3 EU/mL/min for Hemşin apple,[Citation14] 14.62 mM and 0.28 IU/mL for Ataulfo mango,[Citation12] and 12.4 mM and 55.2 µM/min for persimmon.[Citation3] These previous reports reveal the variability in the apparent Km and Vmax values of PPOs from various sources.
Optimum pH and stability
The optimum pH for the diphenolase activity of crude PsPPO towards 4-MTC, catechol, hydrocaffeic acid, catechin, and epicatechin was pH 7.0, 7.0, 4.0–6.0, 5.0, and 5.0, respectively (–, ). Similar values are reported in the literature, such as pH 7.0 for P. elaeagnifolia,[Citation15] chestnut kernel,[Citation13]and Yomra apple (M. communis L.),[Citation11] pH 6.5 for medlar fruits,[Citation5] pH 5.5 for Hemşin apple,[Citation14] and between pH 4.5–8.0 for mulberry fruit.[Citation6] It was reported that the differences in optimum pH for PPO activity depended on several factors, such as the plant source, extraction method, temperature, purity of the enzyme, the number of enzyme isoforms, buffer system used, and type of phenolic substrate.[Citation30] According to Kolcuoğlu,[Citation31] the pH can alter the ionisation of the substrate; hence, it is the determining factor in the expression of enzymatic activity.
Figure 3. pH-activity profile for P. spinosa polyphenol oxidase in the presence of various substrates; (A) 4-methylcatechol, (B) catechol, (C) hydrocaffeic acid, (D) catechin, and (E) epicatechin, and (F) pH stability of P. spinosa polyphenol oxidase at 4°C, at pH 4.0, 5.0, 6.0, and 7.0, incubated in the presence of 4-methylcatechol as a substrate for 24 days.
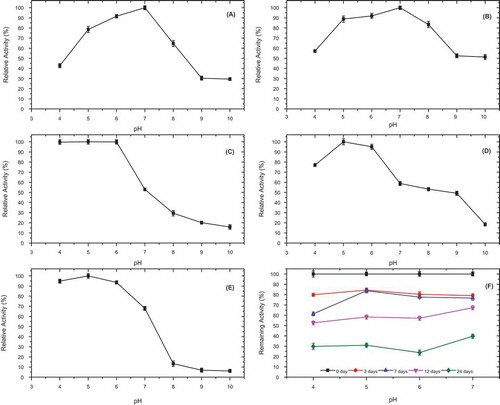
When the crude enzyme eluate was incubated at pH 4.0, 5.0, 6.0, and 7.0 at 4°C for up to 24 d (), 80, 84, 80, and 79% of the original diphenolase activity was, respectively, maintained at the end of 3 d. After 24 d, the enzyme preserved its activity by 40 and 31% at pH 5.0 and 7.0, respectively (). It was earlier reported that PPO from P. elaeagnifolia subsp. Pallas was extremely stable, retaining at least 95% of its original activity over pH in the range of 5.0–8.0 after incubation at 4°C for 72 h.[Citation15] In contrast, persimmon,[Citation3] D. kaki L.,[Citation7] D. lotus,[Citation18]and cherry laurel[Citation21] lost PPO activity, while P. elaeagnifolia,[Citation15] Yomra apple (M. communis L.),[Citation11] and Hemşin apple[Citation14] conserved their respective diphenolase activity at their optimum pH for 24 h incubation.
