ABSTRACT
The sol–gel transition temperatures of high acyl gellan gum solution were investigated using rheaological measurements. The sol–gel transition temperatures were determined from three criteria: the crossover of storage modulus G′ and loss modulus G″, the linear extrapolation method, and the Winter’s criterion. The results showed that the Winter’s criterion was more suitable to determine the sol–gel transition temperature. The sol–gel transition temperature increased with the increasing gellan concentration. The relaxation critical exponent n can be determined by the Winter’s criterion. There is no universal n for the gelation in high acyl gellan gum solutions, indicating that this gelation belongs to the cross-linking of existing macromolecules. The values of n decreased with increasing concentration of gellan gum solution. The fractal dimension df calculated from n without the screened hydrodynamic interaction nor the excluded volume effect suggested a denser structure in the critical gel with higher c. The gelation enthalpy (ΔHgel) was calculated by Eldridge–Ferry model.
Introduction
Gellan gum is a novel bacterial polysaccharide produced by Pseudomonas elodea, which has been approved as a food additive in USA and other countries.[Citation1] The repeating unit of the gellan gum is a tetrasaccharide, which consists of two residues of d-glucose, one residue of l-rhamnose, and the one of d-glucuronic acid. The native gellan gum has an l-glyceryl substituent on O(2) of the 3-linked glucose residue of the tetrasaccharide sequence and, in some repeat units, an acetyl group at O(6) of the same residue.[Citation2] In normal commercial production, both types of substituent are removed by the hot alkali treatment on the fermentation broth. The resulting deacylated product is known as low acyl gellan. Most of the acyl groups can be preserved by use of milder extraction procedures giving high acyl gellan. Compared with other hydrocolloids, gellan has many peculiar advantages such as low dosage, excellent thermal and acid stability, good taste-releasing ability, and good combinability. Therefore, gellan gum has been widely applied in food industry as a thickener, emulsifier, stabilizer, foaming agent and textural modifier, and so on.[Citation3–Citation8]
Since the gelation process in aqueous solutions dominates the properties of gellan gum and therefore dictates its applications in food and other industries, the gelation process has been intensively investigated. During gelation, gellan molecules undergo a phase transition from liquid to gel, known as sol–gel transition. Up to now, various technologies have been developed to probe sol–gel transitions of food hydrocolloids, including rheometry,[Citation9] differential scanning calorimetry,[Citation10–Citation12] electron spin resonance,[Citation13] nuclear magnetic resonance,[Citation14,Citation15] and so on. In all of available methods, small deformation rheaology is preferred due to its easier performance and reproducible results without structure perturbing.[Citation16]
In parallel, several rheaological criteria have been proposed to estimate either the gel temperature or the gel time. Especially, the idea of gel point has received much attention in synthetic and biopolymer gels. In the classical theory, the attributes of gel are an infinite steady-shear viscosity and a zero equilibrium modulus at zero frequency limits.[Citation17] These criteria have been widely employed to study gel point of chemical gels. However, essential information based on viscosity measurement is hard to be obtained in the vicinity of the gel point because shearing affects or even destroys gel formation. Furthermore, experimentally, the steady-shear viscosity cannot be reached at gel point; the same question is encountered for zero equilibrium modulus measurement. In order to circumvent these obstacles, Tung et al. proposed that the gel point of a cross-linking polymer occurs at the time or temperature at which G′ and G″ cross each other at a given frequency.[Citation18] This method is widely used due to its easy operation with successful applications in many biopolymers systems.[Citation19,Citation20] The alternative and common way to characterize the gel point is the extrapolation of G′ value.[Citation21] Hsieh et al. determined Tgel of whey and egg proteins by extrapolation of the rapidly rising values of G′ to the temperature axis.[Citation22,Citation23] Later, Winter et al. found experimentally that at gel point G′ and G″ exhibited a power law on angular frequency ω as .[Citation24–Citation26] The corresponding relaxation modulus G(t) can be expressed as
, where S is the gel strength and n is the relaxation critical exponent. Thus,
, which means that tan δ is independent of ω at the gel point. The exponent n depends on the molecular structure and could be unitized to probe gel structures.
