ABSTRACT
Retrogradation is a common occurrence of starchy food and many methods have been tried to retard it. Uncovering the crystalline structure of retrograded starch provides us the necessary information to get an effective method. In this paper, the crystals of retrograded sweet potato starch with sharp X-ray diffraction peaks were obtained by blending sweet potato amylose and amylopectin with narrow distribution of molecular weight. The 2θ angles of those crystals were at 14.7°, 20.7°, 24.3°, 26.5°, and 29.9°. Intervals between two branched chains in retrograded amylose were 0.606, 0.348 nm, and in retrograded amylopectin 0.336 nm. A possible mechanism for the formation of those crystals was proposed based on analyzing 1H NMR, 13C NMR, X-ray diffraction, and differential scanning calorimeter of those samples. Cocrystallized amylose and amylopectin was necessary to prepare crystals of retrograded starch. More ordered arrangements exist in retrograded starch of sweet potato amylose and amylopectin.
Introduction
Starchy foods are usually heated and cooled many times when they are not eaten up. Such temperature-cycled treatment increases the starch retrogradation rate and melting enthalpy,[Citation1,Citation2] which causes the deterioration of food. The interaction of amylose and amylopectin during retrogradation might be one reason. Several authors have suggested that interactions between amylose and amylopectin happened during retrogradation.[Citation3,Citation4] X-ray diffraction studies suggest that intermolecular association involves a recrystallization process, and the amylopectin in the amylose–amylopectin–water system can be considered as a precipitating agent facilitating the aggregation of amylose.[Citation5] At low amylopectin content, the amylose component functions as nuclei and/or cocrystallizes with the amylopectin to some degree.[Citation6] Such an amylose–amylopectin association has also been determined by differential scanning calorimeter (DSC). The amylose and amylopectin endotherms have peak temperature of ≈140 and <120°C, respectively,[Citation7] whereas blending of them results in films with a second endotherm at 110–120°C. This endotherm results from the melting of cocrystallized amylose and amylopectin and of crystallized amylopectin.[Citation8] Preparation of crystals of retrograded starch with sharp X-ray diffraction is a possible way to know such association well, but the wide molecular weight distribution of starch is a great hindrance. For synthetic amylose, an abrupt change from the B- to the A-pattern happens when its Degree of Polymerization (DP) reduces from 13 to 12.[Citation9] The chain length of starch also has effects on the polymorphic forms of starch crystalline structures.[Citation10,Citation11] Some authors report that the retrograded waxy wheat starch from dual-retrogradation treatment in single-, dual-, and triple-retrogradation groups is more resistant to amylase than other groups.[Citation12] The cause of this phenomenon is still unknown. In this paper, the sweet potato amylose/amylopectin with narrower distribution of molecular weight was made by retrogradation–hydrolysis for 1–4 duplications,[Citation13] and the interaction of those amylose and amylopectin during retrogradation is investigated by 1H NMR, 13C solid-state NMR, X-ray diffraction, and DSC. The properties of retrograded starch containing both amylose and amylopectin with narrow distribution of molecular weight in the paper might explain why the dual-retrogradation waxy wheat starch is more resistant to hydrolysis. Furthermore, a possible mechanism for formation of retrograded sweet potato starch is deduced and the results in the paper will bring a new insight on the role of amylose and amylopectin during retrogradation. The interplanar distance between two crystal planes corresponding to pronounced peak at 2θ = 26.0 is very close to that of graphite,[Citation13] also indicating why retrograded starch could substitute for graphite in Li-ion battery in our experiments at present (). Although the ion capacity and charge–discharge cycle are not very well, the Li-ion battery with retrograded potato amylopectin as cathodes surely has the charge and discharge capacity.
Materials and methods
Materials
Sweet potato starch (moisture content 19.3%, amylose content 23.6%) was offered by Shan Dong Jin-Cheng Co., Ltd. Butanol and ethanol were purchased from Tianjin Fuyu Fine Chemical Co., Ltd. Thermostable α-amylase (12,000 U/mL) from Bacillus licheniformis was purchased from Tianjin Nuoao Technology Development Limited Company. Pullulanase microbial from Bacillus subtilis (Enzyme Commission Number 3.2.1.41; 5000 U/mL), maltose, maltotriose, maltotetrose, maltopentaose, maltohexaose, and maltoheptaose were purchased from Sigma-Aldrich Co. LLC.