Optimum temperature and stability
The temperature dependence for the diphenolase activity of crude PsPPO (–) indicates the optimum temperature towards 4-MTC, catechol, hydrocaffeic acid, catechin, and epicatechin substrates occurred at 40, 30, 60, 60, and 60°C, respectively (–, ). It appears that in the presence of hydrocaffeic acid, catechin, and epicatechin, the diphenolase activity shows low sensitivity to temperatures above its optimum. Comparing other published results for PPO activity, the optimum temperature towards catechol substrate was 55°C for medlar fruits[Citation5] and persimmon (D. kaki L.),[Citation7] 40°C for Cape gooseberry[Citation17] and Yomra apple,[Citation11] and 30–40°C for Hemşin apple.[Citation14] In contrast to Navarro et al.,[Citation7] Özen et al.[Citation3] reported an optimum of 20°C for persimmon (D. kaki L.). In the presence of 4-MTC substrate, the optimal temperature for diphenolase activity reported by other researchers was between 25 and 40°C.[Citation3,Citation5,Citation11,Citation15,Citation18]
Figure 4. Optimum temperature of P. spinosa polyphenol oxidase in the presence of various substrates; (A) 4-methylcatechol, (B) catechol, (C) hydrocaffeic acid, (D) catechin, and (E) epicatechin, and (F) thermal stability of P. spinosa polyphenol oxidase at 30, 40, and 60°C, at pH 7.0, for 60 min.
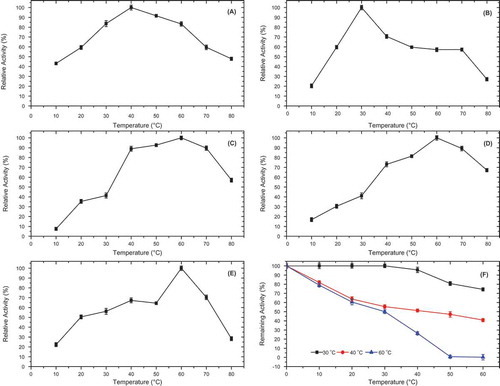
The heat stability profiles for the diphenolase activity of PsPPO () showed that 100, 82, and 79% of the original activity were maintained at 30, 40, and 60°C, at 10 min, respectively. At 1 h incubation, the enzyme conserved its diphenolase activity by 74, 41, and 0.24% at 30, 40, and 60°C, respectively (). In comparison, the diphenolase activity of PPO from persimmon was stable at 20–45°C for 30 min,[Citation3] while Stanley plum PPO was stable at 70°C for 30 min.[Citation4] The thermal stability of the PPO may be related to the ripeness of the plant from which it is sourced, while different molecular forms from the same source may also exhibit variations in their thermostability.[Citation32]
Effect of various inhibitors and metal ions on PPO activity
Polyphenoloxidase is the target for the development of several food anti-browning agents. The ability of ascorbic acid (12.50–200 µM), sodium azide (6.25–200 mM), benzoic acid (1.56–50 mM), sodium metabisulphite (3.13–50 µM), thiourea (0.78–25 mM), and L-cysteine (6.25–200 mM) to inhibit 4-MTC oxidation by PsPPO demonstrated that the variation observed in the IC50 values were associated with differences in the mechanism of inhibition concerning the compound used. Sodium metabisulphite had the greatest inhibitory effect on diphenolase activity, with an IC50 value of 0.01 ± 0.00 mM (, –). Sodium metabisulphite can act as a reducing agent for o-benzoquinones, while thiourea can react with quinones, interfering with PPO activity. Ascorbic acid acts more as an antioxidant than as an enzyme inhibitor because it reduces the initial o-quinone formed by the enzyme to the original diphenol before it undergoes a secondary reaction, which leads to browning.[Citation10] The IC50 values of the other inhibitors studied are given in . Can et al.[Citation11] reported IC50 values of 0.07, 0.05, 30.04, and 9.80 mM for sodium metabisulphite, ascorbic acid, sodium azide, and benzoic acid, respectively. Many studies have highlighted ascorbic acid, sodium metabisulphite, sodium diethyldithiocarbamate, L-cysteine, tropolone, and sodium ascorbate as the most effective PPO inhibitors.[Citation3,Citation5,Citation7,Citation10–Citation16,Citation18,Citation25,Citation27,Citation33,Citation34]
Table 4. The IC50 values of several inhibitors on the diphenolase activity of P. spinosa polyphenol oxidase using 4-MTC as substrate.