Since the behaviors in the sol–gel phase transition could provide a lot of information, which is crucial for the gellan gum application, up to now, a tremendous effort has been invested in this field,[Citation27–Citation30] whereas studies on the static and dynamic properties of high acyl gellan near the gel point are scarce reported. In this article, the sol–gel transition of high acyl gellan gum solution is determined by three rheaological criteria. Moreover, the relaxation exponent, fractal dimension, and gelation enthalpy of the critical gels were discussed to understand the gel structure of high acyl gellan gum. The results shed light on the role of gelling polymers on rheaological properties of homogeneous and phase-separated gel systems.
Materials and method
Materials
Food-grade high acyl gellan (Kelcogel LT-100p, CP Kelco, San Diego, USA) was utilized without further treatment. The contents of inorganic ions Na, K, Ca, and Mg were determined by ICP-AES (SPS8000, KCHG Co. Ltd.: Beijing, China) as Na 0.03%, K 0.78%, Ca 0.14%, Mg 0.03%, respectively. And, the content of acyl groups is 9.7 ± 0.02%. The gellan has the characteristics: molecular weights Mn = 1.32 × 106 and Mw = 1.49 × 106, which were assessed by GPC (PL-GPC 50, Agilent, UK); thus, the molecular weight distribution is 1.13.
Preparation of gellan solutions
The required amount of high acyl gellan powder was gradually added to the appropriate amount of distilled water and stirred at room temperature overnight to homogenize the suspension. Then, this homogeneous suspension was heated with constant stirring to obtain clear solutions at 90°C. Evaporative losses were corrected for by adding appropriate amounts of 90°C distilled water. The concentrations of high acyl gellan solution are 0.15, 0.5, 0.7, 1.0, and 1.5 wt%, respectively.
Rheaological measurements
The rheaological measurements were conducted to determine the gel point with a stress-controlled AR-G2 rheometer (TA Instruments, New Castle, Delaware, USA) using the parallel plates 60 mm in diameter. Temperature was controlled by a Peltier plate with an accuracy of ±0.1°C. A series of strain sweep tests was performed to estimate the zone of linear viscoelasticity of high acyl gellan solution at selected temperatures (60–86°C). The applied strain ranged from 0.25% to 25%, and the angular frequency was 10 rad/s. Hot high acyl gellan solutions were placed on the lower plate previously heated to the desired temperature and the gap between the parallel plates was adjusted to 1 mm. The rim of the plates was covered with silicon oil to minimize the water evaporation. Temperature sweeps were run at 10 rad/s and the cooling rate was 1°C/min. For temperature sweep test, the strain was appropriately changed in the range from 5% for the solution phase down to 1% for the gel phase to ensure the measurement within the linear viscoelastic range. Frequency sweep from 0.1 to 100 rad/s at fixed temperature was also carried out within the linear viscoelasticity region to determine the gel point.
Results and discussion
Observation of sol–gel transition
shows variation of G′ and G″ with angular frequency ω for 1.0 wt% high acyl gellan solutions at indicated temperatures. At high temperature, the high acyl gellan solution presents typical viscoelastic behavior of a semidilute polymer solution with G′ < G″ throughout the accessible frequency range, and G′ and G″ are strongly frequency dependent. The frequency dependence of the modulus tends to G′ ~ ω1.6 and G″ ~ ω0.9 at 80–82°C, which is a characteristic feature of dilute polymer solutions. While at low temperature, G′ becomes higher than G″ and a plateau appears indicating the three-dimension network formation. Therefore, the high acyl gellan solution forms gel with sol–gel transition occurring in cooling process.
