ABSTRACT
Placing Clanis bilineata larvae in cold storage results in denaturation of their myofibrillar (MF) proteins, which alters their texture. In this study, the effect of pullulan on denaturation of MF proteins in C. bilineata larvae frozen at −18°C for 6 months was investigated. Different amounts of pullulan (2.5–7.5 g/100 g wet weight) were added to MF proteins. Alterations in MF protein properties, such as unfrozen water content, sulfhydryl content, calcium-adenylpyrophosphatase activity, and solubility, during frozen storage were evaluated. Pullulan enhanced these properties, suggesting that it is a promising cryoprotectant for C. bilineata larvae meat during frozen storage.
Introduction
Clanis bilineata belongs to the subfamily Ambulicinae (Sphingidae, Lepidoptera). C. bilineata larvae are edible and nutritious. Dried C. bilineata has the following characteristics: protein content, 65.5% (w/w); essential amino acid ratio, 52.84% (w/w); fat content, 23.68% (w/w); ratio of unsaturated fatty acids, 64.17% (w/w); and linolenic acid, which is a functional fatty acid, 36.53% (w/w) of total fatty acids.[Citation1] China is the largest consumer of C. bilineata larvae, with annual consumption of approximately 6000 t.
C. bilineata larva meat is usually placed in frozen storage because of seasonality. However, the protein in C. bilineata larvae is prone to denaturation during frozen storage, resulting in deterioration of quality over time. Deteriorated meat has lower commercial and consumption values. Several studies have investigated the influence of various agents, such as sodium chloride, sodium nitrite, phosphate, amino acids, organic acids, protein hydrolysates, and sugars, on protein denaturation to explore ways to inhibit the denaturation process.[Citation2–Citation12]
Pullulan is a polysaccharide composed mainly of maltotriose repeating units interconnected by α-(1→6) linkages (). Pullulan possesses high water solubility and structural flexibility[Citation13] and has been widely applied in the food, cosmetic, and medical industries.[Citation14] However, data regarding the effects of pullulan addition on protein denaturation at freezing conditions are limited. In this study, the protective effects of pullulan against protein denaturation in C. bilineata larva meat subjected to freezing conditions were investigated. Alterations in unfrozen water, Ca-adenylpyrophosphatase (ATPase) activity, sulfhydryl content, and solubility of proteins were examined.
Materials and methods
Materials
Fifth instar larvae of C. bilineata were purchased from a local agricultural market in Yishan City, Lianyungang, China. All reagents used were of analytical grade.
Preparation of myofibrillar (MF) protein
MF proteins of C. bilineata larvae were prepared according to the method described by Hossain et al.[Citation4] Pullulan (2.5–7.5 g/100 g wet weight) was added to MF protein samples. Sodium hydroxide (0.01 M) was added to the mixture to adjust pH to 7.0. The resulting solution was mixed at ~3°C for 20 min. Approximately 5 g of the mixture was then sealed in a test tube (inner diameter, 12 mm; length, 75 mm) and stored at –18°C for 6 months. Control samples consisting of C. bilineata larvae MF proteins without pullulan were stored similarly. The approximate components of MF proteins of C. bilineata larvae were 85.76% water, 13.57% crude protein, 0.03% crude lipid, and 0.37% crude ash.
Measurement of unfrozen water
The unfrozen water content in frozen C. bilineata larvae MF was determined through methods described previously by Yamashita et al.[Citation11] The heat of fusion of 5–25 mg of distilled water was determined by raising the temperature from −40°C to 25°C at a rate of 1°C/min until a linear relationship was observed between the amount of water and the heat of fusion (79.9 cal/g H2O). Frozen MF protein (20 mg) was placed in a tightly sealed aluminium cell and weighed. The heat of fusion of water in the frozen MF was also measured by an identical method. The amount of unfrozen water is considered bound water and partially bound water.
