ABSTRACT
The phytochemical composition of essential oils and extracts of Tanacetum haussknechtii were investigated with GC–FID–MS and LC–MS/MS techniques and evaluated against oxidation, acetylcholinesterase, tyrosinase, and α-amylase enzymes. The major volatile constituents of T. haussknectii were found to be α- and β-pinene, and borneol. Caffeoylquinic acid derivatives and flavonoids were detected in the aqueous, alcohol, and ethyl acetate extracts. In DPPH assay, the methanol extracts exhibited the highest activity. TEAC assay resulted with superiority of all methanol and the capitula ethyl acetate extract. In β-carotene bleaching assay, linoleic acid was the best protected by the ethyl acetate extract of flower. The flower oil inhibited higher acetylcholinesterase activity than the remaining extracts. The flower ethyl acetate extract was found as the most effective inhibitor of α-amylase. The herb and the leaf+stem water extracts possess highest inhibitory effect on tyrosinase.
Abbreviations: AChE, acetylcholinesterase; BHT, butylated hydroxytoluene; DPPH, 2,2-diphenyl-1-picrylhydrazyl; DOPA, 3,4-dihydroxyphenylalanine; EO, essential oil; GA, gallic acid; GAE, gallic acid equivalent; GC–FID, gas chromatography—flame ionization detector; LC–MS/MS, liquid chromatography–mass spectrometry; MS, mass spectrometry; QE, quercetin equivalent; TCI, thiocholine iodide; TE, Trolox equivalent.
Introduction
Using natural products instead of synthetics has become an important issue in the world. Vascular plants comprising approximately 300 thousand species are the main source for production of natural pharmaceuticals, organic agriculture, cosmetics. The pathogenesis of Alzheimer’s disease is not fully discovered yet. There are several hypotheses on the pathogenesis of this disease. According to one of these hypotheses, healthy people have more acetylcholine neurotransmitter in their brain when compared with Alzheimer’s patients. Inhibiting acetylcholinesterase that is responsible for breakdown of acetylcholine increases the availability of acetylcholine.[Citation1] Therefore, searching for compounds that have inhibitory effect on AChE is considered to be an alternative to current drugs used for treatment of Alzheimer’s disease. Until now, galanthamine from Galanthus nivalis L.[Citation2], eserin from Physostigma venenosum L.[Citation3], and huperzin A from Huperzia serrata (Thumb.) Trev.[Citation4] were found to be pharmacologically active on inhibition of AChE. Galanthamine is prescribed in the United States and the European Union for Alzheimer’s patients. In contrast, owing to its adverse side effects, eserine cannot be used in the treatments. Moreover, huperzin A is still under phase-II clinical trial in China.
It is estimated that 415 million people in the world diabetes is the most common endocrine disease. In Turkey, more than 7 million people have diabetes mellitus. Patients with diabetes experience significant morbidity and mortality from micro- and macrovascular complications. The cost of diabetes to society is very high, and it is escalating every year. Turkey has the highest age-adjusted comparative prevalence (12.8% comparative prevalence) and the third-highest number of people with diabetes in the Europe. [Citation5] There are several approaches in therapy of diabetes. One of them is the inhibition of α-amylase enzyme to decrease digestion of carbohydrates. Nowadays, there is a serious demand for effective and safe inhibitors of α-amylase. In this context, natural compounds from plants and other sources may have a potential. Produced by Actinoplanes sp. fermentation, acarbose is the most common natural drug used for the treatment of diabetes clinically. The literature search revealed reports about the potential of Tanacetum species as antidiabetic agents: T. vulgare L.
