ABSTRACT
Steady shear (η) and complex viscosities (η*) are important rheological properties of honeys. In this study, the effects of temperature and total soluble solids (TSS) concentration on these properties in 40 different Brazilian honeys were investigated. All honeys showed liquid-like behaviour at the temperatures and mechanical spectra tested and, except for the orange blossom and multi-southeast samples, respected the Cox-Merz rule. It was found that η varied between 147.3 Pa s and 0.35 Pa s, and η* between 151.33 Pa s and 0.42 Pa s, at 10°C and 60°C, respectively. Four experimental models (Arrhenius, Williams-Landel-Ferry (WLF), Vogel–Tamman–Fulcher (VTF), and power law) were used to evaluate the effect of temperature on η and η*. The Arrhenius model was the most appropriate for estimation of η of all honeys and η* of some. WLF was the most appropriate for predicting η* of the orange blossom, multi-southeast, and multi-southern honeys. Increase in temperature and decrease in TSS concentration lowered these values. The effect of TSS concentration on η and η* at different temperatures could be appropriately described by the power law and exponential models. Simplified models were proposed to determine the η and η* from the combined effect of both factors, which presented R2 of 0.9540 and 0.9334, and RMSE of 8.00 and 10.44, respectively. Thus, all models obtained in this study provide important tools towards the proper industrialisation of honeys and honey-based products.
Introduction
Honey is a complex semi-liquid food with high nutritional value. It is a natural sweetener whose composition includes a complex mixture of sugars, where the main constituents are fructose and glucose (approximately 60–85%), water (approximately 15–20%), amino acids, vitamins, minerals, enzymes, wax, pollen, organic acids, and pigments. It can be marketed in its pure form, mixed with propolis and plant extracts, or as a raw material for industrial use.[Citation1,Citation2]
The rheological behaviour of honey and its accurate prediction during all processing steps, such as transport in pipes, centrifugation, filtration, and heating, are of great importance in regard to the quality control of the final product and the reduction of economic losses during the process. The steady shear viscosity is a paramount quality parameter in honey processing and is influenced by its composition and, therefore, its floral origin, the bee species involved, climate conditions of the production area, harvesting methods, as well as processing and storage conditions.[Citation3,Citation4] As a result, many studies can be found in the literature which evaluated the viscosity of honey from different countries through steady state shear rheological measurements.[Citation1,Citation5–Citation14]
The small amplitude oscillatory shear (SAOS) test can be used in honey to adequately describe its rheology without considerable changes to its structure.[Citation7] The complex viscosity, measured through SAOS measurements, assesses the total resistance of the sample when subjected to a dynamic shear stress.[Citation15,Citation16] In mechanical spectra, the Newtonian nature of the material is confirmed if the complex viscosity is independent of the frequency.[Citation17] This behaviour is characteristic of liquid-like macromolecular solutions, such as honey.[Citation18] According to the Cox-Merz rule[Citation19], complex and steady shear viscosities, at equivalent frequency (rad.s−1) and shear rate values (s−1), present similar values in non-interactive molecular dispersions. This relationship was applied in Spanish honeys at temperatures of 40, 45, and 50°C[Citation12] but was not obtained in creamy honeys due to the presence of sugar crystals[Citation16], or in heather honeys, which form a dense gel-like fluid structure.[Citation17]
In addition to composition, temperature has a strong influence on the rheological properties of honeys. The Arrhenius model is widely used to evaluate the influence of temperature on the viscosity of honey, but others, including the Williams-Landel-Ferry (WLF), Vogel-Tamman-Fulcher (VTF), and power-law models, have also proven to be equally useful in evaluating this relationship.[Citation5,Citation8,Citation20,Citation21] Therefore, there is no consensus in the literature regarding which is the best model to be used. As a result, this decision will mainly depend on the temperature range analysed, the physico-chemical phenomena involved, and the extrapolation capacity of the model.
One of the most commonly used models is the WLF, which incorporates the glass transition temperature, and is suitable to describe the influence of temperature on the rheology of honeys, mainly within the temperature range of TG to TG + 100 K.[Citation22] Furthermore, this model can be used to predict the viscosity of the material at the glass transition temperature, a property that is extremely complex to measure experimentally due to the limitations of rheometers and viscometers.[Citation23] However, according to some authors, the best model to extrapolate temperature data for the determination of glass viscosity is the VTF.[Citation20,Citation24]
No studies have investigated the use of all these models in assessing the influence of temperature on the steady shear and complex viscosities of Brazilian honeys; it is therefore important to perform such an analysis to allow the proper prediction of these properties in different product processing steps, in relation to temperature changes. The combined effect of temperature and total soluble solids (TSS) concentration on honey plays an important practical role in the prediction of its steady shear viscosity[Citation12], given the ease of determining these variables. However, there is a gap in literature regarding the potentiality of complex viscosity prediction.