Determination of sulfhydryl content
Sulfhydryl content was assayed according to the method of Slyer et al.[Citation15] MF protein (1 g) was mixed with 50 mL of cold distilled water and then homogenized. The protein in the homogenate was diluted to 2 mg/mL with 0.1 M phosphate buffer (pH 7.4). Protein content in the homogenate was assayed by the biuret method. Approximately 0.5 mL of the homogenate was transferred to a tube and dissolved in urea buffer (1:1). After incubation with 0.5 mL of 5,5ˊ-dithiobis (2-nitrobenzoic acid) at room temperature for 15 min, the absorbance of the solution was measured at 412 nm. Sample blanks with 0.5 mL of phosphate buffer without 5,5ˊ-dithiobis (2-nitrobenzoic acid) and reagent blanks with only water were prepared. Sulfhydryl content was calculated using a molar extinction coefficient of 11,400 M−1 cm−1 for 5,5ˊ-dithiobis at this wavelength. The results, expressed in ŋmol, indicated the total free sulfhydryl groups per 1 mg of protein.
Ca-ATPase activity determination
MF protein samples were subjected to different frozen storage periods. The samples were treated according to the procedures described by Yamashita et al.[Citation11] The Ca-ATPase activity of the MF proteins was measured according to the methods of Katoh et al.[Citation16] The samples (0.2–0.4 mg) were incubated at 25°C with 100 mM KCl, 5 mM CaCl2, 25 mM Tris–maleate (pH 7.0), and 1 mM adenosine triphosphate. The reaction was terminated by adding 30% (V/V) trichloroacetic acid to a final concentration of 5% (v/v). The inorganic phosphate liberated in the supernatant was determined according to the method of Fiske and Subbarow.[Citation17] Measurement results were expressed as the ratio of the specific activity before freezing (relative %). The ratio was expressed as micromoles of inorganic phosphate released per 1 mg of protein per minute.
Determination of solubilisation of MF protein
After being subjected to various frozen storage conditions, the samples were centrifuged at 9000 × g at 4°C for 60 min. The protein concentration in the supernatant was then measured to obtain the concentration of salt-soluble protein. This value was used to calculate the percentage of the initial MF proteins remaining after frozen storage.
Analytical methods
The pH of the solution was recorded using a digital pH meter (Model: PHS-3C, CD Instruments, China). Protein concentrations were determined using the biuret method, and bovine serum albumin was used as a standard.[Citation18]
Statistical analysis
All data are presented as mean ± standard deviation. Statistical analysis was performed using Statgraphics Centurion XV version 15.1.02. Multifactor ANOVA with posterior multiple range test was used to determine significant differences among the groups.
Results
Changes in unfrozen water contents in the C. bilineata larvae MF samples are shown in . The unfrozen water content in the control samples sharply decreased initially to approximately 0.32% over the first 2 months of frozen storage and then levelled off. In the pullulan-containing group, although the unfrozen water contents in the C. bilineata larvae MF samples decreased slowly, they were consistently higher than those of the control group during the entire frozen storage period. The sulfhydryl contents in all MF protein samples decreased during the entire frozen storage period (), but decreased sharply only in the initial 2 months of frozen storage in the control group. The sulfhydryl contents in the experimental groups were consistently higher than those of the control group during the entire frozen storage period despite gradual decrease in these contents in the experimental groups.
Figure 2. Effect of pullulan on unfrozen water content in the Clanis bilineata larvae myofibrillar during frozen storage. Bars represent the standard deviation. Data are shown as mean ± SD (n = 3).
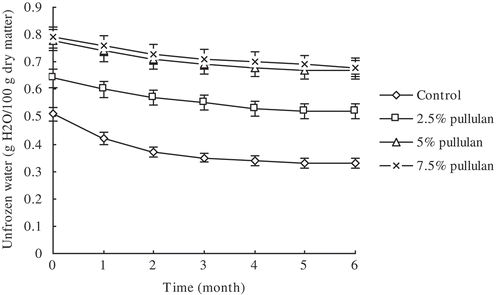
Figure 3. Effect of pullulan on the Clanis bilineata larvae myofibrillar sulfhydryl content during frozen storage. Bars represent the standard deviation. Data are shown as mean ± SD (n = 3).
