ABSTRACT
In this study, changes in the color value, 5-hydroxymethylfurfural (5-HMF), free amino acid (FAA), reducing sugar, peroxide value (PV) and malondialdehyde (MDA) in vacuum-fried Pleurotus eryngii products were investigated. The FAA and reducing sugar decreased, whereas 5-HMF, PV and MDA increased during storage. The brown pigment from vacuum-fried P. eryngii was purified, characterized and identified by HPLC-MS/MS and was found to have a UV-visible maximum absorption at 209 nm. The Fourier-transform infrared spectra spectrum showed that there were long-chain compounds as well as compounds with functional groups of –OH, C=O, –NH2 and high conjugated C=C in the brown pigment. The brown pigment was also found to contain at least five compounds whose molecular weights were 562, 600, 554, 582 and 642, respectively. Among these compounds, there were long-chain fatty acid esters and derivatives produced by lipid oxidation. The presence of amides, aldehydes, ketones and carboxylic acids would suggest that the Maillard reaction was involved in the browning of vacuum-fried P. eryngii during storage.
Introduction
Pleurotus eryngii, or king oyster mushroom, is the third largest cultivated mushroom in the world.[Citation1] It has been successfully accepted by consumers for its flavor and high nutritional values.[Citation2,Citation3] The king oyster mushroom is a good source of carbohydrates, proteins and a variety of amino acids.[Citation4,Citation5] It also contains a number of biologically active compounds with therapeutic benefits including immune modulation as well as antioxidant, anti-inflammatory, antitumor and hypolipidemic activities.[Citation2,Citation6–Citation8] In addition, P. eryngii can be successfully cultivated in many agricultural and agro-industrial wastes simply and inexpensively.[Citation9] As a result, oyster mushrooms have become increasingly popular, with a current global production rate of approximately 3 × 105 tons/year in Asia alone.[Citation10]
Fresh mushrooms are highly perishable, mainly due to their high water content (approximately 89%), high respiration rate and the presence of microflora.[Citation11,Citation12] Thus, preservation technologies are necessary in order to extend the shelf life of these mushrooms. The preservation method used is mainly determined by the final use of the product and the estimated storage time. Drying technology plays an essential role in the manufacture of P. eryngii snacks because moisture content declines and shelf life increases after dehydration.[Citation13] Mushroom drying methods include hot air drying, vacuum drying, microwave drying, fluidized-bed drying, microwave–vacuum drying and freeze drying.[Citation13–Citation15] Among these methods, microwave drying is widely used in the drying of P. eryngii,[Citation16–Citation18] with a primary focus on the unit energy consumption, color, rehydration ratio and nutritional value of the dried P. eryngii products. In the present study, we studied the effects of storage on the quality of dried P. eryngii products in this study.
Vacuum frying is a technology widely used in the manufacture of dehydrated crispy mushrooms due to its advantages in maintaining color, flavor and nutritional qualities of raw material.[Citation19] However, the crispy P. eryngii snack produced by vacuum frying easily turns brown during storage. It has been reported that several techniques were applied to control the browning of fresh P. eryngii during storage including gamma irradiation, coating treatment, modified atmosphere packaging and cold storage.[Citation20–Citation22] Other methods used to control the browning of P. eryngii during processing include the use of antioxidants, inactivation of enzyme, reduction of the processing temperature and air isolation. However, little is known about the effects of storage on the browning of P. eryngii products. Thus, the aim of this study was to determine the effects of 2-month storage on the color value, 5-hydroxymethylfurfural (5-HMF), free amino acid (FAA), reducing sugar, peroxide value (PV) and malondialdehyde (MDA) in vacuum-fried P. eryngii slices. In addition, brown pigment from P. eryngii slices was extracted and characterized.
Materials and methods
Raw materials
Fresh P. eryngii mushrooms were collected from the Institute of Applied Mycology (Huazhong Agricultural University).
Sample preparation
P. eryngii were sorted, cleaned and washed under cold running water and then blanched in boiling water (96–98℃) for 2 min. Next the blanched mushrooms were cut into 5 mm slices and frozen at −25℃ before vacuum frying. Vacuum frying was carried out with vacuum frying equipment (VF-2, HaiRui, China). The working vacuum pressure was 9.6 kPa and the frying temperature was 90℃. After the vegetable oil reached the target temperature, the mushroom slices were placed into the frying basket, the lid was closed and the vessel was evacuated. When the vessel pressure reached vacuum, the frying basket was submerged into the hot oil. After 13 min, the lid of the vessel was opened and the mushroom slices were removed from the basket. The samples (moisture content 5.27% ± 0.90%) were cooled to room temperature and packaged for further analysis. The packaged P. eryngii slices were stored at room temperature for 60 days and sampled every 15 days for color measurement and chemical analysis.