Figure 5. Inhibition effect of common polyphenol oxidase inhibitors on the diphenolase activity of P. spinosa. (A) Ascorbic acid (12.50–200 µM), (B) sodium azide (6.25–200 mM), (C) benzoic acid (1.56–50 mM), (D) sodium metabisulphite (3.13–50 µM), (E) thiourea (0.78–25 mM), and (F) L-cysteine (6.25–200 mM).
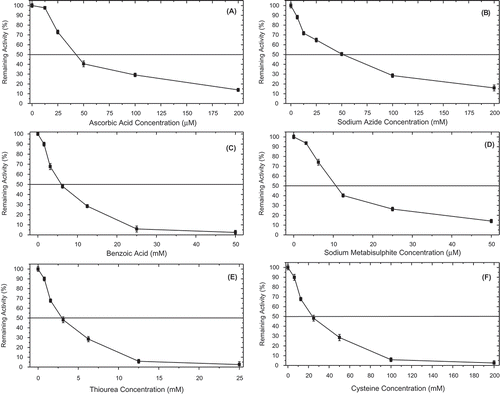
PPO activity was inhibited by K+, Mg2+, Ca2+, Ba2+, Mn2+, Cd2+, and Hg2+, while it was stimulated by Co2+, Ni2+, Zn2+, and Al3+, at 1 mM final concentration (). The diphenolase activity was inhibited by 10 mM final concentration of all the metal ions studied (). Previous studies showed that various metal ions either inhibited or stimulated PPO activity.[Citation3,Citation5,Citation10] According to the researchers, metal ions may have different coordination numbers and geometries in their coordination compounds, and potential as Lewis acids; thus, they may behave differently towards proteins as ligands. These differences may also result in metal binding to different sites, and therefore perturb the enzyme structure in different ways.[Citation35]
Table 5. Effect of various metal ions on P. spinosa polyphenol oxidase activity in the presence of 4-MTC as substrate.
Analysis of phenolic compounds by HPLC-UV
The Rosaceae family consists of approximately 175 species distributed worldwide,[Citation36,Citation37] such as P. armeniaca, P. mume, P. persica, P. salicina, P. domestica, P. genus, P. tomentosa, and P. cerasus. Recent reports confirm that these plants contain high levels of flavonoids. The chromatographic separation of the phenolic compounds in P. spinosa are presented in and their mean quantities (µg/g sample) in . Five phenolic acids were detected in the methanolic plum extract, namely protocatechuic acid (20.40 ± 6.00 µg/100g sample), p-OH benzoic acid (21.81 ± 9.01 µg/100g sample), vanillic acid (155.92 ± 12.60 µg/100g sample), syringic acid (20.70 ± 5.22 µg/100g sample), p-coumaric acid (58.60 ± 6.50 µg/100g sample), as well as flavonoids, as epicatechin (59.80 ± 10.10 µg/100g sample), and flavones, as luteolin (93.91 ± 12.40 µg/100g sample). In an earlier study, P. spinosa fruits presented 29.78 mg phenolic acids/100 g sample.[Citation38] Kaempferol 3-O-arabinofuranoside (juglanin) and quercetin 3-O-arabinofuranoside (avicularin) were isolated from extracts of P. spinosa flowers.[Citation39] P. spinosa L. (blackthorn) has also been found to contain substantial quantities of phenolic antioxidants,[Citation40,Citation41] in particular, flavonol heterosides (quercetin and kaempferol) and phenolic acids (neochlorogenic and caffeic derivatives).
Table 6. Phenolic acid content of Prunus spinosa L. plum.