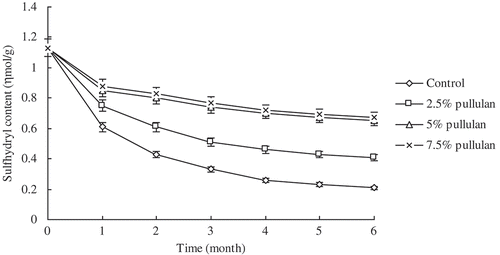
The Ca-ATPase activity of the control group decreased sharply in the first 2 months and decreased gradually thereafter. The Ca-ATPase activity of the pullulan-containing groups was higher than those of the control group during the entire frozen storage period ().
Figure 4. Effect of pullulan on the Ca-ATPase activity of the Clanis Bilineata larvae myofibrillar during frozen storage. Bars represent the standard deviation. Data are shown as mean ± SD (n = 3).
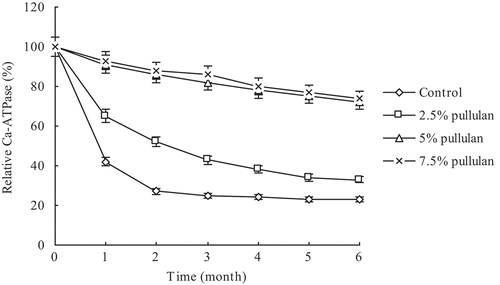
The solubility of the MF protein in all samples decreased throughout the frozen storage period (). The solubility of the control group decreased sharply during the initial 2 months of frozen storage and then levelled off. By contrast, the solubilities of the pullulan-containing groups decreased gradually during the entire frozen storage, and the values were higher than those of the control group (; p < 0.05).
Discussion
Proteins are three-dimensional structures formed and stabilized by several factors, such as hydrophobic interactions, hydrogen bonds, and hydration of polar residues. Thus, disrupting these factors alters the protein structures and can lead to loss of physiological activities, such as biocatalytic activity. Protein denaturation during freezing can be induced by various physical and chemical factors, such as changes in pH, high osmotic pressure, dehydration, and interactions with hydrolase.[Citation4] Sugars can stabilize proteins by stabilizing the structure of the water surrounding the proteins. This stabilizing effect depends on the concentration of sugar in the liquid.[Citation5]
Unfrozen water content is a critical index for the stability of meat-based food. High unfrozen water content indicates adequate binding water, leading to the preservation of protein structures during frozen storage.[Citation4] In the present study, the pullulan-containing group showed higher unfrozen water contents than the control group. This characteristic indicates that pullulan can constrain the water in the samples and consequently inhibit the denaturation of the C. bilineata larva MF proteins.
Formation of ice crystals alters the three-dimensional structures of MF proteins, which exposes the sulfhydryl groups at the intramolecular level. Oxidation of sulfhydryl groups leads to formation of disulfide bonds, which consequently decreases the sulfhydryl content of samples in frozen storage. We found that addition of pullulan inhibited the oxidation of sulfhydryl groups, preventing decrease in sulfhydryl content. These effects stabilized the C. bilineata larva MF proteins during frozen storage.
We investigated the denaturation of frozen C. bilineata larva MF protein in the presence of pullulan by measuring Ca-ATPase activity. Addition of pullulan increased Ca-ATPase activity of the MF protein, but no increase was observed in the control group. These findings indicated that inactivation of Ca-ATPase activity in the control group of the C. bilineata larvae MF protein followed two first-order equations.
Addition of pullulan also increased solubility, unfrozen water content, sulfhydryl content, and Ca-ATPase activity of MF proteins during frozen storage in a dose-dependent manner. The 5% and 7.5% pullulan groups showed higher efficiencies than the 2.5% pullulan group. Notably, no significant difference was observed between the 5% and 7.5% pullulan groups, indicating that 5% pullulan was the optimum amount for use during frozen storage.
Conclusions
The effects of pullulan on denaturation of C. bilineata larva MF proteins during frozen storage were investigated. Addition of pullulan effectively inhibited denaturation of frozen MF proteins in a dose-dependent manner. Thus, pullulan is a potential food cryoprotectant agent.
Additional information
Funding
References
- Wu, S. J.; Men, X.; Chen, S. J. The Analysis and Evaluation of Main Nutrient Components for Herse Bilineata tsingtauica. Journal of Huaihai Institute of Technology 2000, 9, 58–61. (in Chinese).