Therefore, the purpose of the study is: 1) to evaluate the application of the Cox-Merz rule for Brazilian honeys from different floral origins; 2) to evaluate the influence of temperature on the steady shear and complex viscosities of these honeys using the Arrhenius, WLF, VTF, and power law models; and 3) to assess the combined effect of temperature and TSS concentration of these honeys on their steady shear and complex viscosities.
Materials and methods
Honey samples
This study analysed 22 samples of monofloral honey (assa-peixe (Vernonia polysphaera)[Citation3], cipó-uva (Serjania lethalis)[Citation5], eucalyptus (Eucalyptus spp.)[Citation7], and orange blossom (Citrus sinensis)[Citation7]) and 18 samples of multifloral honey from the southeast[Citation8], south[Citation3], northeast[Citation3], and centrewest[Citation4] regions of Brazil, which were obtained from beekeepers and supermarkets. The honey was collected in the period between 2014 and 2015, and the floral source of each honey was provided by the apiarist.
Total soluble solids concentration analysis
Refractive indices of honey samples were measured using a refractometer (Reichert technologies, NY, USA) at 20°C to calculate the TSS concentration (°BRIX), based on Chataway tables.[Citation25]
Rheological measurements
Both small amplitude oscillation stress (SAOS) and steady shear rheological measurements were performed using a RheoStress 6000 rheometer (ThermoHaake, Karlsruhe, Germany) with a 1-mm-gap parallel plate sensor (35-mm diameter). The rheometer is equipped with a Peltier plate at the bottom that controls the temperature within the range of –40 to 200°C with an accuracy of 0.01°C. Additionally, to minimise vertical and horizontal temperature gradients in the sample and temperature fluctuations during a test, an active Peltier-controlled hood was used as a cover, which controls the temperature within the hood to match that of the bottom Peltier plate. The instrument was programmed for a set temperature and equilibrated for 10 min.
For steady shear rheological measurements, the samples were first warmed to 55°C to dissolve any crystals and kept in flasks at 30°C for 48 h to remove air bubbles that could interfere with rheological studies.[Citation26] Steady shear rheology of each sample was investigated in the shear rate range of 0.1–100 s–1 in 2 min by three cycles (upward, downward, and upward again) at 10, 15, 20, 25, 30 40, 50, and 60°C. Data referring to the temperatures ranging between 10 and 30°C were published in another study regarding the rheological characterisation of Brazilian honeys from different floral regions[Citation14], while data for temperatures ranging between 40 and 60°C are exclusive to this study.
The Newtonian model (Eq.1) was derived from shear stress and shear rate. Steady shear viscosity was utilised to determine the flow properties of honey.
where τ is shear stress (Pa), is shear rate (s−1) and η is steady shear viscosity (Pa s). The linear viscoelastic region for SAOS tests was pre-determined by stress sweep tests for each honey sample at 1 Hz. Oscillatory tests to obtain the complex viscosity were performed at frequencies (ω) comprised between 0.1 and 10 Hz at 10, 15, 20, 25, 30, 40, 50, and 60°C. [Citation7] The 1 Pa stress was in the linear viscoelastic region for all the temperatures tested. All rheological measurements were conducted in duplicate, and the average values are reported here.
Differential scanning calorimetry (DSC)
A differential scanning calorimeter DSC-60A (Shimadzu, Japan) was used for thermal scanning of honey samples. The DSC was attached to a refrigerated cooling system that controlled and monitored the temperature efficiently up to –90°C. Samples (7–10 mg) were weighed accurately in polymer coated aluminium pans, hermetically sealed, and allowed to equilibrate at the initial temperature for 10 min. A sealed empty aluminium pan was used as a reference. Runs were conducted from –65 to 230°C. The thermal scans were carried out from –90 to 250°C at a scanning rate of 5°C/min to obtain the complete thermal behaviour of pure honeys from low to high temperatures.[Citation7] The mid-point glass transition temperature, defined by the ASTM Standard (E 1356–03)[Citation27], was considered in this study.
Mathematical models
Cox-Merz rule
Two different models were used to study the relationship between complex (η*) and steady shear (η) viscosities. In the first model (Eq. 2), the data sets of η* vs. η are parallel to the frequency (ω) and shear rate (, respectively, and could be adjusted with the vertical shift factor α.[Citation12,Citation19]
The second model (Eq. 3) used the power type relationship to relate η* and η.[Citation12,Citation28]
where κ and β are constants of the model determined by nonlinear regression of the experimental data.
Temperature dependence of steady shear and complex viscosities
Four different models were used to study the temperature dependence of the rheological parameters η* and η: Williams–Landel–Ferry (WLF), Vogel–Taumman–Fulcher (VTF), Arrhenius, and power law.