Tyrosinase is an enzyme that occurs in mammalian melanocytes and is responsible for hydroxylation of monophenols to o-diphenols and catalization of o-diphenols to o-quinones. In the melanogenesis process, tyrosinase plays a key role in the hydroxylation of l-tyrosine and oxidation of L-DOPA to o-dopaquinone. After several non-enzymatic reactions, melanin is produced from the o-quinones. Inhibition of tyrosinase in the melanogenesis process may slow down the production of abnormal amounts of melanin and offers a solution for esthetic problems on human skin. Another important item, tyrosinase gene is expressed in the human substantia nigra. There is growing evidence that tyrosinase could play a central role in dopamine neurotoxicity as well as contribute to the neurodegeneration associated with Parkinson’s disease.[Citation6] In addition, tyrosinase is responsible for the color change such as browning of fruits and vegetables. In the literature, there are a number of papers about anti-tyrosinase effects of different types of phytochemicals. [Citation7,Citation8]
Reactive oxygen species are known to play a major role in the development of oxidative stress. Oxidative stress may lead to cardiac diseases, diabetes, inflammation, neurodegenerative disorders, cancer, anemia, and atherosclerosis in human body.[Citation9] Many synthetic drugs are produced to control oxidative stress. However, the financial obligations, accessibility issues, and particularly side effects of these synthetic drugs are the main obstacles in the prevention of oxidative stress. Acquiring natural antioxidants from many plant sources with minimal side effects and low cost gained importance against synthetic drugs.
Asteraceae family contains approximately 23,000 species in the world. Tanacetum L. is the third largest genus within Asteraceae and has 160 species worldwide.[Citation10] Tanacetum species spread to Europe, Asia, North Africa, and North America. Forty-six of these 160 species are grown naturally in Turkey.[Citation11] Tanacetum species have been used to cure or lower the pain of several diseases traditionally as well as for food purposes.[Citation12–Citation14] Uses of Tanacetum vulgare (L.) (tansy) as anthelmintic and Tanacetum parthenium (L.) Schultz Bip. (feverfew) as antipyretic are among the well-known traditional uses.[Citation15] Traditional uses of Tanacetum species are summarized in . Tanacetum haussknechtii (Bornm.) Grierson is an endemic of Turkey. Almost no published information about the chemistry and uses of T. haussknechtii is available, despite the local importance of the genus Tanacetum in the traditional health care system of Turkey. Phytochemical investigations on Tanacetum species resulted with high diversity of volatile and non-volatile constituents. A summary of previous phytochemical reports on Tanacetum species is presented in and .
Table 1. Traditional uses of Tanacetum taxa in Turkey.
Table 2. Literature survey on volatile compounds of Tanacetum species.
Table 3. Literature survey on non-volatile compounds of Tanacetum species.
In brief, α-pinene, β-pinene, camphene, sabinene, limonene, γ-terpinene, α-thujone, α-phellandrene, α-terpinene, p-cymene, bornyl acetate, borneol, chrysanthenyl acetate, camphor, α-terpineol, pinocarvone, β-caryophyllene, and caryophyllene oxide were reported as common volatiles[Citation10], while sesquiterpene lactones and flavonoids were detected as non-volatile constituents in the extracts. [Citation35,Citation36]
To the best of our knowledge, there is no any report on biological activity of T. haussknechtii. In this study, we aimed to reveal phytochemistry and biological properties of T. haussknechtii for the first time. The main aim of this paper was to give a first detailed characterization of the essential oil and extracts compositions and antioxidant, anti-acetylcholinesterase, anti-tyrosinase, and anti-amylase activities of an important but hitherto poorly studied traditional medicine of Turkey.