Analysis of quality parameters
Color measurement
The color of P. eryngii items was measured using an UltraScan® XE meter (HunterLab, USA). The color values were expressed as L values (lightness) unit.
Chemical analysis
Mushroom samples were homogenized in distilled water using a Waring® disintegrator (Karl Kolb, Dreieich, Germany). The homogenate was then centrifuged at 5000 g for 10 min for collection of the supernatant. 5-HMF content was determined by a modified method.[Citation23] The 5-HMF in the supernatant was developed by reacting with 0.5% (w/v) thiobarbituric acid (TBA) after which absorbance (550 nm) of the solution was measured. Total FAA in the supernatant were determined by the ninhydrin colorimetric method.[Citation24] A dinitrosalicylic acid reagent was used to estimate the total reducing sugar in the supernatant,[Citation25] and glucose was used as a standard. Mushroom samples were ground and the resultant powder was extracted by petroleum ether. The extract was then used to determine the PV according to a reported method.[Citation26] TBA was used for the measurement of MDA content in all mushroom samples. [Citation27]
Extraction and purification of brown pigment
Mushroom samples were ground at a low temperature (4℃), and approximately 2.0 g of samples were placed in a 250 ml conical flask. Next 8% (w:w) of NaOH solution was added based on a solid–liquid ratio of 1:40 (w/v). The entire extraction was carried out at 60℃ for 80 min. When the preset extraction time was reached, the conical flask was removed from the water bath and the extract was filtered with a medium-grade paper filter. Then the filtrate pH was adjusted to 1–4 using 2 mol/l HCl solution in order to flocculate the brown pigment. The crude brown pigment was then centrifuged at 6000 rpm/min for 15 min and freeze-dried.
In order to characterize and identify the brown pigment, the crude brown pigment was further purified. The extracted crude pigment was re-dissolved in 8% of (w:w) NaOH solution, and pH was adjusted to 1–4 to flocculate the pigment. The precipitated pigment was collected after centrifuging. This method of alkali-solution and acid-isolation was repeated three times. Next, the pigment was freeze-dried and re-dissolved in 6 mo1/l HCl solution at 100℃ for 4 h in order to remove proteins, carbohydrates, lipids and other impurities. The hydrolyzate was filtered, the residue of pigment was washed three times using distilled water and freeze-dried for further study.
Ultraviolet-visible spectra (UV-VIS) analysis of brown pigment
The UV-VIS spectrum of the brown pigment sample was obtained in absolute ethanol using a spectrophotometer (UV-2550, Shimadzu, Japan) in the spectral range of 200–800 nm.
Fourier-transform infrared spectra (FT-IR) analysis of brown pigment
The brown pigment sample was mixed with spectroscopic-grade potassium bromide powder and then ground and pressed into pellets for FT-IR measurement. FT-IR spectra were recorded with a Nexus 470 FT-IR spectrometer (ThermoNicolet, USA) at the frequency range of 4000–400 cm−1.
HPLC-MS analysis of brown pigment
The purified pigment was dissolved in an ammonium hydroxide solution (pH 7.5) and then filtered with a syringe filter (Waters, Milford, MA, USA) for HPLC analysis. The separation and determination of the brown pigment was performed using a Waters e2695 reverse-phase HPLC system with a photodiode array detector and a Shim-pack VP-ODS C18 column (150 × 4.6 mm, i.d., 5 lm, Shimadzu). The mobile phase was ammonium hydroxide/acetonitrile (5:95, v:v, pH 7.5). The injection volume was 10 μl, and the flow rate was 0.4 ml/min. All samples were detected at 209 nm.
The identification of brown pigment was performed using an Agilent HPLC-MS system coupled with an ESI interface (Agilent Corporation, CA, USA) and a UV photodiode array detector. The mobile phases and gradient program were the same as those utilized for the HPLC analysis conditions. ESI-MS was detected by positive ion mode. The mass scale was recorded from 100 to 1000 m/z.