WLF model (Eq. 4):
where η is the steady shear viscosity (Pa s), ηG the glass transition viscosity (Pa s), T the absolute temperature (K), TG the glass transition temperature (K,), and C1, C2 are constants. The fitting of experimental data by the WLF model was performed by nonlinear regression analysis in different forms [Citation20]:
Fixing C1 and C2 as universal constants (C1 = −40.16 e; C2 = 51.6 K. This is the practice in polymer research[Citation23] (WLF1);
Fixing C1 and C2 as universal constants (C1 = −40.16 e; C2 = 51.6 K) and considering the TG obtained experimentally (WLF2);
Utilising TG obtained experimentally and ηG equal to 1011 Pa s (WLF3);
Utilising TG obtained experimentally (WLF4).
The model whose data fit best to the relationship between viscosity and temperature was also used to evaluate the relationship between the complex viscosity and temperature.
VTF model (Eq. 5):
where η is the steady shear viscosity (Pa s), η∞ the steady shear viscosity (Pa s) at T = ∞ and T0 (absolute temperature at which the relaxation time relevant to molecular displacements becomes infinite), and B the phenomenological coefficient. In some studies, the value of T0 is fixed at 184 K, as estimated for sugar solutions in aqueous medium with similar TSS concentrations.[Citation29] In others, the value used for T0 is equal to TG.[Citation8,Citation24] Fitting of experimental data by the VTF model was performed by nonlinear regression analysis in different forms:
Utilising T0 equal to 184 K (VTF1);
Utilising T0 equal to TG (VTF2).
The model whose data fit best to the relationship between viscosity and temperature was also used to evaluate the relationship between the complex viscosity and temperature.
Arrhenius model (Eq. 6):
where η is the steady shear viscosity or complex viscosity (Pa s), η0 the material constant (Pa s), Eat,η the flow activation energy (J/mol), R the gas constant (J/mol.K), and T the absolute temperature (K).
Power law model (Eq. 7):
where η is the steady shear viscosity or complex viscosity (Pa s), T the absolute temperature (K), TG the glass transition temperature (K), and k and m are constants.
Effect of total soluble solids concentration
The steady shear viscosity, complex viscosity, and some model constants (TG, B, Eat,η and m) were evaluated using power law (Eq. 8) and exponential models (Eq. 9).
where y is the variable model response (steady shear viscosity (Pa s), complex viscosity (Pa s), TG concerning the WLF1 model, B concerning the VTF1 model, Eat,η concerning the Arrhenius model, or m concerning the power law model).
Combined effect of temperature and total soluble solids concentration
The following models (Eqs. 10 and 11) were investigated to evaluate the combined effect of temperature and TSS concentration on steady shear and complex viscosities:
where C is the TSS concentration in °BRIX; T is the temperature (K); and η0, D1, and D2 are parameters determined by nonlinear regression analysis.
Statistical analysis
The data corresponding to TSS concentration was analysed by one-factor analysis of variance (ANOVA). Multiple comparisons were performed using the Tukey’s multiple-range test and statistical significance was set at α = 0.05. It should be mentioned that a nonlinear regression technique, which uses the Levenberg-Marquardt method to solve nonlinear regression, was used. The performance of derived models was evaluated using the statistical parameter root mean square error (RMSE – Eq. 12), in addition to R2. These parameters can be calculated as follows:
where yexp,i is the experimental data, ypre,i is the predicted data obtained by each nonlinear model, and N is the number of data points. This statistical analysis was performed using version 9.1 of the Statistical Analysis System (SAS) software.
Results and discussion
shows the results obtained for the TSS concentrations of the honeys. The multifloral-centrewest type presented a TSS content significantly lower (p < 0.005) than the assa-peixe, cipó uva, orange blossom, and multifloral-southeast types. The range of TSS values found in this study, 80.89 to 83.57°BRIX, is higher than that found in Mexican honeys, 77.0 to 80.67% [Citation30], honeys of India, 79.16 to 80.03% [Citation31], and those produced in Rio Grande do Norte, Brazil, 70 to 81%.[Citation32] However, our results resemble the values obtained in honeys from Spain, 80.4 to 82.0%[Citation12], Romania, 79.8 to 81.94%[Citation8,Citation33], and Israel, 80.5 to 83.2%.[Citation34] Anomalous TSS values may indicate product adulteration[Citation35], which was not observed in this study.
Table 1. TSS concentration (°BRIX) of selected Brazilian honeys.