Materials and methods
Chemicals
2,2-Diphenyl-1-picrylhydrazyl (DPPH), 2,2′-azino-bis(3-ethylbenzothiazoline-6-sulfonic acid) (ABTS), 6-hydroxy-2,5,7,8-tetramethylchromane-2-carboxylic acid (Trolox), gallic acid, butylated hydroxytoluene (BHT), quercetin, β-carotene, linoleic acid, Tween 20, Folin & Ciocalteu’s phenol reagent (FCR), Tris (hydroxymethyl) aminomethane (ACS reagent), acetylcholinesterase (AChE) from Electrophorus electricus (electric eel, Type VI-S, 200–1000 units/mg protein), bovine serum albumin (BSA), acetylthiocholine iodide (ATCI), 5,5′-dithiobis(2-nitrobenzoic acid) (DTNB), galanthamine, tyrosinase from mushroom (EC 1.14.18.1), 3,4-dihydroxy-L-phenylalanine (L-DOPA), 1-naphthyl acetate, Fast Blue B Salt (dye content 95%), kojic acid, α-amylase from porcine pancreas (Type VI-B, ≥10 units/mg solid), acarbose, and galanthamine hydrobromide from Lycoris sp. were purchased from Sigma-Aldrich (St. Louis, MO, USA). Starch-soluble extra pure iodine and potassium iodide were purchased from Merck (Darmstadt, Germany). Sodium phosphate, disodium phosphate, aluminum chloride, ultrapure water, methanol (MeOH), dimethyl sulfoxide (DMSO), and ethyl acetate (EtAc) were extra pure analytical grade. A C9–C40 n-alkane standard solution was purchased from Fluka (Buchs, Switzerland).
General
Agilent 5975 GC-MSD system (Agilent, USA; SEM Ltd., Istanbul, Turkey) was equipped with the HP-Innowax FSC column (60 m × 0.25 mm id with 0.25 µm film thickness, Agilent, USA). The GC–FID analysis was performed with capillary GC using an Agilent 6890N GC system (SEM Ltd., Istanbul, Turkey). Microtiter plate assay was performed with Biotek Powerwave XS microplate reader. Ultrapure water (0.05 µS/cm) was obtained from a Direct-Q® Water Purification System (Germany). TLC sprayer Biostep Desaga (SG e1 BS130.605) was used for spraying of enzyme and reagent solutions. Spectrophotometric measurements were performed with spectrophotometer UV-PharmaSpec 1700 (Shimadzu). TLC Silica gel 60 F254 aluminum sheet (Merck) was used for thin-layer chromatographic experiments.
Plant material
T. haussknechtii was collected in the flowering stage in Sivas: Kangal-Gürün road, 16 km, 1545 m, 39° 07′ 52.0″ N, 37° 14′ 33.2″ E (August, 2015) and identified by Dr. M. Tekin (Trakya University). Voucher specimens (ESSE No. 15011) are maintained in Herbarium of the Faculty of Pharmacy (Anadolu University, Eskişehir, Turkey).
Essential oil isolation
Air-dried plant material (leaf+stem and capitula) was separately subjected to hydrodistillation (3 h) using Clevenger-type apparatus according to European Pharmacopoeia. Essential oil yields were calculated on moisture-free basis. The oils were diluted with n-hexane (10%, v/v) for GC–FID and GC/MS analysis.
Preparation of extracts
The leaf+stem and capitula of T. haussknechtii were powdered and separately subjected to maceration in ethyl acetate, methanol, and water at room temperature (drug/solvent ratio 1:10) for 24 h with continuous shaking. The filtrated organic phases of each plant part were evaporated until dryness in vacuo (MeOH, EtAc) or lyophilized (water) and preserved in the freeze dryer until the assays were performed. The dried extracts were dissolved in methanol (10 mg/mL) and used as the stock solutions prior subjection to biological activity screening, total phenols content and total flavonoids content evaluations.
Gas-chromatographic analysis
The GC/MS and GC–FID analyses were performed with an Agilent 5975 GC-MSD and Agilent 6890N GC systems (Agilent, USA; SEM Ltd., Istanbul, Turkey) equipped with the HP-Innowax FSC column (60 m × 0.25 mm id with 0.25 µm film thickness, Agilent, USA) in conditions reported earlier. [Citation54]
Identification and quantification of compounds
Identification of the volatile constituents was achieved as reported previously.[Citation54] Briefly, identification of the individual compounds was based on the following: (i) comparison of the GC/MS Relative Retention Indices (RRI) of the compounds on polar column determined relative to the retention times of a series of n-alkanes (C8–C40), with those of authentic compounds or literature data; (ii) computer matching with commercial mass spectral libraries: MassFinder software 4.0, Adams Library, Wiley GC/MS Library (Wiley, New York, NY, USA), and Nist Library, and comparison of the recorded spectra with literature data. Confirmation was also achieved using the in-house “Başer Library of Essential Oil Constituents” database, obtained from chromatographic runs of pure compounds performed with the same equipment and conditions ().