Conclusion
The characterization of biochemical properties of PPO is important because of the browning reactions, which occur in fruits and vegetables. For this reason, PPO enzyme from Prunus spinosa L. subsp. dasyphylla plum was extracted and has been characterised for the first time. The optimum pH varies from about 4.0 to 7.0, depending on the substrate. The optimum temperature for maximum enzyme activity was 60°C (for hydrocaffeic acid, catechin, and epicatechin), which is much higher than the values commonly reported for PPOs from other fruits. Based on the enzyme kinetic, which was performed by means of the model of Lineweaver–Burk, the kinetic parameters (Vmax, Km) were determined for the substrates. The enzyme seemed to have the highest affinity (lowest Km) for 4-MTC, catechol, hydrocaffeic acid, catechin, and epicatechin. L-tyrosine was also tested, but was little oxidised by PPO. The partially purified PPO enzyme appears to have biochemical characteristics similar to several plant PPOs in terms of substrate specificity, pH, and temperature of assay and kinetic parameters. Among the various PPO inhibitors tested, sodium metabisulphite was the most effective inhibitor of the enzyme with an IC50 0.01 ± 0.00 mM. Due to its nontoxic properties, ascorbic acid with an IC50 0.04 ± 0.00 mM may be promising alternatives to sulphite in preventing the browning of plant foods. In addition, six activity bands were detected in the “Prunus spinosa L. subsp. dasyphylla” extract assayed by native gel electrophoresis, suggesting for the first time the presence of at least six PPO izoenzymes in plum fruits. Besides these results, it was revealed the plum extract contained protocatechuic acid, p-OH benzoic acid, vanillic acid, syringic acid, epicatechin, p-coumaric acid, and luteolin.
Nomenclature
DHPPA | = | 3-(3,4-dihydroxyphenyl) propionic acid |
L-DOPA | = | L-3,4-dihydroxyphenylalanine |
4-MTC | = | 4-methylcatechol |
PPO | = | polyphenol oxidase |
PsPPO | = | Prunus spinosa polyphenol oxidase |
References
- Guo, L.; Ma, Y.; Shi, J.; Xue, S. The Purification and Characterisation of Polyphenol Oxidase from Green Bean (Phaseolus vulgaris L.). Food Chemistry 2009, 117, 143–151.
- Rivas, N.J.; Whitaker, J.R. Purification and Some Properties of Two Polyphenol Oxidases from Bartlett Pears. Plant Physiology 1973, 52, 501–507.
- Özen, A.; Colak, A.; Dincer, B.; Güner, S. A diphenolase from Persimmon Fruits (Diospyros kaki L., Ebenaceae). Food Chemistry 2004, 85, 431–437.
- Siddiq, M.; Sinha, N.K.; Cash, J.N. Characterization of Polyphenoloxidase from Stanley Plums. Journal of Food Science 1992, 57, 1177–1179.
- Dincer, B.; Colak, A.; Aydin, N.; Kadioglu, A.; Güner, S. Characterization of Polyphenol Oxidase from Medlar Fruits (Mespilus germanica L. Rosaceae). Food Chemistry 2002, 77, 1–7.
- Arslan, O.; Erzengin, M.; Sinan, S.; Ozensoy, O. Purification of Mulberry (Morus alba L.) Polyphenol Oxidase by Affinity Chromatography and Investigation of Its Kinetic and Electrophoretic Properties. Food Chemistry 2004, 88, 479–484.
- Navarro, J. L.; Tarrega, A.; Sentandreu, M.A.; Sentandreu, E. Partial Purification and Characterization of Polyphenol Oxidase from Persimmon. Food Chemistry 2014, 157, 283–289.
- Palma-Orozco, G.; Ortiz-Moreno, A.; Dorantes-Alvarez, L.; Sampedro, J. G.; Najera, H. Purification and Partial Biochemical Characterization of Polyphenol Oxidase from Mamey (Pouteria sapota). Phytochemistry 2011, 72, 82–88.
- Palma-Orozco, G.; Marrufa-Hernandez, N.A.; Sampedro, J.G.; Najera, H. Purification and Partial Biochemical Characterization of Polyphenol Oxidase from Mango (Mangifera indica cv. Manila). Journal of Agricultural and Food Chemistry 2014, 62, 9832–9840.
- Batista, K.A.; Batista, G.L.A.; Alves, G.L.; Fernandes, K.F. Extraction, Partial Purification and Characterization of Polyphenol Oxidase from Solanum lycocarpum Fruits. Journal of Molecular Catalysis B: Enzymatic 2014, 102, 211–217.