shows the dynamic rheological behaviour of Brazilian honeys within a wide temperature range. The higher the η*, the higher the total resistance of the sample when subjected to a dynamic shear stress. The value of η* would potentially be an adequate indicator to detect adulteration at levels ranging between 10% and 50% of saccharose and fructose syrup, because the resistance to deformation decreases with adulterant addition.[Citation36] The η* is independent of frequency, indicating a liquid-like behaviour of the honeys, reported by many authors in the literature.[Citation11,Citation12,Citation18,Citation37] In contrast, the η* values of creamed honey and honey mixed with propolis decrease with increasing frequency.[Citation2,Citation16] The increase in temperature results in an increased kinetic energy of the honeys, causing a decrease in intermolecular forces and the consequent reduction of η*.[Citation20] The honey cipó uva ( – b) presented, at 10 Hz, a variation of its η* from 151.33 Pa s to 0.67 Pa s at 10°C and 60°C, respectively. This honey showed the highest η* values, except at 60°C. At this temperature, the highest value of η* was 0.84 Pa s, referring to the honey assa peixe ( – a). On the other hand, the multifloral-centrewest honey ( – h) showed the lowest η* values, ranging from 53.72 at 10°C to 0.42 Pa s at 60°C. The variation among samples occurs because of the natural variation in honey compositions, especially in terms of moisture and sugar contents.[Citation38]
Figure 1. Effect of temperature on complex viscosity obtained from sweep test of selected Brazilian honeys: a) Assa Peixe; b) Cipó Uva; c) Eucalyptus; d) Orange Blosson; e) Multi-Southeast; f) Multi-South; g) Multi-Northeast; h) Multi-Centerwest
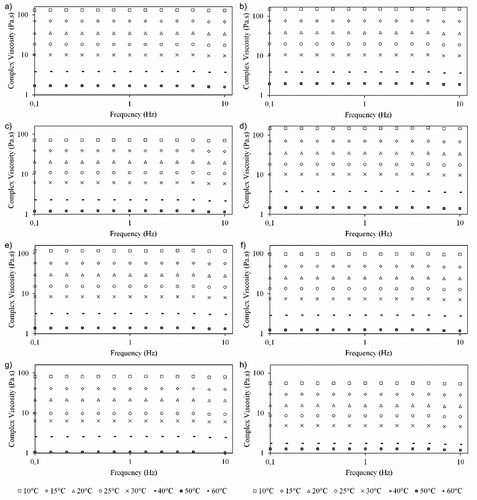
With regards to the steady shear viscosity (), results obtained at temperatures ranging from 10°C to 30°C have been reported in a previous study.[Citation14] Between 40°C and 60°C, the honeys presented an excellent fit to the Newton model, with R2 greater than 0.9995 and RMSE lower than 1.7120, which confirms the results obtained in other studies.[Citation8,Citation9,Citation11] Furthermore, just as η*, η decreases as the temperature increases for all honeys.
Cox-Merz rule
The relationship between η and η * can be evaluated using the Cox-Merz rule (Equations 2 and 3 – ), which allows comparing the values obtained from dynamic-oscillatory tests with shear testing at steady state.[Citation39] In the frequency range of 0.62 to 62.83 rad/s, and shear values comprised between 0.62 to 62.83 s−1 at temperatures ranging from 10°C to 60°C, all honeys evaluated, with the exception of orange blossom (H4) and multi-Southeast (H5), attended the Cox-Merz rule, presenting a shift factor (α) close to 1 (0.913 to 0.998), with R2 greater than 0.9944, and RMSE less than 2.0096. At temperatures below 30°C, Spanish honeys did not meet the rule, with η* values lower than η.[Citation12] However, as observed in the present study, when not meeting the Cox-Merz rule, the η* is typically greater than the η, and deviation occurs due to deterioration of the material structure as a result of the effect of the shear rate applied on the system.[Citation40]
Table 2. Shift factor (α) of the Cox-Merz Rule and k and β parameters of power type relationship of selected Brazilian honeys.
The η* and η data cited above also showed a good fit to the power type relationship (), with R2 greater than 0.9967 and RMSE lower than 1.3618. The κ parameter varied from 1.185 to 0.742 for the honeys assa peixe and multi-northeast, respectively. The parameter β varied from 0.970 to 1.082 for the eucalyptus and multi-northeast honeys, respectively. These variations were similar to those observed for Spanish honeys.[Citation12] However, heather honeys showed values significantly different to those obtained from this study, where κ varied from 0.017 to 0.264 and β varied from 1.39 to 2.11, and these values are characteristic of non-Newtonian fluids.[Citation17] There was not influence of soluble solids content on the parameters α, k, and β, corroborating the results obtained by other authors.[Citation12,Citation17]
Influence of temperature and total soluble solids concentration
The influence of temperature on viscosity was investigated using various models widely discussed in literature (). Regarding the WLF, adjustments of experimental data for the model were performed by nonlinear regression in four different ways.[Citation20] Among these methods of manipulating the WLF equation, the WLF1 model, where C1 (e) and C2 (K) are defined as universal constants equal to ˗40.16 e and 51.6 K, respectively, resulted in the best fit, since it showed the highest R2 (>0.9990) and lowest RMSE (<1.6238) values. In contrast, the WLF2 model, which uses the aforementioned universal constants and the experimental TG values, presented the worst fit with R2 greater than 0.9766 and RMSE lower than 7.0750.
Table 3. Parameters calculated of WLF, VTF, Arrhenius, and power law for Brazilian honey steady shear viscosity (η) and complex viscosity (η*).