Table 4. Chemical composition of T. haussknechtii essential oils.
The non-volatile secondary metabolites were identified based on MS fragmentation pattern obtained with MS/MS detector and were matched with the data given in the references listed in and according to previously published procedure.[Citation55] Detailed description of phenolic compounds identification together with spectral data is given in the supplementary material.
Table 5. LC–MS/MS analysis results for T. haussknechtii extracts.
Antioxidant activity
Free radical scavenging assay (DPPH test)
The scavenging effect of the samples on DPPH free radical was determined using a modified method of Brand-Williams.[Citation80] The solutions of the EOs (30 mg/mL), the extracts (10 mg/mL), and standard (0.1 mg/mL) were prepared in methanol (MeOH). Moreover, 100 µL of the sample (EO/extract/standard) solution was mixed with 100 µL DPPH solution (0.08 mg/mL in MeOH) in 96-flat bottom well plate cells. The mixtures were allowed to stand in the dark for 30 min. Decrease in the absorbance was recorded at 517 nm. Gallic acid was used as a positive control. The experiments were performed in triplicate. The free radical scavenging activity of the samples was expressed as percentage of inhibition calculated according to Eq. (1):
where Abscontrol is the absorbance of the control (containing all reagents except the test compound), Abssample is the absorbance of the sample with added DPPH. The IC50 values were obtained by plotting the DPPH scavenging percentage of each sample against the sample concentration. Data were analyzed using the SigmaPlot software (Version 12.0).
ABTS radical cation scavenging activity (TEAC assay)
Trolox equivalent antioxidant capacity of the samples was estimated toward ABTS•+ according to the procedure described by Re et al. [Citation81] with slight modification. Moreover, 7 mM ABTS and 2.5 mM K2S2O8 dissolved in 10 mL ultrapure water were incubated in the dark for 16 h at room temperature to create ABTS•+ free radical cation. Prior to the assay, the ABTS•+ solution was diluted with absolute ethanol to an absorbance 0.7–0.8 at 734 nm. The solutions of the EOs (30 mg/mL), the extracts (10 mg/mL), and Trolox (3.0; 2.0; 1.0; 0.5; 0.25; 0.125 mM) were prepared in MeOH. Moreover, 10 µL of the sample (EO/extract/standard) solution was mixed with 990 µL of ABTS•+ solution, and 10 µL of MeOH mixed with ABTS•+ solution was used as control. The mixtures were allowed to stand in the dark for 30 min at room temperature. Gallic acid (0.1 mg/mL) was used as positive control. Decrease in the absorbance was recorded at 734 nm to obtain the linear Trolox equation. Experiments were performed in triplicate. ABTS•+ scavenging activity of the samples was expressed as Trolox equivalent antioxidant capacity and calculated using linear equation obtained for Trolox (y = 33.644x + 2.6523, r2 = 0.9942).
Lipid peroxidation inhibition (β-carotene bleaching test)
The lipid peroxidation inhibition of the samples was performed as described previously[Citation82] with slight modification. Briefly, 1.2 mL of β-carotene solution (1 mg/mL) prepared in chloroform was mixed with Tween 20 (1200 mg) and linoleic acid (120 mg). Chloroform was evaporated fully under vacuum (at 40°C) and N2. Then, 300 mL of oxygenated ultrapure water was added to the mixture and vigorously shaken. The emulsion obtained was freshly prepared before each experiment and stored in the dark. The solutions of the EOs (30 mg/mL), extracts (10 mg/mL), and BHT (standard, 1mg/mL) were prepared in MeOH. Moreover, 10 µL of the sample (EO/extract/standard) solution was mixed with 2 mL of β-carotene emulsion in 96-deep-well plate. In addition, 300 µL of this mixture was transferred to 96-well flat bottom plates, and the absorbance values were recorded (at 50°C) every 15 min of 120 min at 470 nm. Results were calculated according to Eq. (2):
where AA is the antioxidant activity, Abs0sample and Abs120sample are the absorbance of the sample at 0 min and 120 min, Abs0control and Abs120control are the absorbance of the control at 0 min and 120 min.