Statistical analysis
The data collected reflect the results of three repetitions. The results were expressed as mean values and standard error (SE). Analysis of variance was performed using a one-way analysis of variance, and differences between the means of samples were analyzed by Duncan’s test at a significance level of 0.05.
Results and discussion
Changes in quality attributes of vacuum-fried P. eryngii during storage
Color is one of the most important attributes of fried food since it affects overall quality.[Citation28] Higher L values indicate a lighter color, which means greater desirability for consumers. The color values (L values) of vacuum-fried mushrooms during storage are given in . The L value of the vacuum-fried mushroom was 85.61 ± 1.47, which was higher than the 58.42 reported by Chen et al.[Citation29] and the 78.93 reported by Chen et al.,[Citation17] but lower than the 86.92 reported by Chen et al.[Citation18] However, the L values of the mushroom samples decreased during storage (P < 0.05). Our results indicated that fried P. eryngii turned brown during storage. The decrease in L values representing a shift toward darker coloration may be attributed to non-enzymatic browning, including the Maillard reaction and lipid oxidation.
Table 1. Effects of storage on the quality attributes of vacuum-fried P. eryngii.
The 5-HMF, a classic product of the Maillard reaction, has previously been used as a browning marker and indicator of inadequate storage in various food products.[Citation30] As shown in , there is a significant difference in the content of 5-HMF in vacuum-fried P. eryngii during storage. The content of 5-HMF in the mushroom sample was 52.55 ± 2.46 μg/g, while its content increased to 82.92 ± 3.78 μg/g after 60 days of storage. The results showed that the levels of this furfural increased drastically during storage (P < 0.05). Our results also indicated that the increase of the 5-HMF in vacuum-fried mushrooms was consistent with the decrease of L values during storage. As shown in , the vacuum-fried P. eryngii had higher FAA levels than the 4.73 mg/g reported by Chen et al.,[Citation17] but lower than the 10.29 mg/g reported by Li et al.[Citation13] These differences could be due to varied drying technologies. Moreover, the contents of FAA and reducing sugar in vacuum-fried mushrooms decreased during storage (P < 0.05). Our results showed that both FAA and reducing sugar contents in the mushroom samples correlated with their L values during storage. Thus, we would suggest that the decrease of L values in vacuum-fried mushrooms could be due to the Maillard reaction.
The non-enzymatic browning reaction resulting from lipid oxidation has gained considerable attention recently.[Citation31] It has been reported that PV is a useful parameter that can be employed in the evaluation of lipid oxidation.[Citation32] The PV of vacuum-fried P. eryngii was found to increase during storage (P < 0.05), and the PV of the mushroom samples increased to 230.15 ± 6.52 mg/100 g after 60 days of storage (). MDA was reported to be the main product of membrane lipid oxidation.[Citation33] Throughout the 60 days of storage, MDA content increased in mushroom samples (P < 0.05). Lin et al.[Citation34] also observed that MDA content increased in CO2 treated and untreated button mushrooms, and they reported that CO2 treatment could alleviate oxidative injury during storage. These results would suggest that CO2 could inhibit the production of MDA in mushrooms during storage. Therefore, further studies on CO2 inhibition should be undertaken to validate this. Our results indicated that the increase in PV and MDA in vacuum-fried mushrooms was consistent with their corresponding decrease in L values during storage. Thus, the decrease in L values in vacuum-fried mushrooms could be due to lipid oxidation.
UV-VIS spectra assay of brown pigment
The UV-VIS absorption spectrum of purified brown pigment is shown in . The results showed that the brown pigment had a maximum absorption at 209 nm and the values of absorption decreased toward the visible region. The absorbed characteristic of the brown pigment was similar to the UV-VIS absorption spectra of the melanin pigment.[Citation35] In addition, a typical small absorption peak at 270–280 nm was observed. The small peak in this region is usually present in the spectra of natural melanin, which has been attributed to the proteins bound to the natural melanin.