Methods
Preparation of repeatedly retrograded sweet potato amylose and amylopectin: The repeatedly retrograded sweet potato amylose and amylopectin was prepared according to Ref. [Citation13–Citation16]. An amount of 10 g sweet potato starch blended with 100 mL distilled water was gelatinized for 20 min at 95°C by continuous stirring. Gelatinized starches were autoclaved at 120°C for 30 min and retrograded at 4°C for 24 h. Then, 0.6 mL high-temperature α-amylase (12,000 U/mL) was added to hydrolyze not retrograded starch into soluble carbohydrates for 6 h at 90°C. Crude retrograded sweet potato starch was precipitated by centrifuge (3040 × g for 5 min) and the supernatant fraction was discarded. The purified retrograded starches were dissolved in 4 M potassium hydroxide followed by neutralizing with 6 M hydrochloric acid. Then, the amylose in retrograded sweet potato starch was precipitated from the solution by adding three times the volume of 1-butanol, further isolation was done by centrifuge (3040 × g for 5 min). The supernatants, containing amylopectin, were collected, concentrated, and precipitated with excess ethanol. The amylopectin in supernatants was isolated by centrifuge (3040 × g for 5 min). The repeatedly retrograded samples (three times) derived from above sweet potato amylose and amylopectin were prepared under the same treatment of those retrograded sweet potato starches, the only difference was that middle-temperature α-amylase was used for purifying retrograded amylopectin. So, four kinds of sweet potato amylose and amylopectin were obtained corresponding to first-, duel-, tertiary-, and quaternary-retrograded samples. They were named amylose-1, amylose-2, amylose-3, amylose-4 and amylopectin-1, amylopectin-2, amylopectin-3, amylopectin-4, respectively.
Retrogradation of mixture of different sweet potato amylose and amylopectin: Mixture of above sweet potato amylose-4 and amylopectin-4 was blended at the ratio of 1:1 (w/w dry-starch basis) and retrograded at the same condition in Ref. [Citation13] and the dry method was also the same as that of retrograded sweet potato starch.
NMR: The samples have been analyzed with 1H NMR and 13C solid-state NMR. A sample of dried starch is first resolved in small amount of 4 M KOH and put into deuterated water (D2O) at 1.5–5.0 w/w%. The mixture is heated in a water batch (60°C) and shaken till clear homogeneity. 1H NMR spectra of these samples were recorded on a Mercury Vx-300 MHz machine (Varian, USA) operating at 300.07 MHz for the 1H nucleus, 75.45 MHz for the 13C nucleus, with a 45.0° pulse and a relaxation delay time of 1.0 s. 13C solid-state NMR spectra were acquired on a Varian Unity 300 MHz spectrometer. The dried starches were packed into 5-mm rotors at room temperature, and the 13C rf field strength was 75 kHz, which corresponded to a 90° pulse width of 3.4 μs whereas the spinning rate of MAS was set at a value in the range of 9–12 kHz.
X-ray diffraction: The X-ray patterns of the starches were obtained with copper, nickel foil filtered, and Ka radiation using a diffractometer (D-500 Siemens, Madison, WI, USA) following the method in Ref. [Citation17]. The diffractometer was operated at 27 mA and 50 kV. The scanning region of the diffraction angle (2θ) was from 5 to 60 at 0.04 step size with a count time of 2 s. Retrograded potato starches treated by different methods were equilibrated at 100% relative humidity for 24 h at 25°C prior to examination. Correspondence between diffraction angle (θ) and wavelength is given by the Braggs equation
2dsinθ = nλ
where d is the reticular distance between crystal planes in nanometers, θ is the diffraction angle in degrees, n (n = 1) is a numeric factor, and λ (λ = 0.154 nm) is the wavelength in nanometers.
DSC analysis: Melting parameters of different starches were measured using a DSC (DSC 204 HP, Netzsch, Germany) equipped with a thermal analysis data station and data recording software. The scanning temperature range and the heating rates were 0–250°C and 10°C/min, respectively. In all measurements, the starch samples were adjusted to 1:3 flour-to-water ratios and each starch slurry (4.0 mg starch in 12 µL distilled water) was transferred to an hermetically sealed, pressure rated pans (Mettler, ME-27331), and an empty pan was used as reference for all measurements. The transition temperatures reported were the onset (To), peak (Tp), and conclusion (Tc). The endothermic enthalpy of samples (ΔH) was estimated by integrating the area between the thermogram and a baseline under the peak and was expressed in terms of Joules per gram of dry starch. Three replicates per sample were analyzed.
Statistical analysis
The DSC traces were determined in triplicate. The averages and Duncan t-test were computed to measure variations in all samples. The least significant difference at the 5% probability level (P < 0.05) was calculated for each parameter.