Total phenol content
The total phenolic contents of the extracts were determined as gallic acid equivalent (GAE) using FC reagent using the method of Singleton.[Citation83] Briefly, 50 µL of the sample, 3.9 mL ultrapure water, and 250 µL FCR were mixed in a test tube and vortexed. After 1–8 min incubation, 750 µL Na2CO3 (20%) was added to the mixture and vortexed again. The absorbance after 2 h at 25°C at 760 nm was compared with gallic acid calibration curve (y = 2.3503x+0.0347, r2 = 0.9922). The experiment was performed in triplicate.
Total flavonoid content
The total flavonoid contents of the extracts were determined as quercetin equivalents (QE) using AlCl3 reagent. [Citation84] Briefly, 200 µL of the sample, 200 µL of AlCl3 (2%), and 4.6 mL of absolute ethanol were mixed in tube. A blank consisting of 1 drop acetic acid instead of AlCl3 was prepared for each sample as well. After 40 min at 25°C incubation, the absorbance was recorded at 415 nm. The total flavonoid content values were calculated by comparison with quercetin calibration curve (y = 2.6386x – 0.1381, R2 = 0.9939).
Enzyme inhibitory activity
TLC assay for screening of ache inhibition
The anti-AChE activity assay on TLC plates described by Marston et al. [Citation85] has been used for rapid screening of enzyme inhibition by plant EOs and extracts. The enzyme activity was detected by the conversion of naphthyl acetate into naphthol and the formation of the purple-colored diazonium dye with Fast Blue B salt. Inhibitors of AChE produced white spots on the dye-colored background of the TLC plate. Briefly, the plate was previously washed with acetone and dried to eliminate possible contaminants. Each sample was applied on the plate in three different amounts (EOs in 0.4, 0.1, 0.04 mg, the extracts in 0.1, 0.02, 0.005 mg) as spot, and the solvent was removed under nitrogen gas. After drying of the spots, the plate was sprayed with 2 mL of AChE solution (3.33 U/mL in Tris buffer, pH 8.0 with addition of BSA) using a TLC-sprayer. The plate with loaded samples was incubated for 20 min at 37°C in humid atmosphere. For this purpose, the Petri dish with water was also incubated in the oven. The inhibition of AChE activity was detected using the reagent consisting of freshly prepared mixture (1:4) of naphthyl acetate (2.5 mg/mL in abs. ethanol) and Fast Blue Salt (2.5 mg/mL in water). This reagent (4 mL) was sprayed onto the plate to give a purple coloration. The active samples were determined from the colorless areas occurred on purple ground (within 30 min).