In a comparative study of different methods for adjusting the WLF model to the experimental data of supersaturated carbohydrate system models, the results obtained were similar to the ones obtained in the present study with regards to WLF1 and WLF2 for honeys, in relation to both the adjust quality and the parameters predicted by the models. For solutions of fructose, fructose-glucose, and glucose, the WLF4, which uses the TG value obtained experimentally, showed the highest R2 values (>0.992).[Citation20]
Other authors employed the WLF1 model in their studies[Citation5,Citation41] and obtained results different from those observed in this study () for the predicted values of ηG, from 2.21 x 1011 to 2.62 x 1011 Pa s and 1.88 x 1011 to 2.86 x 1011 Pa s, respectively, and TG, from 223.83 to 228.44 K and 220.34 to 227.43 K, respectively. The TG values predicted in these studies are close to those obtained experimentally for the selected Brazilian honeys[Citation14] (). shows the discrepancy between the TG values obtained experimentally and the values obtained by the WLF1 model using the steady shear viscosity data and WLF1 using the complex viscosity data. Therefore, despite its fitting quality, the WLF1 model was not able to predict TG values similar to those obtained by DSC.
Figure 3. TG values obtained by different methods as a function of TSS concentration of selected Brazilian honeys
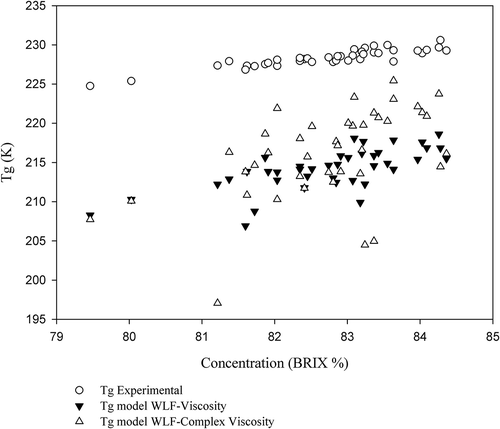
When fixing the glass viscosity at 1011 Pa s, despite the differences with experimental values of TG obtained for the honeys, as in the WLF3 model, it does not significantly lose its prediction quality.[Citation22] The WLF4 model was applied to evaluate the influence of temperature on viscosity of honeys from Romania[Citation8] and Australia.[Citation22] In both cases, WLF4 was considered the best model when compared to the Arrhenius, VTF, and power law models. Regarding the predicted values of the constants C1 and C2 (K), in this study, they were different from the values used in WLF1, considered universal constants, which indicates that they may vary in function of the material under study.[Citation23]
The VTF1 model, in which a T0 value of 184 K was used, showed higher quality of fit with the experimental data than the VTF2 model, where T0 was equal to TG. This contradicts the results obtained in a study focusing on the influence of temperature on the viscosity of Australian honeys at subzero temperatures. [Citation23] In this work, the authors concluded that the use of TG increases the predictive ability of the WLF and VTF models. Some authors opted for the use of VTF1[Citation12,Citation20] for their analysis, obtaining results that corroborate with those seen in this study. Others used VTF2[Citation8,Citation21], and obtained η∞ values around 10–4 Pa s, and B values ranging from 602.85 to 718.94 K and 617.0 to 757.1 K, respectively, which differ greatly from those obtained in this study (η∞ values around 10–2 Pa s and B values ranging from 467.88 to 481.70 K).
When comparing the regression parameters of all models for the steady shear viscosity (), the Arrhenius model stands out as that with the highest R2 (>0.9997) and lowest RMSE (<0.1736) values for all honeys. In contrast, for Argentinian honeys[Citation20], at temperatures ranging from −5°C to 67°C, the VTF model was the most appropriate, and for Spanish honeys [Citation21], for a temperature range between 7°C and 55°C, the power law model showed the best fit to the experimental data for most of the analysed honeys.
Based on the above, the model that best represents the effect of temperature on viscosity will depend on the temperature range studied, the extrapolation capacity of the model, and the physico-chemical properties of the honeys, that vary mainly according to floral origin, climatic conditions of the production area, harvesting methods, and processing and storage conditions. [Citation3,Citation4]
The activation energy (Eat,η) () ranged from 84.57 to 92.53 kJ/mol for the honeys cipó uva and multi-centrewest, respectively, similar to the values reported in the literature. [Citation8,Citation11,Citation12,Citation42,Citation43] The effect of TSS concentration on Eat,η may be described properly (p < 0.05) by means of the power law (Eq. 8) and exponential equations (Eq. 9) (). The increase in TSS concentration results in an exponential increase in activation energy, indicating that the viscosity is more sensitive to temperature changes at higher TSS concentrations, as demonstrated in another study.[Citation12]
Table 4. Effect of TSS concentration on the models parameters WLF1, VTF1, Arrhenius, and power law.