Determination of sol–gel transition by dynamic rheaology method
G′ and G″ crossover
The sol–gel transition is one of the most relevant phenomena in food processing. Studies on thermosetting resins[Citation18] suggested that the gel point might occur at the time or temperature at which G′ and G″ cross each other at a given frequency. This criterion is successfully applied to the gelation of some polysaccharides, such as gelatin[Citation20] and κ-carrageenan gels.[Citation19] So, we used this criterion to determine the gelation temperature (Tgel,C) of high acyl gellan gum solution first.
presents the temperature dependence of G′ and G″ during temperature sweep on cooling at 1°C/min for the high acyl gellan solution at indicated concentrations c. It reveals that the sol–gel transition temperatures vary with the solution concentration. The beginning of the gelation process is dominated by the viscous behavior (G″ > G′) while the elastic behavior (G″ ≪ G′) did it in the final stage of experiment. Both moduli arise as a result of the increasing density of junction zones, but G′ rises more sharply until it intercepts and then exceeds G″. The variation of G′ and G″ upon cooling demonstrates the solution undergoing a transition from liquid-like properties to solid-like properties. Tgel,C of the high acyl gellan solution with various concentrations is listed in . It is obvious that the increase of high acyl gellan concentration shifts the crossover of G′ and G″ to higher temperatures which is quiet similar to the result of Flores-Huicochea et al.[Citation30] This is because gelation process is accelerated due to the polymer concentrated, in which the ensemble average distance between macromolecule molecules narrows down to speed up the physical bond formation rate between neighboring molecules.[Citation31]
Table 1. Sol–gel transition temperatures and critical exponent n of high acyl gellan solutions determined by different criteria.
Linear extrapolation method
The gradual development of the network structure is reflected by a progressive increase in storage modulus (G′). As shown in , G′ increases with temperature depressing, as a result of the density of junction zones increasing. When the sample is cooled to the critical temperature, G′ started to increase abruptly due to the rapid formation of junction zones in the polymer network. After this initial rapid stage, G′ shifts to continuous but mild uprising, due to the slow formation of junction zones and followed by their spatial rearrangement. But the rate of G′ enhancement becomes small with temperature further cooling down.
From the view of the classical viscoelasticity theory, the abrupt increase in G′ is used as an indication of the onset of the sol–gel transition. The initial rise in G′ is associated with the three-dimensional network formation where most of the sol fraction aggregates to build a gel. The gelation temperature Tgel,G′ of sample can be determined by linear extrapolation of the straight-line portion of each cooling curve back to the value G′ = 0 from the crossover with the x-axis (temperature). Tgel,G′ is presented in . The results displace that this value is significantly affected by the gellan concentration as Tgel,G′ increases with gellan concentrated in solutions. Meanwhile, Tgel,C is lower than Tgel,G′.
Winter’s criterion
Up to now, the most frequently used method for the gel point determination is the Winter’s criterion. The sol–gel transition is determined according to Winter’s criterion of via the interpolation method, where tan δ is independent of frequency. displays this interpolation with the data from as examples. It is found that the curves of tan δ do intersect at a temperature Tgel,ω. Consequently, the Winter’s criterion is available for determination of the sol–gel point in the high acyl gellan gum solutions. Tgel,ω and critical exponent n determined in this way are summarized in . n Values are estimated by the intersecting point of the tan δ versus temperature curve. Moreover, Tgel,ω is considerably larger than Tgel,C and Tgel,G′.
Figure 3. Tan δ versus the temperature of 1.0 wt% high acyl gellan gum. The gelling temperature Tgel,ω is estimated from the crossover of the frequency data.