- Can, Z.; Dincer, B.; Sahin, H.; Baltas, N.; Yildiz, O.; Kolayli, S. Polyphenol Oxidase Activity and Antioxidant Properties of Yomra Apple (Malus communis L.) from Turkey. Journal of Enzyme Inhibition and Medicinal Chemistry 2014, 29, 829–835.
- Cheema, S.; Sommerhalter, M. Characterization of Polyphenol Oxidase Activity in Ataulfo Mango. Food Chemistry 2015, 171, 382–387.
- Gong, Z.; Li, D.; Liu, C.; Cheng, A.; Wang, W. Partial Purification and Characterization of Polyphenol Oxidase and Peroxidase from Chestnut Kernel. LWT-Food Science and Technology 2015, 60, 1095–1099.
- Aydin, B.; Gulcin, I.; Alwasel, S.H. Purification and Characterization of Polyphenol Oxidase from Hemşin Apple (Malus communis L.). International Journal of Food Properties 2015, 18, 2735–2745.
- Baltas, N. Investigation of a Wild Pear Species (Pyrus elaegnifolia subsp. elaegnifolia Pallas) from Antalya, Turkey: Polyphenol Oxidase Properties and Anti-xanthine Oxidase, Anti-urease and Antioxidant Activity International Journal of Food Properties. 2016, 20(3), 585–595.
- Ünal, M.U.; Şener, A. Two-year Comparison of the Biochemical Properties of Polyphenol Oxidase from Turkish Alyanak Apricot (Prunus armenica L.). Food Chemistry 2016, 190, 741–747.
- Bravo, K.; Osorio, E. Characterization of Polyphenol Oxidase from Cape Gooseberry (Physalis peruviana L.) Fruit. Food Chemistry 2016, 197, 185–190.
- Faiz, Ö.; Baltas, N. Polyphenol Oxidase Properties, Anti-urease, and Anti-Acetylcholinesterase Activity of Diospyros lotus L. (plum persimmon). International Journal of Food Properties 2016, 20, 1186–1196.
- Aliyazicioğlu, R.; Yildiz, O.; Sahin, H.; Eyuboglu, O.E.; Ozkan, M.T.; Karaoglu, S.A.; Kolayli, S. Phenolic Components and Antioxıdant Activity of Prunus spinosa from Gümüşhane, Turkey. Chemistry of Natural Compounds, 2015, 51, 346–349.
- Kültür, S. Medicinal Plants Used in Kirklareli Province (Turkey). Journal of Ethnopharmacology 2007, 111, 341–364.
- Colak, A.; Özen, A.; Dincer, B.; Güner, S.; Ayaz, A.F. Diphenolases from Two Cultivars of Cherry Laurel (Laurocerasus officinalis Roem.) Fruits at an Early Stage of Maturation. Food Chemistry 2005, 90, 801–807.
- Espin, J.C.; Trujano, M.F.; Tudela, J.; Garcia-Canovas, F. Monophenolase Activity of Polyphenol Oxidase from Haas Avocado. Journal of Agricultural and Food Chemistry 1997, 45, 1091–1096.
- Lowry, O.H.; Rosebrough, N.J.; Farr, A.L.; Randall, R.J. Protein Measurement with the Folin Phenol Reagent. The Journal of Biological Chemistry 1951, 193, 265–275.
- Laemmli, U.K. Cleavage of Structural Proteins during the Assembly of the Head of Bacteriophage T4. Nature 1970, 227, 680–685.
- Lineweaver, H.; Burk, D. The determination of Enzyme Dissociation Constants. Journal of the American Chemical Society 1934, 56, 658–666.
- Liu, F.; Zhao, J.H.; Wen, X.; Ni, Y.Y. Purification and Structural Analysis of Membrane-Bound Polyphenol Oxidase from Fuji Apple. Food Chemistry 2015, 183, 72–77.
- Can, Z.; Yildiz, O.; Sahin, H.; Tturumtay, E.A.; Silici, S.; Kolayli, S. An Investigation of Turkish Honeys: Their Physico-chemical Properties, Antioxidant Capacities and Phenolic Profiles. Food Chemistry 2015, 180, 133–141.