The influence of honey TSS concentration on the TG coefficient of the WLF1 model, on the B coefficient of the VTF1 model, and on the coefficient m in the power law model, was also appropriately described (p <0.05) through the power law (Eq. 8) and exponential equations (Eq. 9) (). With increasing TSS concentration, all parameters of the models evaluated increased exponentially. The behaviour observed for TG is indicated in the diagram of different states of a food as a function of the solids content and temperature.[Citation44] Understanding this behaviour is important when selecting the appropriate temperature and TSS conditions to be applied during processing and storage in order to allow greater stability of the food.[Citation44] The coefficient B is indicative of fluid fragility. The higher its value, the more resistant the material structure to thermal degradation.[Citation24] Thus, with increasing TSS concentration, honeys are considered less vulnerable to this type of degradation. The constant m, despite its increase with TSS concentration, has no physical significance.[Citation20]
The influence of temperature on the complex viscosity was evaluated using the WLF and VTF models, which showed the best fits for steady shear viscosity data, in addition to the Arrhenius and power law models (). Contrary to what was observed for the steady shear viscosity, the Arrhenius model only showed the best values, in terms of statistical parameters, for the honeys assa peixe, cipó uva, eucalyptus, multi-northeastern, and multi-centrewest, with R2 values greater than 0.9994 and RMSE values below 0.8476 (). For the honeys orange blossom, multi-southeast, and multi-southern, the WLF model presented R2 values greater than 0.9999 and low RMSE values (<0.4133) () and, therefore, in these cases, was more suitable for determining the complex viscosity in function of the temperature.
There were no proper adjustments of the coefficients of these models in relation to the TSS concentration (p >0.05). The Eat,η was 86.34 and 100.40 kJ/mol for the multi-centrewest and orange blossom honeys, respectively, which corroborates with the values obtained for honeys from Turkey. [Citation18] The TG predicted for the WLF model ranged from 210.44 to 220.70 K and also differed from the experimental values obtained by DSC (). The B values varied between 1361.68 and 1581.04 K for the eucalyptus and orange blossom honeys, respectively, and are in the range of values obtained by other authors regarding the steady shear viscosity data.[Citation12,Citation20] The m coefficient varied from 7.53 to 8.63 for the eucalyptus and orange blossom honeys, respectively, which were also in concordance with the values previously obtained for honeys and modelled supersaturated carbohydrate solutions, in terms of steady shear viscosity data.[Citation20]
The effect of TSS concentration on the steady shear and complex viscosities of selected Brazilian honeys at different temperatures may be adequately described by the power law (Eq. 8) and exponential models (Eq. 9) (), since most R2 and RMSE values were very similar for both models. These results are similar to those obtained during the evaluation of Spanish honeys within the temperature range of 25°C to 50°C. [Citation12] The increase in TSS concentration and consequent reduction in water content causes an increase in steady shear and complex viscosities values, due to the ability of water to dilute food systems. [Citation39]
Table 5. Effect of TSS concentration in the steady shear viscosity and complex viscosity.
Combined effect of temperature and total soluble solids concentration
Understanding the combined effect of temperature and TSS concentration presents an important practical application when it comes to determining the rheological properties of honeys in terms of steady shear and complex viscosities. For both properties, the model that presented the lowest RMSE (8.0020 and 10.4432, respectively) and highest R2 (0.9540 and 0.9517, respectively) was that described by equation 10. Therefore, the following equations (13 and 14) were proposed to evaluate η and η* of Brazilian honeys, respectively, within the range of concentrations (C) and temperatures (T) studied. For the evaluation of Spanish honeys, the same equation was defined as the best model for determining the steady shear viscosity[Citation12]; however, its R2 value was 0.8221, lower than the R2 obtained here (0.9540), and its coefficients (η1, D1 and Ea) varied greatly from those obtained in this study.
The activation energy of the steady shear viscosity prediction model was lower than that of the complex viscosity one, indicating that the latter is more sensitive to temperature changes. This demonstrates the increased sensitivity of the measurements made by means of dynamic oscillatory testing and the efficiency of the determination of physical and chemical changes of the materials. [Citation12]
Conclusion
Brazilian honeys presented liquid-like behaviour in the mechanical spectrum (0.1 to 10 Hz) evaluated at temperatures ranging between 10°C and 60°C. Regarding the η and η*, the shift factor α was close to 1 for most of the evaluated honeys, indicating compliance with the Cox-Merz rule. The WLF1, WLF3, WLF4, VTF1, Arrhenius, and power law models showed good fit, considering the statistical criteria evaluated (R2 and RMSE). The Arrhenius model was the best for steady shear viscosity data. Regarding the complex viscosity, the WLF1, VTF1, Arrhenius, and power law models also showed good fit, and the Arrhenius model was the most adequate for the assa peixe, cipó uva, eucalyptus, multi-northeastern and multi-centrewest honeys, while the WLF1 model gave the best outcomes for the orange blossom, multi-southeast, and multi-southern honeys. The WLF1 model could not predict a TG value similar to that obtained via the DSC. Regarding the effect of TSS concentration (ranging between 79.52°BRIX and 84.48°BRIX) on the rheological parameters, η and η* were properly modelled using the exponential and power law models. The equations defining the combined effect of temperature and TSS concentration are useful to ensure adequate processing and quality control of honeys and honey-based products.