Microtiter assay for determination of ache inhibition
Acetylcholinesterase (AChE) inhibition of the samples was evaluated using Ellman’s method as previously reported [Citation86] with slight modification. Three buffers were used: (A) 50 mM Tris-HCl (pH = 8.0, in ultrapure water); (B) 0.1% BSA in buffer A; (C) 0.1 M NaCl and 0.02 M MgCl2•6H2O in buffer A. In the 96-well flat bottom plates, 25 µL of the sample (EO/extract/standard), 50 µL of buffer B, and 25 µL of AChE (0.22 U/mL in buffer A) solution were pipetted with 8-channel automatic pipette (Eppendorf Research® plus, Germany) and incubated for 15 min at 25°C. Then, 125 µL of Ellman’s reagent DTNB (3.0 mM in buffer C) and 25 µL of substrate ATCI (15 mM, in ultrapure water) were added. Hydrolysis of ATCI was monitored by the formation of the yellow 5-thio-2-nitrobenzoate anion as a result of the reaction of DTNB with thiocholines, catalyzed by enzymes at 412 nm utilizing a 96-well microplate reader (Biotek Powerwave XS, USA). The mixture was allowed to stand 15 min at 25°C, and the absorbance was recorded at 412 nm. Similarly, a blank (for eliminating the colors of the samples) was prepared by adding the sample solution to all reaction reagents and 25 µL of buffer instead of enzyme. The control wells contained all the reagents without the sample (the solvents of the samples instead were added). Galanthamine hydrobromide from Lycoris sp. (0.1 mg/mL) was used as positive control. The percentage inhibition was calculated according to Eq. (3):
where Abscontrol and Abscontrol blank are the absorbance of the control and its blank, and Abssample and Abssample blank are the absorbance of the sample and its blank. Data obtained from in vitro enzyme inhibition assays were expressed as the mean standard error (±SEM).
Tyrosinase inhibition
The tyrosinase inhibitory activity of the samples was measured using the modified dopachrome method with L-DOPA as substrate, as previously reported[Citation87] with slight modifications. In 96-well flat bottom plates, 80 µL of sodium phosphate buffer (0.1 M, pH = 6.8), 40 µL of the sample (EO/extract/standard) solution, and 40 µL of tyrosinase (33 U/mL in buffer, pH = 6.8) were pipetted with 8-channel automatic pipette and incubated for 10 min at 23°C. The reaction was initiated with the addition of 40 µL of the substrate L-DOPA (2.5 mM in buffer) to the mixture. The absorbance was recorded at 475 nm after 15 min incubation at 23°C. The sample blanks contained all reaction reagents and 40 µL of buffer instead of enzyme. The tyrosinase inhibition activity of the samples was expressed as the kojic acid equivalent (KAE) and calculated using the linear equation obtained for kojic acid (y = 1014.1X+11.798, r2 = 0.9958). Quercetin (1 mg/mL in methanol) was used as positive control.
α-Amylase inhibition
The effect of the samples on α-amylase was evaluated using the iodine/potassium iodide (I/KI) method. [Citation88] Briefly, 25 µL sample solution and 50 µL α-amylase (0.8 U/mL in 20 mM of sodium phosphate buffer pH = 6.9) pipetted in 96-well flat bottom plates and incubated for 10 min at 37°C. The reaction was initiated with addition of the substrate solution (50 µL 0.05% soluble starch solution in ultrapure water). Then, the mixture was subjected to a second incubation for 10 min at 37°C. After incubation, the reaction was stopped with 25 µL of HCl (1 M). Finally, 100 µL of I/KI was pipetted to the wells. The sample blanks contained all reaction reagents and 50 µL of buffer instead of enzyme. The control wells contained all the reagents without the sample (the solvents of the samples instead were added). The percentage inhibition was calculated according to Eq. (4):
where Abscontrol and Abscontrol blank are the absorbance of the control and its blank, and Abssample and Abssample blank are the absorbance of the sample and its blank. The α-amylase inhibition activity of the samples was expressed as the acarbose equivalent (AE) and calculated using the linear equation obtained for acarbose (y = 71.925X+41.653, r2 = 0.993).