Results and discussion
1H nuclear magnetic resonance spectroscopy
shows the chemical shifts of 1H NMR of sweet potato amylose (A1–A4) and amylopectin (B1–B4) treated by retrogradation-hydrolysis for 1–4 times. The proton spectra in all samples in show a large water peak (due to residual H2O in KOH). Absence of resonance signal in the range of 5.6 and 4.5 ppm, which is assigned to the –OH groups from glucose unit of starch[Citation18] in all samples in , indicates that they have fast exchanged with the water peak.[Citation19] The peaks at 3.47, 3.19 (3.17), and 3.05 (3.06) ppm in A4 and B4 are attributed to H-3/H-5, H-6, and H-2/H-4.[Citation20,Citation21] During the repeated retrogradation hydrolysis, however, those overlapping peaks in samples of amylose and amylopectin retrograding for 2–4 times separate gradually, which causes the peaks assigned to H-2/H-4 to separate from overlapping peaks. Absence of peaks around 5.0 assigned to H-1 in native starch[Citation21] in indicates that the proton of reducing end (C1) of sweet potato amylose and amylopectin has probably formed a solid-like zone[Citation18] during retrogradation, and such bonds keep locked even the samples are resolved in solutions at 60°C.
Figure 2. 1H NMR of sweet potato amylose (A1–A4) and amylopectin (B1–B4) during repeated retrogradation-hydrolysis. A1: Amylose retrogrades first; A2: Amylose retrogrades for second time; A3: Amylose retrogrades for third time; A4: Amylose retrogrades for fourth time; B1: Amylopectin retrogrades first; B2: Amylopectin retrogrades for second time; B3: Amylopectin retrogrades for third time; B4: Amylopectin retrogrades for fourth time.
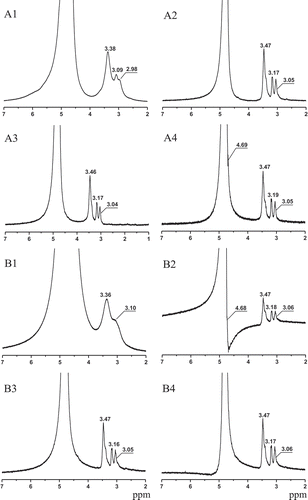
13C nuclear magnetic resonance spectroscopy
shows the chemical shifts of 13C solid-state NMR of sweet potato amylose (A4), amylopectin (B4), and their mixtures. Such results agree well with Ref. [Citation22]. Assignments of the resonance were consistent with literature data.[Citation23,Citation24] Signals at 101.6 and 62.6 are attributed to C1 and C6 in hexapyranoses, respectively. The overlapping signal at 73.5 ppm is associated with C2, C3, and C5. Compared with Refs. [Citation22–Citation25], the obvious differences in are the narrower line-widths of peaks and absence of resonances at −103 and 80–83 ppm (representing amorphous domains). The former indicates the higher crystallinity of retrograded sweet potato starches,[Citation25] the latter shows that there is nearly no detectable amorphous component in those starches, which is the same as that in hydrated, highly crystalline starch samples.[Citation24,Citation26]
An A-type crystal presents three resonances at 102, 101, and 100 ppm, whereas in a B-type conformation, the C1 resonance exhibits two resonances at 101 and 100 ppm, this corresponds to the nonidentical sugar residues of amylose and amylopectin. According to Ref. [Citation27], the C1 resonance is a triplet at 102, 101, and 100 ppm for the A-type crystalline polymorph, and a doublet at 101 and 100 ppm for B-type crystalline starch, but only one C1 resonances exists in ; so, the crystalline pattern of retrograded sweet potato starch is different from those of A or B-type crystalline.
Compared with the resonance of sweet potato amylose and amylopectin before and after blending in , one can easily find that there is a small resonance at 93.9 ppm in sweet potato amylose and amylopectin, but the resonance disappears after blending. The resonance represents the signals from single helix and amorphous structures.[Citation21,Citation28,Citation29] Then, the results in suggest that interaction of amylose and amylopectin during retrogradation can reduce single helix and amorphous domain of retrograded starch. Such resistant starch might be more resistant to amylase in our body and potentially be used to prepare functional food.