Additional information
Funding
References
- Al-Mahasneh, M. A.; Rababah, T. M.; Amer, M.; Al-Omoush, M. Modeling Physical and Rheological Behavior of Minimally Processed Wild Flowers Honey. J. Food Process. Preservation 2014, 38, 21–30.
- Da Costa, C. C.; Pereira, R. G. The Influence of Propolis on the Rheological Behaviour of Pure Honey. Food Chem. 2002, 76, 417–421.
- Yanniotis, S.; Skaltsi, S.; Karaburnioti, S. Effect of Moisture Content on the Viscosity of Honey at Different Temperatures. J. Food Eng. 2006, 72, 372–377.
- Turhan, I.; Tetik, N.; Karhan, M.; Gurel, F.; Tavukcuoglu, H. R. Quality of Honeys Influenced by Thermal Treatment. LWT Food Sci. Technol. 2008, 41, 1396–1399.
- Juszczak, L.; Fortuna, T. Rheology of Selected Polish Honeys. J. Food Eng. 2006, 75, 43–49.
- Bhandari, B.; D’Arcy, B.; Chow, S. Rheology of Selected Australian Honeys. J. Food Eng. 1999, 41, 65–68.
- Ahmed, J.; Prabhu, S. T.; Raghavan, G. S. V.; Ngadi, M. Physico-Chemical, Rheological, Calorimetric and Dielectric Behavior of Selected Indian Honey. J. Food Eng. 2007, 79, 1207–1213.
- Oroian, M.;. Physicochemical and Rheological Properties of Romanian Honeys. Food Biophys. 2012, 7, 296–307.
- Dobre, I.; Georgescu, L. A.; Alexe, P.; Escuredo, O.; Seijo, M. C. Rheological Behavior of Different Honey Types from Romania. Food Res. Int. 2012, 49(1), 126–132.
- Gómez-Díaz, D.; Navaza, J. M.; Quintáns-Riveiro, L. C. Physicochemical Characterization of Galician Honeys. Int. J. Food Properties 2012, 15, 292–300.
- Nayik, G. A.; Dar, B. N.; Nanda, V. Physico-Chemical, Rheological and Sugar Profile of Different Honeys from Kashmir Valley of India. Arabian J. Chem. [Internet] 2015. http://www.sciencedirect.com/science/article/pii/S1878535215002579
- Oroian, M.; Amariei, S.; Escriche, I.; Gutt, G. Rheological Aspects of Spanish Honeys. Food Bioprocess Technol. 2013, 6, 228–241.
- Kayacier, A.; Karaman, S. Characteristics of Selected Turkish Honeys. J. Texture Stud. 2008, 39, 17–27.
- Silva, V. M. D.; De Carvalho, L. A.; De Oliveira, N. L.; Torres Filho, R. D. A.; De Resende, J. V. Rheological and Thermal Properties of Selected Brazilian Honeys from Various Floral Origins. J. Texture Stud. 2016, 47, 208–219.
- Yamul, D. K.; Lupano, C. E. Viscoelastic Properties of Whey Protein Concentrate Gels with Honey and Wheat Flour at Different Ph. J. Texture Stud. 2009, 40, 319–333.
- Karasu, S.; Toker, S. O.; Yilmaz, M. T.; Karaman, S.; Dertli, E. Thermal Loop Test to Determine Structural Changes and Thermal Stability of Creamed Honey : Rheological Characterization. J. Food Eng. 2015, 150(150), 90–98.
- Witczak, M.; Juszczak, L.; Gałkowska, D. Non-Newtonian Behaviour of Heather Honey. J. Food Eng. 2011, 104(4), 532–537.
- Kayacier, A.; Yüksel, F.; Karaman, S. Dynamic Mechanical Spectra of Selected Turkish Honeys: Construction of Predictive Models for Complex Viscosity Using Two Different Nonlinear Modeling Techniques. Int. J. Food Properties 2014, 17(June 2011), 93–110.
- Cox, W. P.; Merz, E. H. Correlation of Dynamic and Steady Flow Viscosities. J. Polymer Sci. 1958, 28(118), 619–622.
- Recondo, M. P.; Elizalde, B. E.; Buera, M. P. Modeling Temperature Dependence of Honey Viscosity and of Related Supersaturated Model Carbohydrate Systems. J. Food Eng. 2006, 77, 126–134.
- Gómez-Díaz, D.; Navaza, J. M.; Quintáns-Riveiro, L. C. Effect of Temperature on the Viscosity of Honey. Int. J. Food Properties 2009, 12(April 2007), 396–404.
- Sopade, P. A.; Halley, P.; Bhandari, B.; D’Arcy, B.; Doebler, C.; Caffin, N. Application of the Williams – Landel – Ferry Model to the Viscosity – Temperature Relationship of Australian Honeys. J. Food Eng. 2002, 56, 67–75.
- Sopade, P. A.; Halley, P. J. Dynamic and Steady-State Rheology of Australian Honeys at Subzero Temperatures. J. Food Process Eng. 2004, 27, 284–309.