- Dihort-García, G.; Tolano-Villaverde, I. J.; Ezquerra-Brauer, J. M.; Ocaño-Higuera, V. M.; Ramírez De León, J. A.; Torres-Arreola, W.; Marquez-Rios, E. Effects of pH and Sodium Chloride on the Gelling Properties of a Protein Concentrate Obtained from Jumbo Squid Mantle (Dosidicus gigas). International Journal of Food Properties 2016, 19, 314–325.
- Alford, L.; Kannan, G.; Kouakou, B.; Lee, J. H. Curing Properties of Sodium Nitrite in Restructured Goat Meat (Chevon) Jerky. International Journal of Food Properties 2017, 20, 526–537.
- Hossain, M. A.; Alikhan, M. A.; Ishihara, T.; Hara, K.; Osatomi, K.; Osaka, K.; Nazaki, Y. Effect of Proteolytic Squid Protein Hydrolysate on the State of Water and Denaturation of Lizardfish (Saurida wanieso) Myofibrillar Protein during Freezing. Innovative Food Science & Emerging Technologies 2004, 5, 73–79.
- Matsumoto, I.; Arai, K. Cooperative Effect of Carboxylic Acid and Sugar against Freeze Denaturation of Fish Myofibrillar Protein. Nippon Suisan Gakkaishi 1987, 53, 2187–2193.
- Nozaki, Y.; Ichikawa, H.; Tabata, Y. Effect of Amino Acids on the State of Water and ATPase Activity Accompanying Dehydration of Fish Myofibrils. Nippon Suisan Gakkaishi 1991, 57, 1531–1537.
- Ooizumui, T.; Hashimoto, K.; Ogura, J.; Arai, K. Quantitative Aspect for Protective Effect of Sugar and Sugar Alcohol against Denaturation of Fish Myofibrils. Nippon Suisan Gakkaishi 1981, 47, 901–908.
- Pan, S. K.; Wu, S. J. Effect of Chitooligosaccharides on the Denaturation of Weever Myofibrillar Protein during Frozen Storage. International Journal of Biological Macromolecules 2014, 65, 549–552.
- Wang, H. B.; Pan, S. K.; Wu, S. J. Chitooligosaccharides Suppress the Freeze-Denaturation of Actomyosin in Aristichthys nobilis Surimi Protein. International Journal of Biological Macromolecules 2014, 63, 104–106.
- Wu, S. J.; Pan, S. K.; Wang, H. B. Effect of Trehalose on Lateolabrax japonicus Myofibrillar Protein during Frozen Storage. Food Chemistry 2014, 160, 281–285.
- Yamashita, Y.; Zhang, N.; Nozaki, Y. Effect of Chitin Hydrolysate on the Denaturation of Lizard Fish Myofibrillar Protein and the State of Water during Frozen Storage. Food Hydrocolloids 2003, 17, 569–576.
- Wu, S. J.;. Effect of Trehalose on the State of Water, Protein Denaturation and Gel-Forming Ability of Weever Surimi. International Journal of Food Properties 2016, 19, 521–525.
- Sugumaran, K. R.; Ponnusami, V. Statistical Modeling of Pullulan Production and Its Application Inpullulan Acetate Nanoparticles Synthesis. International Journal of Biological Macromolecules 2015, 81, 867–876.
- Prajapati, V. D.; Girish, K.; Jani, G. K.; Khanda, S. M. Pullulan: An Exopolysaccharideand Its Various Applications. Carbohydrate Polymers 2013, 95, 540–549.
- Soyer, A.; Özalp, B.; Dalmış, Ü.; Bilgin, V. Effects of Freezing Temperature and Duration of Frozen Storage on Lipid and Protein Oxidation in Chicken Meat. Food Chemistry 2010, 120, 1025–1030.
- Katoh, N.; Uchiyama, H.; Tsukamoto, S.; Arai, K.; Biochemical, A. Study on Fish Myofibrillar ATPase. Nippon Suisan Gakkaishi 1977, 43, 857–867.
- Fiske, C. H.; Subbarow, Y. The Colorimetric Determination of Phosphorus. Journal of Biological Chemistry 1925, 66, 375–400.
- Robinson, H. W.; Hodgen, C. G. The Biuret Reaction in the Determination of Serum Proteins. Journal of Biological Chemistry 1940, 135, 707–725.