XRD analysis
The X-ray diffractograms of the sweet potato amylose and amylopectin are presented in a. For sweet potato amylose, the strong diffraction peaks at 2θ of 28°, 40°, 50°, 58°, 66°, and 73° are assigned to the face-centered cubic lattice of KCl[Citation30] in repeatedly retrograded starches in a A1–A4. Presence of KCl was from isolation of amylose and amylopectin in retrograded starch, in which KOH was used to resolve retrograded starch and HCl was added then to adjust its pH value. After the quantic-retrogradation, the intensities of those peaks become weak and diffraction peaks appear at 2θ of 28°, 42°, 49°, 54°, and 63°, indicating that the defective crystal structure of KCl appears and less KCl exists in those samples. In the first to the third retrogradation-hydrolysis, the retrograded sweet potato amylose shows diffraction peaks at 2θ of 14.9°, 19.3°, 20.2°, 24.4°, 26.7°, 29.6°, 30.1°, 31.7°, 36.0°, and 38.3°, as to the fourth retrogradation-hydrolysis, they shift to sharp peaks at 2θ of 14.6° (d = 0.606 nm), 25.6° (d = 0.348 nm), 29.6° (d = 0.302 nm), and 31.8° (d = 0.282 nm). The sharpness of those peaks and the loss of peaks at 36.0° and 38.3° infer the crystal with well-defined morphology of sweet potato amylose has formed. During repeated retrogradation-hydrolysis, the long chains in retrograded amylose could not have the same chance to form hydrogen bonds with other ones; so, parts of those chains will not involve in hydrogen bond forming and be hydrolyzed by amylase. When the chains are cut to certain length, all the chains and self-chains will form hydrogen bonds. In this condition, those chains with same length will form hydrogen bonds quickly, providing the chance to form certain crystal plane basing on hydrogen bond. Such results agree well with Ref. [Citation31], which demonstrates that the proportion of short chains (~10–13 glucose units) appears to affect crystal type significantly. A-type crystalline structure of starch is with diffraction peaks at 2θ of 15°, 17°, 20°, and 23°; for B type, 5°, 17°, 20°, 22°, and 24°; for C type, combination of A and B type; for a typical V-type crystalline, at 7°, 13°, and 20°.[Citation32] The crystal pattern of the sweet potato amylose in the quantic-retrogradation is totally different from those reported in Ref. [Citation33]. Compared with the single crystal of amylose,[Citation34] obviously there are only five planes in the crystal and its d-spacing is closer. The reason for this should be further studied. For sweet potato amylopectin, the intensity of KCl diffraction peaks at 2θ of 28.1°, 40.3°, 50.0°, 58.4°, 66.2°, and 73.5° becomes weak, which might be caused by the hindering of the movement of K+ or Cl− to gather on crystal nucleus and to form crystal. The retrograded sweet potato amylopectin shows diffraction peaks at 2θ of ~13.8° (d = 0.641 nm), ~16.9° (d = 0.524 nm), ~20.9° (d = 0.425 nm), ~22.1° (d = 0.403 nm), and ~26.6° (d = 0.334 nm), and the diffraction intensity of the sharp peak at ~26.6° is the most pronounced angle, suggesting that the long arrangement structure mainly consists of such planes. Such structure of sweet potato amylopectin is different from those in granule[Citation31] and similar to A-type crystallinity.[Citation33]
Figure 4. X-ray diffraction of sweet potato amylose (A1–A4), amylopectin (B1–B4)(a) and mixture of amylose and amylopectin(b)(c).
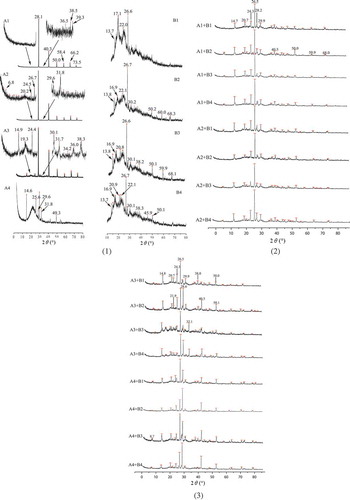
b and 4c show the X-ray diffraction of the blending of sweet potato amylose and amylopectin. The results show that the interaction of sweet potato amylose and amylopectin during retrogradation gives rise to crystal with 2θ angles at 14.7° (d = 0.601 nm), 20.7° (d = 0.428 nm), 24.3° (d = 0.365 nm), 26.5° (d = 0.336 nm), and 29.9° (d = 0.298 nm), which is different from that of A-, B-, C-, or V-type pattern.[Citation35–Citation37] According to literature, retrograded corn/pulse starch displays a typical B + V[Citation35] or A + B-type[Citation37] crystalline structure, which is obviously distinct from the results in b and 4c. The reason for this seems to derive from different source of starch, wide distribution of starch chain and retrogradation conditions, etc. Compared to the results in , it can be seen that the signals at 14.7° and 24.3° are probably from sweet potato amylose, 26.5° from amylopectin, and 20.7° and 29.9° from both of two. Situ et al.[Citation38] report that the peak at around 2θ = 26.0° becomes more pronounced as the resistant starch content increases, which agrees well with our results. The reticular distance between crystal planes corresponding to this angle is 0.336 nm, which is very close to that of graphite.[Citation13] This explains why retrograded starch could substitute for graphite in Li-ion battery in our experiments at present (data not shown). The absence of broad peaks in b and c suggests that the blending of sweet potato amylose and amylopectin is necessary to prepare crystal of retrograded starch with sharp peaks. For biomacromolecule, the presence of crystal similar to mineral salt is unusual and there are many possible applications in industry for this new material.