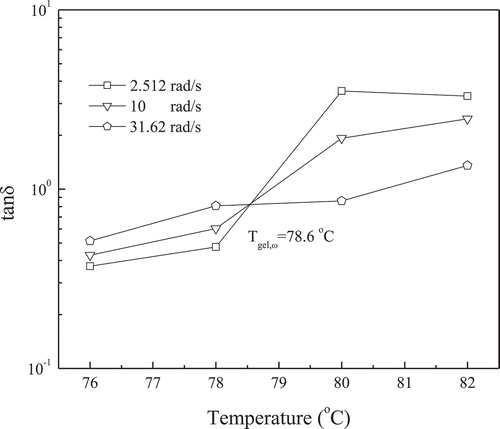
According to , G′ and G″ are strongly dependent on ω and of significant difference under different ω. On the basis of viscoelasticity theory, higher frequency corresponds to shorter time, so G′ and G″ values will be higher. As the gelation temperature is an intrinsic property of the material, it cannot be dependent on the frequency of the dynamic rheaological experiment. In addition, Chambon et al. found that the crossover of G′ and G″ can hardly present the real sol–gel transition because it was obtained at a single angular frequency.[Citation32] There is only one case that gel point coincides with the crossover of G′ and G″ in which the critical exponent n = 1/2. For those polymers with n ≠ 1/2, the crossover occurs before gel point for n < 1/2 or after gel point for n > 1/2.[Citation33] As a result, the crossover of G′ and G″ is not suitable to characterize the sol–gel transition of high acyl gellan solution. So compared to other two methods, Winter’s criterion is more appropriate to characterize the sol–gel transition of high acyl gellan.
Dai et al. studied the sol–gel transition of low acyl gellan solution and found that Tgel,ω was 33.1, 39.4, 45.6, 55.3°C for 1.0, 1.5, 2.0, 2.5 wt% low acyl gellan solutions, respectively.[Citation28] It is obvious that high acyl gellan solutions gelate at much higher temperature than low acyl gellan solutions when they are at the same concentration. The glyceryl groups are located inside the double helix, and the acetyl groups lie on its periphery. Additionally, the glyceryl groups allow double helix formation even at high temperatures (70–80°C) and the acetyl groups have the double helices.[Citation30]
Concentration dependence of sol–gel transition temperature and application of the Eldridge–Ferry equation
As abovementioned, the high acyl gellan gum system follows Winter’s criterion quite satisfactorily; therefore, the sol–gel transition temperature Tgel,ω of this type of solution could be assessed and is plotted in as a function of concentration c. It is found that the sol–gel transition temperature increases with high acyl gellan concentration increasing, and a relatively small amount of concentration change could induce great gelation temperature increase. Tgel,ω increases as the high acyl gellan gum concentration increases as well, due to the increase in cross-linking junctions between the gellan chains. The relationship between high acyl gellan concentration and the sol–gel transition temperature could be formulated by
where Tgel,ω is the absolute sol–gel transition temperature determined from Winter’s criterion, c is the high acyl gellan concentration (R2 = 0.9970, P < 0.001). Eldridge and Ferry[Citation34] studied the relationship between the melting point (Tm) and concentration (c) of gelatin gels. Assuming an equilibrium binary association of polymer chains and using the van’t Hoff law, they suggested the following relationship from which it is possible to calculate the enthalpy () of the cross-linking process and to get some information about the stability of the junction zones into gel.
This approach is satisfactorily applied to many biopolymer gelled systems, including agarose,[Citation35] LM pectin/Ca2+, HM pectin/Ca2+,[Citation36] and κ-carrageenan.[Citation37] Eq. (2) is applied to estimate the gelation enthalpy at the sol–gel transition point. For high acyl gellan gum, plots of ln c versus 1/Tgel,ω () shows good linearity. The gelation enthalpy (∆Hgel) is determined from the slope of the straight line of the Eldridge–Ferry plots shown in . The enthalpy change obtained in this way characterizes the enthalpy associated with the strongest network junction average over the concentration range, that is, the junction zones remain stable until the sol–gel transition is reached. Value of ∆Hgel obtained for high acyl gellan gum is 128.54 kJ/mol.