Infrared spectra assay of brown pigment
Purified brown pigment was identified by the FT-IR spectrum. As shown in , the infrared spectra of the brown pigment displayed a broad and intense peak at around 3400 cm−1 for the characteristic absorption of hydroxyl groups (–OH).[Citation36] This result was probably due to the formation of hydrogen bond that made that peak wide and strong. The absorption peaks at 2925 and 2854 cm−1 were the resonance absorption peaks of –CH and –CH2, which were formed by the stretching vibration of C–H. The strong absorption peak at 1644 cm−1 specified the presence of C=O in the brown pigment molecule.[Citation37] The absorption peak at 1459 cm−1 was caused by the bending vibration of –CH3 and –CH2. The absorption peak at 1380 cm−1 was the characteristic methyl group peak. The appearance of the absorption peak at 721 cm−1 illustrated the presence of long-chain molecules that contain more than four CH2 groups. The bending vibration of C–CH3 caused the formation of peak at 1110 cm−1. Moreover, the conjugated system formed by C=O and C=C could result in the movement of absorption peaks toward a lower frequency. This movement was a possible explanation of the absorption peak at 1540 cm−1. The presence of C=O, high conjugated C=C and long-chain compounds revealed that lipid oxidation might explain the formation of brown pigment in vacuum-fried mushrooms during storage. It was notable that the absorption peaks in the range of 1600–1400 cm−1 could also be caused by the vibration of an aromatic ring. The weak absorption peaks in the range of 600–700 cm−1 indicated that the hydrogen atoms on the phenyl groups had been substituted and a conjugated system was formed. The FT-IR spectra of the brown pigment showed similarities in the main absorbance bands to previously studied melanin.[Citation38] It has been reported that synthesized melanin had signals around 1045 and 1220 cm−1, which corresponds to carbonyl, alcoholic or phenolic groups, respectively.[Citation39] However, the characteristic bands at 1075 cm−1 for phenols or carboxylic groups were not observed in the infrared spectra of the brown pigment as fungal melanin.[Citation40] Our results would thus suggest that the brown pigment isolated from vacuum-fried P. eryngii is a type of melanin-like pigment.
Identification of brown pigment
The purified brown pigment was analyzed by HPLC-MS. As shown in , the retention time of brown pigment is about 2.2 min. The standard mass spectrometry (MS1) of the brown pigment under the ESI positive scan mode is shown in . The brown pigment was found to be a mixture of compounds containing a variety of chemical components. The highest intensity of quasi-molecular ion peak [M + H]+ was obtained at m/z 562.9, which indicated that there was a compound with the molecular weight of 562 (named compound 1) in the pigment. Similarly, the second highest intensity of quasi-molecular ion peak [M + H]+ at m/z 600.8 showed that the molecular weight of another compound was 600 (named compound 2). The brown pigment also contained compounds whose molecular weights were 554 (named compound 3), 582 (named compound 4) and 642 (named compound 5), respectively. The second-order mass spectrometry (MS2) of the compounds in the brown pigment is shown in . A neutral loss of 74 was found in compound 1 (), which indicated that it contained a long-chain fatty acid methyl ester. The fragment ion peak at m/z 583.2 [M + H-17]+ () indicated the loss of –OH in compound 2. As shown in , the neutral loss of 44 in compound 3 could be caused by the loss of –CONH2, which would mean that there were amide groups in compound 3. Moreover, the neutral loss of 46 would suggest that compound 3 was an aromatic ester derivative. There was also a neutral loss of 44 (–CONH2) in compound 4 (). The fragment ion peak at 564.7 [M + H-18]+ indicated that compound 4 might contain –OH, –COOH or –CHO groups in which it would be easy to lose a molecule of water through the elimination reaction. As shown in , the loss of –OH from compound 5 produced the fragment ion peaks at m/z [M + H-17]+.
Figure 3. The HPLC (A) and standard mass spectrometry (MS1) (B) of brown pigment from vacuum-fried P. eryngii.
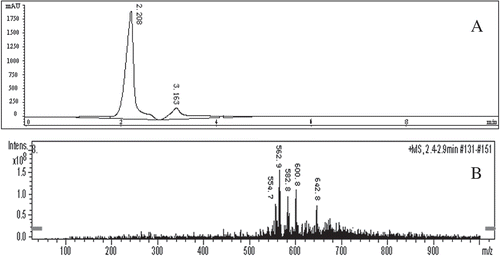
Figure 4. The secondary mass spectrogram (MS2) of compounds in brown pigment from vacuum-fried P. eryngii. (A) Compound 1 (m/z = 562.9), (B) compound 2 (m/z = 600.8), (C) compound 3 (m/z = 554.7), (D) compound 4 (m/z = 582.8) and (E) compound 5 (m/z = 642.8).