Thermal properties
Thermal properties of sweet potato amylose, amylopectin, and mixture of both were investigated using DSC and are depicted in and . The results in show that the melting peak temperature (Tp) of retrograded sweet potato amylose raises from 119.2 to 131.8°C as the amylose retrogrades from the first to the third time. However, when the amylose retrogrades for the fourth time, such data decrease to 127.1°C. According to literature,[Citation7,Citation39] amylose endotherm had a peak temperature higher than 120°C, the results in and a agree well with them. The Tp difference of different sweet potato amyloses could be attributed to their various degree of crystallinity, with those from the third retrogradation having the highest ones. In other words, a more perfect recrystallization is obtained in the group, although crystallization degrees of all samples in the paper are not obviously distinctive (data not shown, all around 45%).
Table 1. Mean thermal properties of sweet potato amylose/amylopectin with multiple retrogradation.
Figure 5. DSC of sweet potato amylose (A1–A4), amylopectin (B1-B4) (1) and mixture of sweet potato amylose and amylopectin (1:1 g/g) during repeated retrogradation-hydrolysis (2).
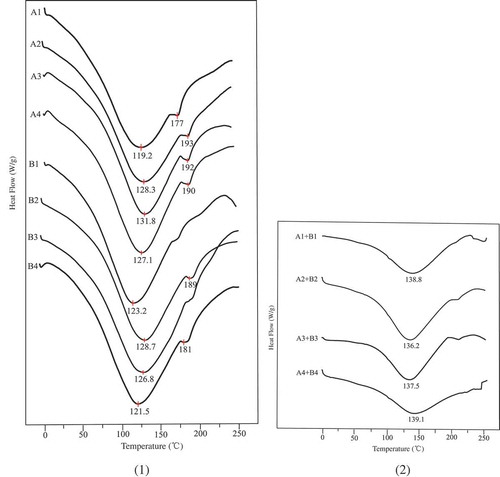
The endotherm above 150°C, which is associated with the melting of amylose double helices,[Citation40] is most pronounced in amylose samples in Fig. 5a A1–A4. Such endotherm in other samples in and those in is very weak, especially for those of blending groups, indicating that double helixes are not the indispensable components of retrograded starch. The Tp of all recrystallized sweet potato amylopectin is in the range of 123–129°C, which is in agreement with that of glutinous rice starch.[Citation40] Such results are different from those of other reference,[Citation41] in which the Tp is less than 80°C. The reason for this is that the amylopectin in the paper has a relatively narrow distribution of molecular weight, which leads to a more perfect recrystallization. Interestingly, the Tp of all recrystallized compound of sweet potato amylose and amylopectin is in the range of 136–140°C, higher than any single sample. The interaction of amylose and amylopectin during retrogradation in Ref. [Citation3] has been easily verified by using those amylose and amylopectin with narrow distribution of molecular weight in the paper. A surprising degree of regularity for the melting enthalpies (ΔH) of sweet potato amylose and amylopectin is observed in , the narrower the distribution, the higher the enthalpy. Only the fourth retrograded amylopectin is an apparent exception, which may be caused by crystalline region with less strength.[Citation42] In common with Tp, as shown in , the blending of sweet potato amylose and amylopectin during retrogradation results in the higher ΔH. The most exciting finding is that ΔH of amylose-2 + amylopectin-2 group gets the highest one, indicating the highest level of crystallinity. Such results skillfully explain why the duel-retrograded starch is more resistant to amylase hydrolysis,[Citation12] crystals of retrograded starch with a high level of crystallinity exist in the sample. Because there are amorphous regions in crystals of retrograded starch, the crystallinity from Jade software could not represent the ordered structure of such samples accurately.