Concentration dependence of relaxation exponent and fractal dimension
From , it could be found that relaxation exponents n estimated from Winter’s criterion decrease with gellan concentrated. Furthermore, this value for physical gelation is not universal but spreads in the range of 0–1. In principle, it depends on not only the nature of gels but also the conditions of the aqueous environment. The sol–gel transition of physical gel formation in polysaccharides systems has been explored, and its critical exponents n near the gel point have been reported by Winter’s criterion as well. Hossain et al. investigated sol–gel transition behavior in pure ι-carrageenan solutions with the relaxation exponent ranging from 0.18 to 0.21 in salt-free states and from 0.17 to 0.24 in addition of potassium ions.[Citation38] Dai et al. reported the relaxation exponent to be 0.57–0.72 for low acyl gellan containing potassium ions and 0.37–0.63 with the presence of calcium ions at the gel point.[Citation39] The range of n for the critical high acyl gellan in this work is from 0.42 to 0.52, which is smaller than the universal value of 2/3 for the end-linking chemical gels. Chambon and Winter[Citation26] studied many systems with conclusion as n = 0.5 for stoichiometrically balanced gels while 1/2 < n < 1 for imbalanced gels. Smaller n value roughly indicates that the critical gel networks are much denser.
In order to further reveal the structure of the critical gel, we relate the relaxation exponent to the fractal dimension. Muthukumar[Citation40] investigated the cross-linking process for polydisperse systems using a hyperscaling relation developed by the percolation theory. If the hydrodynamic interaction as well as the excluded volume effect can be screened out completely, n is expressed as
where n is the critical exponent, df is the fractal dimension of the polymer, and d is the space dimension. If the hyperscaling assumption of the percolation model is valid, plus d = 3 for our study, df could be estimated since n is known.
Furthermore, in the framework of Eq. (3), values of the relaxation exponent in the range of 0 < n < 1 are possible with the corresponding fractal in the physically domain of 1 ≤ df ≤ 2.5. And, df are obtained with 1.98, 2.04, 2.05, 2.07, and 2.09 in 0.15, 0.5, 0.7, 1.0, and 1.5 wt% high acyl gellan solutions, respectively. df is small and slightly increases with the increase of c, indicating that in the critical high acyl gellan gel the cluster space is loose and becomes denser at higher c. The sol–gel transition of gellan gum is caused by the aggregation of double helix, so the double helix content will determine the intermolecular aggregation. It is well known that temperature and polymer concentration all affect the helix content in gellan gum solutions. At the same temperature, the high acyl gellan solution with higher concentration has more helix content, leading to more aggregation and denser critical gel. And, the weak dependence of df on c may come from the narrow available concentration range in the present experiments.
Conclusions
The sol–gel transition temperatures of 0.15–1.5 wt% high acyl gellan gum solution are investigated by using rheaological measurements. Three rheaological criteria are applied to estimate the sol–gel transition temperature, the crossover of storage modulus G′ and loss modulus G″, the linear extrapolation method, and the Winter’s criterion. The results show that the Winter’s criterion is more suitable to estimate the sol–gel transition of high acyl gellan. With the concentration of high acyl gellan gum uprising, the sol–gel transition temperatures Tgel,ω increase. The relaxation critical exponent n can be determined by the Winter’s criterion too. There is no universal n for the gelation in high acyl gellan aqueous solutions, indicating that this gelation belongs to the cross-linking of existing macromolecules. n Decreases with high acyl gellan concentrated. The fractal dimension df calculated from n without the screened hydrodynamic interaction nor the excluded volume effect suggests a denser structure in the critical gel with higher c. The gelation enthalpy (ΔHgel) is calculated by Eldridge–Ferry model, which is 128.54 kJ/mol for high acyl gellan gum solutions.
Additional information
Funding
References
- Morris, E. R.; Nishinari, K.; Rinaudo, M. Gelation of gellan-A Review. Food Hydrocolloids 2012, 28, 373–411.
- Matsukawa, S.; Watanabe, T. Gelation Mechanism and Network Structure of Mixed Solution of Low-And High-Acyl Gellan Studied by Dynamic Viscoelasticity, CD and NMR Measurements. Food Hydrocolloids 2007, 21, 1355–1361.
- Sherafati, M.; Kalbasi-Ashtar, A.; Mousavi, S. M. A. Effects of Low and High Acyl Gellan Gums on Engineering Properties of Carrot Juice. Journal of Food Process Engineering 2013, 36, 418–427.