- Angell, C.; Bressel, R.; Green, J.; Kanno, H.; Oguni, M.; Sare, E. Liquid Fragility and the Glass-Transition in Water and Aqueous-Solutions. J. Food Eng. 1994, 22, 115–142.
- AOAC. Official Methods of Analysis 16th ed.; Association of Official Analytical Chemists: Arlington, 1995.
- Mossel, B.; Bhandari, B.; D’Arcy, B.; Caffin, N. Use of an Arrhenius Model to Predict Rheological Behaviour in Some Australian Honeys. LWT Food Sci. Technol. 2000, 33, 545–552.
- ASTM. Standard Test Method for Glass Transition Temperatures by Differential Scanning Calorimetry or Differential Thermal Analysis; Annual Book of ASTM Standards. West Conshohocken, United States; E 1356 – 03, 2003.
- Bistany, K. L.; Kokini, J. L. Comparison of Steady Shear Rheological Properties and Small Amplitude Dynamic Viscoelastic Properties of Fluid Food Materials. J. Texture Stud. 1983, 14, 113–124.
- Parker, R.; Ring, S. G. A Theoretical Analysis of Diffusion Controlled Reactions in Frozen Solutions. Cryo-Letters 1995, 16, 197–208.
- Viuda-Martos, M.; Ruiz-Navajas, Y.; Zaldivar-Cruz, J. M.; Kuri, V.; Fernández-López, J.; Carbonell-Barrachina, Á. A.;, et al. Aroma Profile and Physico-Chemical Properties of Artisanal Honey from Tabasco, Mexico. Int. J. Food Sci. Technol. 2010, 45, 1111–1118.
- Nayik, G. A.; Nanda, V. Physico-Chemical, Enzymatic, Mineral and Colour Characterization of Three Different Varieties of Honeys from Kashmir Valley of India with a Multivariate Approach. Polish J. Food Nutr. Sci. 2015, 65(2), 101–108.
- Costa, P. A.; Moraes, I. C. F.; Sobral, J. A.; Gomide, C. A.; Carrer, C. C. Physical Properties of Honeys Produced in the Northeast of Brazil. Int. J. Food Stud. 2013, 2(April), 118–125.
- Oroian, M.;. Measurement, Prediction and Correlation of Density, Viscosity, Surface Tension and Ultrasonic Velocity of Different Honey Types at Different Temperatures. J. Food Eng. 2013, 119(1), 167–172.
- Cohen, I.; Weihs, D. Rheology and Microrheology of Natural and Reduced-Calorie Israeli Honeys as a Model for High-Viscosity Newtonian Liquids. J. Food Eng. 2010, 100(2), 366–371.
- Terrab, A.; Recamales, A. F.; Hernanz, D.; Heredia, F. J. Characterisation of Spanish Thyme Honeys by Their Physicochemical Characteristics and Mineral Contents. Food Chem. 2004, 88, 537–542.
- Tahsin, M.; Berna, N.; Said, O.; Karaman, S.; Dertli, E.; Sagdic, O.;, et al. Steady, Dynamic and Creep Rheological Analysis as a Novel Approach to Detect Honey Adulteration by Fructose and Saccharose Syrups : Correlations with HPLC-RID Results. Food Res. Int. 2014, 64, 634–646.
- Yoo, B.;. Effect of Temperature on Dynamic Rheology of Korean Honeys. J. Food Eng. 2004, 65, 459–463.
- Lazaridou, A.; Biliaderis, C. G.; Bacandritsos, N.; Sabatini, A. G. Composition, Thermal and Rheological Behaviour of Selected Greek Honeys. J. Food Eng. 2004, 64, 9–21.
- Steffe, J. F.;. Rheological Methods in Food Process Engineering 2° ed.; Freeman Press: East Lansing, USA, 1996.
- Chamberlain, E. K.; Rao, M. A. Rheological Properties of Acid Converted Waxy Maize Starches in Water and 90% DMSO/10% Water. Carbohydr. Polym. 1999, 40, 251–260.
- Al-Malah, K. I. M.; Abu-Jdayil, B.; Zaitoun, S.; Ghzawi, A. A.-M. Application of WLF and Arrhenius Kinetics to Rheology of Selected Dark-Colored Honey. J. Food Process Eng. 2001, 24, 341–357.
- Khanlari, G. R.; Heidari, M.; Momeni, A. A.; Abdilor, Y. Prediction of Shear Strength Parameters of Soils Using Artificial Neural Networks and Multivariate Regression Methods. Eng. Geol. 2012, 131–132, 11–18.
- Saxena, S.; Panicker, L.; Gautam, S. Rheology of Indian Honey : Effect of Temperature and Gamma Radiation. Int. J. Food Sci. 2014, 2014, 1–6.
- Rahman, M. S.;. State Diagram of Foods: Its Potential Use in Food Processing and Product Stability. Trends Food Sci. Technol. 2006, 17, 129–141.