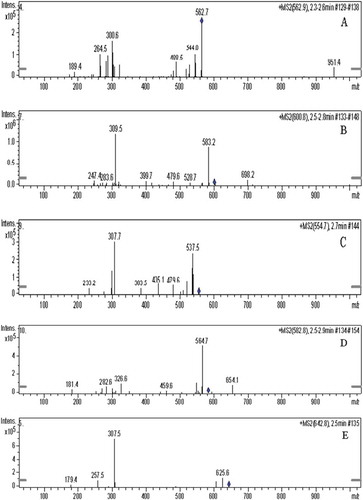
Overall the results of mass spectrometry would suggest that the brown pigment consisted of multiple chemical components, including long-chain fatty acid esters and derivatives produced by lipid oxidation. Peaks in the range of m/z 296–m/z 310 would represent for the oxidation products. The presence of amides, aldehydes, ketones and carboxylic acids in the brown pigment could be a result of the Maillard reaction during long-term storage. However, the data was inadequate for determining the molecular structure of these compounds. Further studies are needed to identify the molecular structure of brown pigment to clarify the browning mechanism of vacuum-fried P. eryngii.
Conclusion
In this study, the decrease of L values of vacuum-fried P. eryngii indicated that fried P. eryngii was browning during storage. We found that the brown pigment was a mixture that contained long-chain fatty acid esters, amides, aldehydes, ketones and carboxylic acids. Our results suggested that the browning of vacuum-fried P. eryngii might be caused by the Maillard reaction and lipid oxidation during storage. However, the complexity of chemical components in the brown pigment demonstrated that the browning of crispy P. eryngii during storage might also be due to multiple interactions. Therefore, the actual formation mechanism of brown pigment from vacuum-fried P. eryngii is envisaged in the future.
Conflict of interest
The authors declare no competing financial interest.
Additional information
Funding
References
- Mishra, K. K.; Pal, R. S.; Arunkumar, R.; Chandrashekara, C.; Jain, S. K.; Bhatt, J. C. Antioxidant Properties of Different Edible Mushroom Species and Increased Bioconversion Efficiency of Pleurotus Eryngii, Using Locally Available Casing Materials. Food Chem. 2013, 138, 1557–1563.
- Jeong, Y. T.; Jeong, S. C.; Gu, Y. A.; Islam, R.; Song, C. H. Antitumor and Immunomodulating Activities of Endo-Biopolymers Obtained from a Submerged Culture of Pleurotus Eryngii. Food Sci. Biotechnol. 2010, 19, 399–404.
- Ma, G.; Yang, W.; Mariga, A. M.; Fang, Y.; Ma, N.; Pei, F.; Hu, Q. Purification, Characterization and Antitumor Activity of Polysaccharides from Pleurotus Eryngii Residue. Carbohydr. Polym. 2014, 114, 297–305.
- Yildiz, S.; Yildiz, Ü. C.; Gezer, E. D.; Temiz, A. Some Lignocellulosic Wastes Used as Raw Material in Cultivation of the Pleurotus Ostreatus Culture Mushroom. Process Biochem. 2002, 38, 301–306.
- Zhang, A. Q.; Zhang, Y.; Yang, J. H.; Sun, P. L. Structural Elucidation of a Novel Heteropolysaccharide from the Fruiting Bodies of Pleurotus Eryngii. Carbohydr. Polym. 2013, 92, 2239–2244.
- Kalyoncu, F.; Oskay, M.; Sağlam, H.; Erdoğan, T. F.; Tamer, A. Ü. Antimicrobial and Antioxidant Activities of Mycelia of 10 Wild Mushroom Species. J. Med. Food. 2010, 13, 415–419.
- Choi, J. H.; Kim, H. G.; Jin, S. W.; Han, E. H.; Khanal, T.; Do, M. T.; Hwang, Y. P.; Choi, J. M.; Chun, S. S.; Chung, Y. C.; Jeong, T. C.; Jeong, T. C. Topical Application of Pleurotus Eryngii Extracts Inhibits 2, 4-Dinitrochlorobenzene-Induced Atopic Dermatitis in NC/Nga Mice by the Regulation of Th1/Th2 Balance. Food Chem. Toxicol. 2013, 53, 38–45.
- Chen, J.; Mao, D.; Yong, Y.; Li, J.; Wei, H.; Lu, L. Hepatoprotective and Hypolipidemic Effects of Water-Soluble Polysaccharidic Extract of Pleurotus Eryngii. Food Chem. 2012, 130, 687–694.