- Buldo, P.; Benfeldt, C.; Carey, J. P.; Folkenberg, D. M.; Jensen, H. B.; Sieuwerts, S.; Vlachvei, K.; Ipsen, R. Interactions of Milk Proteins with Low and High Acyl Gellan: Effect on Microstructure and Textural Properties of Acidified Milk. Food Hydrocolloids 2016, 60, 225–231.
- Danalache, F.; Mata, P.; Moldao-Martins, M.; Alves, V. D. Novel Mango Bars Using Gellan Gum as Gelling Agent: Rheological and Microstructural Studies. LWT-Food Science and Technology 2015, 62, 576–583.
- Danalache, F.; Carvalho, C. Y.; Alves, V. D.; Moldao-Martins, M.; Mata, P. Optimisation of Gellan Gum Edible Coating for Ready-To-Eat Mango (Mangiferaindica L.) Bars. International Journal of Biological Macromolecules 2016, 84, 43–53.
- Jap, V.; Da Cunha, R. L. High Acyl Gellan as an Emulsion Stabilizer. Carbohydrate Polymers 2016, 139, 115–124.
- Iurciuc, C.; Savin, A.; Lungu, C.; Martin, P.; Popa, M. Gellan. Food Applications. Cellulose Chemistry Technology 2016, 50, 1–13.
- Tau, T.; Gunasekaran, S. Thermorheological Evaluation of Gelation of Gelatin with Sugar Substitutes. LWT-Food Science and Technology 2016, 69, 570–578.
- Miyoshi, E.; Takaya, T.; Nishinari, K. Effects of Glucose, Mannose and Konjacglucomannan on the Gel-Sol Transition in Gellan Gum Aqueous Solutions by Rheology and DSC. Polymer Gels & Networks 1998, 6, 273–290.
- Mdc, N.-S.; Tecante, A. Rheological and Calorimetric Study of the Sol-Gel Transition of κ-carrageenan. Carbohydrate Polymers 2007, 69, 763–773.
- Liu, S. J.; Bao, H. Q.; Li, L. Thermoreversible Gelation and Scaling Laws for Grapheneoxide-Filled Kappa-Carrageenan Hydrogels. European Polymer Journal 2016, 79, 150–162.
- Tsutsumi, A.; Ozawa, T.; Okada, M.; Hiraoki, T.; Ishii, F. Electron Spin Resonance Study on the Sol-Gel Transition of Gellan Gum Aqueous Solution by the Addition of Paramagnetic Metal-Ions. Progress in Colloid and Polymer Science 1999, 114, 31–35.
- Annaka, M.; Honda, J. I.; Nakahira, T.; Seki, H.; Tokita, M. Multinuclear NMR Study on the Sol-Gel Transition of Gellan Gum. Progress in Colloid and Polymer Science 1999, 114, 25–30.
- Hikichi, K.;. Sol-Gel Transition of κ-carrageenan as Viewed through NMR. Polymer Gels & Networks 1993, 1, 19–31.
- Nishinari, K.;. Sol-Gel Transition of Biopolymer Dispersions. Macromolecular Symposia 2000, 159, 205–214.
- Rao, M. A.; Hartel, R. W. Phase/State Transitions in Food: Chemical, Structural and Rheological Changes; Marcel Dekker Inc: New York, 1998; 111–158.
- Tung, C. Y. M.; Dynes, P. J. Relationship between Viscoelastic Properties and Gelation in Thermosetting Systems. Journal of Applied Polymer Science 1982, 27, 569–574.
- Stading, M.; Hermansson, A. M. Rheological Behaviour of Mixed Gels of κ-carrageenan-locust Bean Gum. Carbohydrate Polymers 1993, 22, 49–56.
- Djabourov, M.; Leblond, J.; Papon, P. Gelation of Aqueous Gelatin Solutions. II. Rheology of the Sol-Gel Transition. Journal De Physique 1988, 49, 333–343.
- Dahme, A. Gel Point Measurements on High-Methoxyl Pectin Gels by Different Techniques. Journal of Texture Studies 1992, 23, 1–11.
- Hsieh, Y. L.; Regenstein, J. M. Modeling Gelation of Egg Albumen and Ovalbumin. Journal of Food Science 1992, 57, 856–861.