The possible crystal structure of retrograded sweet potato starch
A schematic diagram in a and 6b exhibits the possible way of interaction between amylose and amylopectin during retrogradation. First, the sweet potato amylose and amylopectin with a single chain from granule are dissolved in water by unwinding. As shown in a, sweet potato amylopectin (black line) extends up to a tree-branch shape, and some of their branches with shorter side chains (red line) from different chains combine together by hydrogen bonds. A series of those parallel bonds produces the layered structure of retrograded starch, and the height of the layer should be determined by inclination of those bonds. Such inclination, however, is controlled by enthalpy of starch solutions. In other words, the higher the solutions temperature, the higher the layer structure. Sweet potato amylose (blue line) roves around amylopectin with the help of water. As the temperature of the solution of sweet potato amylose and amylopectin becomes low, more chains from amylose or amylopectin arrange in certain orders by hydrogen bonds as shown in b. The results of X-ray diffraction in b indicate that there are about five kinds of planes in retrograded sweet potato starch with d-spacing being 0.602, 0.428, 0.365, 0.336, and 0.298 nm, respectively. The d-spacing of 0.336 nm with the highest intensity of X-ray diffraction should generate between two adjacent amylopectins, which construct the basic framework of retrograded starch crystal. The d-spacing of 0.602 nm, formed by amylose, is probably the distance between two branched chains of amylose, in the same way as that of d-spacing of 0.336 nm, from amylopectin. Other two planes might originate from hydrogen bonds formed by both amylose and amylopectin and the conditions to control those planes should be further studied. The d-spacing of 0.336 nm and 0.602 nm could be enlarged by selecting longer branching chain of amylopectin or amylose with long distance between two adjacent branching chains, thus the crystals will become the ideal material instead of graphene[Citation14] as potential anodes in lithium-ion rechargeable batteries. Why these planes only generate between the given adjacent branching chains will be the greatest interest for us, and uncovering the secret will allow us to take a big step forward to control starch retrogradation effectively.
Conclusion
The blending of sweet potato amylose and amylopectin during retrogradation results in the higher ΔH. More ordered arrangements exist in retrograded starch of sweet potato amylose and amylopectin. The crystal of retrograded sweet potato starch with sharp X-ray diffraction could be prepared by blending sweet potato amylose and amylopectin with narrow distribution of molecular weight. There are five planes in retrograded sweet potato starch. The reticular distance between crystal planes corresponding to pronounced peak at 2θ = 26.5° is very close to that of graphite, suggesting why retrograded starch could substitute for graphite in Li-ion battery in our experiments at present. The other reticular distances between crystal planes, such as 0.602, 0.428, 0.365, and 0.298 nm, might absorb other ions such as K+, indicating that retrograded starch is a potential cathode of ion battery.
Conflict of interest
The authors have declared no conflict of interest.
Acknowledgments
Authors would like to thank Zhao Xiaoshuang and Wang Zhenhai for their help in experiments. We also thank professor Zhou Zhen in Nan Kai University for his help in battery experiment.
Additional information
Funding
References
- Ashwar, B. A.; Gani, A.; Wani, I. A.; Shah, A.; Masoodi, F. A.; Saxena, D. C. Production of Resistant Starch from Rice by Dual Autoclaving-Retrogradation Treatment: Invitro Digestibility, Thermal and Structural Characterization. Food Hydrocolloids 2016, 56, 108–117. doi:10.1016/j.foodhyd.2015.12.004.
- Li, W.; Tian, X.; Wang, P.; Saleh, A. S. M.; Luo, Q.; Zheng, J.; Ouyang, S.; Zhang, G. Recrystallization Characteristics of High Hydrostatic Pressure Gelatinized Normal and Waxy Corn Starch. International Journal of Biological Macromolecules 2016, 83, 171–177.
- Zhou, X.; Wang, R.; Yoo, S. H.; Lim, S. T. Water Effect on the Interaction between Amylose and Amylopectin during Retrogradation. Carbohydrate Polymers 2011, 86, 1671–1674.
- Witt, T.; Doutch, J.; Gilbert, E. P.; Gilbert, R. G. Relations between Molecular, Crystalline, and Lamellar Structures of Amylopectin. Biomacromolecules 2012, 13, 4273–4282.
- German, M. L.; Blumenfeld, A. L.; Guenin, Y. V.; Yuryev, V. P.; Toistoguzov, V. B. Structure Formation in Systems Containing Amylose, Amylopectin, and Their Mixtures. Carbohydrate Polymers 1992, 18, 27–34.
- Gudmundsson, M.; Eliasson, A.-C. Retrogradation of Amylopectin and the Effects of Amylose and Added Surfactants/Emulsifiers. Carbohydrate Polymers 1990, 13, 295–315.