- Kirbag, S.; Akyuz, M. Effect of Various Agro-Residues on Growing Periods, Yield and Biological Efficiency of Pleurotus Eryngii. J. Food Agric. Environ. 2008, 6, 402–405.
- Im, C. H.; Park, Y. H.; Hammel, K. E.; Park, B.; Kwon, S. W.; Ryu, H.; Ryu, J. S. Construction of a Genetic Linkage Map and Analysis of Quantitative Trait Loci Associated with the Agronomically Important Traits of Pleurotus Eryngii. Fungal Genet. Biol. 2016, 92, 50–64.
- Villaescusa, R.; Gil, M. I. Quality Improvement of Pleurotus Mushrooms by Modified Atmosphere Packaging and Moisture Absorbers. Postharvest Biol. Technol. 2003, 28, 169–179.
- Reis, F. S.; Barros, L.; Martins, A.; Ferreira, I. C. Chemical Composition and Nutritional Value of the Most Widely Appreciated Cultivated Mushrooms: An Inter-Species Comparative Study. Food Chem. Toxicol. 2012, 50, 191–197.
- Li, X.; Feng, T.; Zhou, F.; Zhou, S.; Liu, Y.; Li, W.; Ye, R.; Yang, Y. Effects of Drying Methods on the Tasty Compounds of Pleurotus Eryngii. Food Chem. 2015, 166, 358–364.
- Walde, S. G.; Velu, V.; Jyothirmayi, T.; Math, R. G. Effects of Pretreatments and Drying Methods on Dehydration of Mushroom. J. Food Eng. 2006, 74, 108–115.
- Fernandes, Â.; Barros, L.; Barreira, J. C.; Antonio, A. L.; Oliveira, M. B. P.; Martins, A.; Ferreira, I. C. Effects of Different Processing Technologies on Chemical and Antioxidant Parameters of Macrolepiota Procera Wild Mushroom. LWT-Food Sci. Technol. 2013, 54, 493–499.
- Chen, J.; Lin, H.; Li, H.; Lin, Z.; Lin, Y. Modeling and Optimization of Microwave Vacuum Drying of Pleurotus Eryngii Slices. J. Vacuum Sci. Technol. 2013, 33, 1184–1190.
- Chen, J.; Lin, H.; Lin, Y.; Li, H.; Wang, Z. Optimized Technology of Pleurotus Eryngii by Microwave-Vacuum Drying Based on Quality and Energy Consumption. Trans. Chin. Soc. Agric. Eng. 2014, 30, 277–284.
- Chen, J.; Lin, H.; Li, H.; Lin, Y.; Wang, Z. Studies on Optimized Parameters of Combined Hot-Air and Microwave Vacuum Drying for Pleurotus Eryngii. J. Chin. Inst. Food Sci. Technol. 2014, 14, 131–140.
- Da Silva, P. F.; Moreira, R. G. Vacuum Frying of High-Quality Fruit and Vegetable-Based Snacks. LWT-Food Sci. Technol. 2008, 41, 1758–1767.
- Akram, K.; Ahn, J. J.; Yoon, S. R.; Kim, G. R.; Kwon, J. H. Quality Attributes of Pleurotus Eryngii following Gamma Irradiation. Postharvest Biol. Technol. 2012, 66, 42–47.
- Li, P.; Zhang, X.; Hu, H.; Sun, Y.; Wang, Y.; Zhao, Y. High Carbon Dioxide and Low Oxygen Storage Effects on Reactive Oxygen Species Metabolism in Pleurotus Eryngii. Postharvest Biol. Technol. 2013, 85, 141–146.
- Dama, C. L.; Kumar, S.; Mishra, B. K.; Shukla, K. B.; Mathur, S.; Doshi, A. Antioxidative Enzymatic Profile of Mushrooms Stored at Low Temperature. J Food Sci. Technol. 2010, 47, 650–655.
- Keeney, M.; Bassette, R. Detection of Intermediate Compounds in the Early Stages of Browning Reaction in Milk Products. J. Dairy Sci. 1959, 42, 945–960.
- Moore, S.; Stein, W. H. Photometric Ninhydrin Method for Use in the Chromatography of Amino Acids. J. Biol. Chem. 1948, 176, 367–388.