- Y L, H.; J M, R.; Rao, M. A. Gel Point of Whey and Egg Proteins Using Dynamic Rheological Data. Journal of Food Science 1993, 58, 116–119.
- Winter, H. H.; Chambon, F. Analysis of Linear Viscoelasticity of a Crosslinking Polymer at the Gel Point. Journal of Rheology 1986, 30, 367–382.
- Winter, H. H.;. Transient Networks: Evaluation of Rheology during Chemical Gelation. Progress in Colloid and Polymer Science 1987, 75, 104–110.
- Chambon, F.; Winter, H. H. Linear Viscoelasticity at the Gel Point of a Crosslinking PDMS with Imbalanced Stoichiometry. Journal of Rheology 1987, 31, 683–697.
- Miyoshi, E.; Nishinari, K. Rheological and Thermal Properties near the Sol-Gel Transition of Gellan Gum Aqueous Solutions. Progress in Colloid and Polymer Science 1999, 14, 68–82.
- Dai, L.; Liu, X.; Liu, Y.; Tong, Z. Concentration Dependence of Critical Exponents for Gelation in Gellan Gum Aqueous Solutions upon Cooling. European Polymer Journal 2008, 44, 4012–4019.
- Huang, Y. Q.; Singh, P. P.; Tang, J. M.; Swanson, B. G. Gelling Temperatures of High Acyl Gellan as Affected by Monovalent and Divalent Cations with Dynamic Rheological Analysis. Carbohydrate Polymers 2004, 56, 27–33.
- Flores-Huicochea, E.; Rodríguez-Hernández, A. I.; Espinosa-Solares, T.; Tecante, A. Sol-Gel Transition Temperatures of High Acyl Gellan with Monovalent and Divalent Cations from Rheological Measurements. Food Hydrocolloids 2013, 31, 299–305.
- Tang, J.; Tung, M. A.; Zeng, Y. Gelling Properties of Gellan Solutions Containing Monovalent and Divalent Cations. Journal of Food Science 1997, 62, 688–712.
- Chambon, F.; Petrovic, Z. S.; Mac Knight, W. J.; Winter, H. H. Rheology of Model Polyurethanes at the Gel Point. Macromolecules 1986, 19, 2146–2149.
- Winter, H. H. Can the Gel Point of a Cross-Linking Polymer Be Detected by the G′-G” Crossover? Polymer Engineering & Science 1987, 27, 1698–1702.
- Eldridge, J. E.; Ferry, J. D. Studies of the Cross-Linking Process in Gelatin Gels. III. Dependence of Melting Point on Concentration and Molecular Weight. The Journal of Physical Chemistry 1954, 58, 992–995.
- Watase, M.; Nishinari, K. Dynamic Viscoelasticity and Anomalous Thermal Behaviour of Concentrated Agarose Gels. Die Makromolekulare Chemie 1987, 188, 1177–1186.
- Fu, J. T.; Rao, M. A. The Influence of Sucrose and Sorbitol on Gel-Sol Transition of Low-Methoxyl Pectin+ Ca2+ Gels. Food Hydrocolloids 1999, 13, 371–380.
- Watase, M.; Nishinari, K. Rheological and Thermal Properties of Carrageenan Gels. Effect of Sulfate Content. Die Makromolekulare Chemie 1987, 188, 2213–2221.
- Hossain, K. S.; Miyanaga, K.; Maeda, H.; Sol-Gel Transition, N. N. Behavior of Pure ι-Carrageenan in Both Salt-Free and Added Salt States. Biomacromolecules 2001, 2, 442–449.
- Dai, L.; Liu, X.; Tong, Z. Critical Behavior at Sol-Gel Transition in Gellan Gum Aqueous Solutions with KCl and CaCl of Different Concentrations. Carbohydrate Polymers 2010, 81, 207–212.
- Muthukumar, M.;. Screening Effect on Viscoelasticity near the Gel Point. Macromolecules 1989, 22, 4658–4660.