- Jeffrey, D. K.; Donald, B. T. Amylose and Amylopectin Interact in Retrogradation of Dispersed High-Amylose Starches. Cereal Chemistry 1999, 76, 282–291.
- Åsa, R. W.; Mats, S.; Paul, G. Crystallinity and Morphology in Films of Starch, Amylose and Amylopectin Blends. Biomacromolecules 2002, 3, 84–91.
- Beate, P.;. Influence of Chain Length of Short Monodisperse Amyloses on the Formation of A-And B-Type X-Ray Diffraction Patterns. International Journal of Biological Macromolecules 1987, 9, 105–108.
- Klaochanpong, N.; Puttanlek, C.; Rungsardthong, V.; Puncha-Arnon, S.; Uttapap, D. Physicochemical and Structural Properties of Debranched Waxy Rice, Waxy Corn and Waxy Potato Starches. Food Hydrocolloids 2015, 45, 218–226. doi:10.1016/j.foodhyd.2014.11.010.
- You, S. Y.; Lim, S. T.; Lee, J. H.; Chung, H. J. Impact of Molecular and Crystalline Structures on in Vitro Digestibility of Waxy Rice Starches. Carbohydrate Polymers 2014, 112, 729–735.
- Hu, X.; Xie, Y.; Jin, Z.; Xu, X.; Chen, H. Effect of Single-, Dual-, and Triple-Retrogradation Treatments on in Vitro Digestibility and Structural Characteristics of Waxy Wheat Starch. Food Chemistry 2014, 157, 373–379.
- Lian, X.; Dong, S.; Gao, K.; Kang, H.; Li, L. Sweet Potato Amylose and Amylopectin with Narrower Distribution of Molar Mass and Chain Length Obtained by a Repeated Retrogradation–Hydrolysis Procedure. Journal of Applied Polymer Science 2016, 133, 8311–8319.
- Lian, X.; Wen, Y.; Zhu, W.; Li, L.; Zhang, K.; Wang, W. Retrograded Starches as Potential Anodes in Lithium-Ion Rechargeable Batteries. International Journal of Biological Macromolecules 2012, 51, 632–634.
- Schoch, T. J.;. Fractionation of Starch by Selective Precipitation with Butanol. Journal of the American Chemical Society 1942, 64, 2957–2961.
- Thory, R.; Sandhu, K. S. A Comparison of Mango Kernel Starch with A Novel Starch from Litchi (Litchi Chinensis) Kernel: Physicochemical, Morphological, Pasting, and Rheological Properties. International Journal of Food Properties 2017, 20, 911–921.
- Keatkrai, J.; Lumdubwong, N.; Chaiseri, S.; Jirapakkul, W. Characteristics of Menthone Encapsulated Complex by Mungbean, Tapioca, and Rice starches[J]. International Journal of Food Properties 2017, 20, 810–820.
- Liu, H.; Chaudhary, D.; Yusa, S.; Tadé, M. O. Glycerol/starch/Na+-montmorillonite Nanocomposites: A XRD, FTIR, DSC and 1H NMR Study. Carbohydrate Polymers 2011, 83, 1591–1597.
- Jane, J. L.; Robyt, J. F.; Huang, D. H. 13C-NMR Study of the Conformation of Helical Complexes of Amylodextrin and of Amylose in Solution. Carbohydrate Research 1985, 140, 21–35.
- Teleman, A.; Kruus, K.; Ämmälahti, E.; Buchert, J.; Nurmi, K. Structure of Dicarboxyl Malto-Oligomers Isolated from Hypochlorite-Oxidised Potato Starch Studied by 1 H and 13 C NMR Spectroscopy. Carbohydrate Research 1999, 315, 286–292.
- Gidley, M. J.; Bociek, S. M. Carbon-13 CP/MAS NMR Studies of Amylose Inclusion Complexes, Cyclodextrins, and the Amorphous Phase of Starch Granules: Relationships between Glycosidic Linkage Conformation and Solid-State Carbon-13 Chemical Shifts. Journal of the American Chemical Society 1988, 110, 3820–3829.
- Zhang, X.; Golding, J.; Burgar, I. Thermal Decomposition Chemistry of Starch Studied by 13 C High-Resolution Solid-State NMR Spectroscopy. Polymer 2002, 43, 5791–5796.
- Gidley, M. J.; Bociek, S. M. Molecular Organization in Starches: A Carbon 13 CP/MAS NMR Study. Journal of the American Chemical Society 1985, 107, 7040–7044.