- Miller, G. L.;. Use of Dinitrosalicylic Acid Reagent for Determination of Reducing Sugar. Anal. Chem. 1959, 31, 426–428.
- Cebi, N.; Yilmaz, M. T.; Sagdic, O.; Yuce, H.; Yelboga, E. Prediction of Peroxide Value in Omega-3 Rich Microalgae Oil by ATR-FTIR Spectroscopy Combined with Chemometrics. Food Chem. 2017, 225, 188–196.
- Shamberger, R. J.; Shamberger, B. A.; Willis, C. E. Malonaldehyde Content of Food. J. Nutr. 1977, 107, 1404–1409.
- Dueik, V.; Robert, P.; Bouchon, P. Vacuum Frying Reduces Oil Uptake and Improves the Quality Parameters of Carrot Crisps. Food Chem. 2010, 119, 1143–1149.
- Chen, J.; Yang, Y.; Weng, M.; Lai, P.; Shen, H. Optimization of Combined Hot-Air and Vacuum Drying Technology for Instant Pleurotus Eeryngii. Trans. Chin. Soc. Agric. Eng. 2014, 30, 331–338.
- Serra-Cayuela, A.; Aguilera-Curiel, M. A.; Riu-Aumatell, M.; Buxaderas, S.; López-Tamames, E. Browning during Biological Aging and Commercial Storage of Cava Sparkling Wine and the Use of 5-HMF as a Quality Marker. Food Res. Int. 2013, 53, 226–231.
- Lu, F. S. H.; Bruheim, I.; Haugsgjerd, B. O.; Jacobsen, C. Effect of Temperature Towards Lipid Oxidation and Non-Enzymatic Browning Reactions in Krill Oil upon Storage. Food Chem.. 2014, 157, 398–407.
- Farhoosh, R.; Hoseini-Yazdi, S. Z. Shelf-Life Prediction of Olive Oils Using Empirical Models Developed at Low and High Temperatures. Food Chem. 2013, 141, 557–565.
- Gürbüz, G.; Heinonen, M. LC-MS Investigations on Interactions between Isolated β-lactoglobulin Peptides and Lipid Oxidation Product Malondialdehyde. Food Chem. 2015, 175, 300–305.
- Lin, Q.; Lu, Y.; Zhang, J.; Liu, W.; Guan, W.; Wang, Z. Effects of High CO2 In-Package Treatment on Flavor, Quality and Antioxidant Activity of Button Mushroom (Agaricus Bisporus) during Postharvest Storage. Postharvest Biol. Technol. 2017, 123, 112–118.
- Kimura, T.; Fukuda, W.; Sanada, T.; Imanaka, T. Characterization of Water-Soluble Dark-Brown Pigment from Antarctic Bacterium, Lysobacter Oligotrophicus. J. Biosci. Bioeng. 2015, 120, 58–61.
- Kanmani, P.; Yuvaraj, N.; Paari, K. A.; Pattukumar, V.; Arul, V. Production and Purification of a Novel Exopolysaccharide from Lactic Acid Bacterium Streptococcus Phocae PI80 and Its Functional Characteristics Activity in Vitro. Bioresour. Technol. 2011, 102, 4827–4833.
- Akyuz, S.; Akyuz, T.; Emre, G.; Gulec, A.; Basaran, S. Pigment Analyses of a Portrait and Paint Box of Turkish Artist Feyhaman Duran (1886-1970): The EDXRF, FT-IR and Micro Raman Spectroscopic Studies. Spectrochimica Acta . A: Mol. Biomol. Spectrosc. 2012, 89, 74–81.
- Zhang, J.; Cai, J.; Deng, Y.; Chen, Y.; Ren, G. Characterization of Melanin Produced by a Wild-Type Strain of Bacillus Cereus. Front. Biol. China. 2007, 2, 26–29.
- Drewnowska, J. M.; Zambrzycka, M.; Kalska-Szostko, B.; Fiedoruk, K.; Swiecicka, I. Melanin-Like Pigment Synthesis by Soil Bacillus Weihenstephanensis Isolates from Northeastern Poland. PLoS One. 2015, 10, e0125428.
- Sun, S.; Zhang, X.; Sun, S.; Zhang, L.; Shan, S.; Zhu, H. Production of Natural Melanin by Auricularia Auricula and Study on Its Molecular Structure. Food Chem. 2016, 190, 801–807.