- Ribani, M.; Collins, C.H.; Bottoli, C.B. Validation of Chromatographic Methods: Evaluation of Detection and Quantification Limits in the Determination of Impurities in Omeprazole. Journal of Chromatography A. 2007, 1156(1–2), 201–205.
- Sakıroglu, H.; Yılmaz, E.; Erat, M.; Öztürk A.E. Selected Properties of Polyphenol Oxidase Obtained from Ispir Sugar Bean. International Journal of Food Properties 2013, 16, 1314–1321.
- Sellés-Marchart, S.; Casado-Vela, J.; Bru-Martinez, R. Isolation of a Latent Polyphenol Oxidase from Loquat Fruit (Eriobotrya japonica Lindl.): Kinetic Characterization and Comparison with the Active form. Archives of Biochemistry and Biophysics 2006, 446, 175–185.
- Kolcuoğlu, Y. Purification and Comparative Characterization of Monophenolase and Diphenolase Activities from a Wild Edible Mushroom (Macrolepiota gracilenta). Process Biochemistry 2012, 47, 2449–2454.
- Zhou, H.; Feng, X. Polyphenol Oxidase from Yali pear (Pyrus bretschneideri). Journal of the Science of Food and Agriculture 1991, 57, 307–313.
- Tuncay, D.; Yagar, H. Comparison of Polyphenol Oxidases Prepared From Different Parts of Artichoke (Cynara Scolymus L.). International Journal of Food Properties 2011, 14, 809–821.
- Aydemir, T. Selected Kinetic Properties of Polyphenol Oxidase Extracted from Rosmarinus Officinalis L. International Journal of Food Properties 2010, 13, 475–485.
- DiTusa, C.A.; Christensen, T.; McCall, K.A.; Fierke, C.A.; Toone, E.J. Thermodynamics of Metal Ion Binding. 1. Metal Ion Binding by Wild-type Carbonic Anhydrase. Biochemistry 2001, 40, 5338–5344.
- Jang, G.H.; Kim, H.W.; Lee, M.K.; Jeong, S.Y.; Bak, A.R.; Lee, D.J.; Kim, J.B. Characterization and Quantification of Flavonoid Glycosides in the Prunus genus by UPLC-DAD-QTOF/MS. Saudi Journal of Biological Sciences 2016, doi: 10.1016/j.sjbs.2016.08.001.
- Rashid, F.; Ahmed, R.; Mahmood, A.; Ahmad, Z.; Bibi, N.; Kazmi, S.U. Flavonoid Glycosides from Prunus armeniaca and the Antibacterial Activity of a Crude Extract. Archives of Pharmacal Research 2007, 30, 932–937.
- Guimarães, R.; Barros, L.; Dueñas, M.; Carvalho, A.M.; Queiroz, M.J.; Santos-Buelga, C.; Ferreira, I. Characterisation of Phenolic Compounds in Wild Fruits from Northeastern Portugal. Food Chemistry 2013, 14, 3721–3730.
- Olszewska, M.; Wolbis, M. Flavonoids from the Flowers of Prunus spinosa L. Acta Poloniae Pharmaceutica 2001, 58, 367–372.
- Ruíz-Rodríguez, B.M.; Ancos, B.; Sánchez-Moreno, C.; Fernández-Ruíz, V.; Sánchez-Mata, M.C.; Cámara, M.; Tardío, J. Wild blackthorn (Prunus spinosa L.) and Hawthorn (Crataegus monogyna Jacq.) Fruits as Valuable Sources of Antioxidants. Fruits 2014, 69, 61–73.
- Veličković, J.M.; Kostić, D.A.; Stojanović, G.S.; Mitić, S.S.; Mitić, M.N.; Ranđelović, S.S.; Ðorđević, A.S. Phenolic Composition, Antioxidant and Antimicrobial Activity of the Extracts from Prunus spinosa L. fruit. Hemijska Industrija 2014, 68, 297–303.