- Atichokudomchai, N.; Varavinit, S.; Chinachoti, P. A Study of Ordered Structure in Acid-Modified Tapioca Starch by 13 C CP/MAS Solid-State NMR. Carbohydrate Polymers 2004, 58, 383–389.
- Morgan, K. R.; Furneaux, R. H.; Larsen, N. G. Solid-State NMR Studies on the Structure of Starch Granules. Carbohydrate Research 1995, 276, 387–399.
- Bogracheva, T. Y.; Wang, Y. L.; Hedley, C. L. The Effect of Water Content on the Ordered/Disordered Structures in Starches. Biopolymers 2001, 58, 247–259.
- Tan, I.; Flanagan, B. M.; Halley, P. J.; Whittaker, A. K.; Gidley, M. J. A Method for Estimating the Nature and Relative Proportions of Amorphous, Single, and Double-Helical Components in Starch Granules by 13C CP/MAS NMR. Biomacromolecules 2007, 8, 885–891.
- Mutungi, C.; Passauer, L.; Onyango, C.; Jaros, D.; Rohm, H. Debranched Cassava Starch Crystallinity Determination by Raman Spectroscopy: Correlation of Features in Raman Spectra with X-Ray Diffraction and 13 C CP/MAS NMR Spectroscopy. Carbohydrate Polymers 2012, 87, 598–606.
- Morrison, W. R.; Tester, R. F.; Gidley, M. J.; Karkalas, J. Resistance to Acid Hydrolysis of Lipid-Complexed Amylose and Lipid-Free Amylose in Lintnerised Waxy and Non-Waxy Barley Starches. Carbohydrate Research 1993, 245, 289–302.
- Saravanan, R.; Mohanlal, S. K. X-Ray Investigations on the Defect Structure of KCl with Cd++ Impurities. Crystal Research and Technology 1995, 30, 55–62.
- Hizukuri, S.;. Relationship between the Distribution of the Chain Length of Amylopectin and the Crystalline Structure of Starch Granules. Carbohydrate Research 1985, 141, 295–306.
- Bai, X.; Dong, Z.; Wu, X.; Tong, J.; Zhou, J. Changes in Crystalline Structure of Microspheres of Corn Starch and Amylose under Isothermal and Temperature Cycling Treatments. Industrial Crops and Products 2013, 51, 220–223.
- Hasjim, J.; Jane, J. Production of Resistant Starch by Extrusion Cooking of Acid-Modified Normal-Maize Starch. Journal of Food Science 2009, 74, C556–C562.
- Hsein-Chih, H. W.; Sarko, A. The Double-Helical Molecular Structure of Crystalline B-Amylose. Carbohydrate Research 1978, 61, 7–25.
- Luckett, C. R.; Wang, Y. J. Effects of β-amylolysis on the Resistant Starch Formation of Debranched Corn Starches. Journal of Agricultural and Food Chemistry 2012, 60, 4751–4757.
- Zeng, J.; Hu, Y.; Gao, H.; Sun, J.; Ma, H. Fructooligosaccharides Impact on the Hydration and Retro-Gradation of Wheat Starch and Gel. International Journal of Food Properties 2016, 19, 2682–2692.
- Ambigaipalan, P.; Hoover, R.; Donner, E.; Liu, Q. Retrogradation Characteristics of Pulse Starches. Food Research International 2013, 54, 203–212.
- Situ, W.; Chen, L.; Wang, X.; Li, X. Resistant Starch Film-Coated Microparticles for an Oral Colon-Specific Polypeptide Delivery System and Its Release Behaviors. Journal of Agricultural and Food Chemistry 2014, 62, 3599–3609.
- Shamai, K.; Bianco-Peled, H.; Shimonic, E. Polymorphism of Resistant Starch Type III. Carbohydrate Polymers 2003, 54, 363–369.
- Lian, X.; Wang, C.; Zhang, K.; Li, L. The Retrogradation Properties of Glutinous Rice and Buckwheat Starches as Observed with FT-IR, 13C NMR and DSC. International Journal of Biological Macromolecules 2014, 64, 288–293.
- Silverio, J.; Fredriksson, H.; Andersson, R.; Eliasson, A.-C.; Åman, P. The Effect of Temperature Cycling on the Amylopectin Retrogradation of Starches with Different Amylopectin Unit-Chain Length Distribution. Carbohydrate Polymers 2000, 42, 175–184.
- Singh, J.; Singh, N. Studies on the Morphological, Thermal and Rheological Properties of Starch Separated from Some Indian Potato Cultivars. Food Chemistry 2001, 75, 67–77.