Results and discussion
Our study aimed to elucidate further knowledge on the secondary metabolites of T. haussknechtii species and screened its volatile and non-volatile fraction for antioxidant, anti-AChE, anti-tyrosinase, and anti-α-amylase properties. The hydrodistillation of T. haussknechtii resulted with yellowish colored EOs with nice odor. The oil yields obtained from the leaf+stem and the capitula were 0.09% and 0.08%, respectively. Different parts of T. haussknechtii (herb, leaf+stem, capitula) were extracted with methanol, ethyl acetate, and water. The yields of the extracts are presented in . The GC–FID and GC/MS techniques allow us to determine qualitative and quantitative profiles of T. haussknechtii EOs. The list of detected volatile compounds with their relative percentages, retention indices, and method of identification is given in in order of their elution on the HP-Innowax FSC column. The GC analysis of the leaf+stem and the capitula oils resulted with 60 and 56 constituents, representing 92.0% and 91.4% of the total oils, respectively. The EOs were characterized with high diversity of volatile constituents that were classified as mono- and sesquiterpene hydrocarbons and their oxygenated forms. The EOs from the leaf+stem and the capitula were characterized with high abundance of monoterpenes with α-pinene (14.5% and 12.3%, resp.), β-pinene (7.2% and 6.4%, resp.), santolinatriene (1.7% and 7.4%, resp.), and borneol (17.2% and 20.5%, resp.) as major constituents. In the literature, there are several reports about Tanacetum species with pinene and borneol as major volatiles: T. argenteum (Lam.) Willd. ssp. argenteum (L.) All., T. argenteum (Lam.) Willd. subsp. canum (C. Koch) Grierson var. canum (C. Koch) Grierson, T. densum (Lab.) Schultz Bip. ssp. amani Heywood, T. densum (Lab.) Schultz Bip. ssp. amani Heywood. [Citation89–Citation91]
Table 6. Yield of extracts and essential oil, total phenols content, and total flavonoids content in T. haussknechtii extracts.
A spectrophotometric evaluation of the extracts with FCR revealed that all the methanol extracts were characterized with high abundance of the phenols. The total phenols contents in the extracts ranged from 36.0 ± 0.46 to 5.94 ± 0.07 mg GAE/g extract. The total flavonoids contents in the extracts ranged from 30.44 ± 0.56 to 5.5 ± 0.14 mg QE/g extract. The extracts obtained with ethyl acetate from the capitula were found as rich with flavonoids. All results are given in .
LC–MS/MS analysis of T. haussknechtii extracts obtained with different polarity solvents resulted with identification of 47 phenolic compounds. Their mass spectral data are given in . According to LC–MS/MS results, the main compounds of the methanol extracts were 5-caffeoylquinic and dicaffeoylquinic acids (3,5/4,5) (). An unknown C hexoside at m/z 785 [M-H]− was also determined in the herb and capitula methanol extracts. However, they were not detected in the leaf and stem extracts. That fact lets us to consider that the main source of this unknown C hexoside is the capitula. Beside 3,5-dicaffeoylquinic acid, quercetin and derivatives were also determined as dominant in ethyl acetate extracts. The water extracts were distinguished with considerably low amount of phenolic compounds. Representative LC–MS/MS chromatogram obtained for the methanol extract from T. haussknechtii herb is presented in .
Figure 1. 3,5-Dicaffeoylquinic acid determined in the methanol and ethyl acetate extracts of T. haussknechtii.
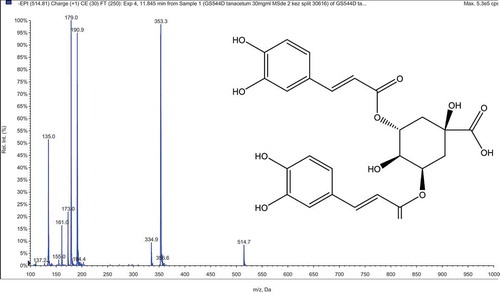
Figure 2. LC–MS/MS chromatogram of the methanol extract from the herb of T. haussknechtii. Description of the peaks is given in .
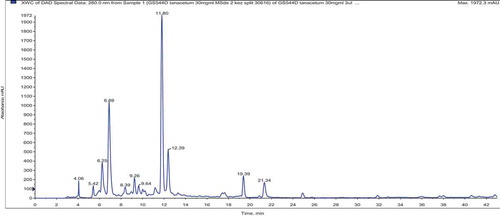
Antioxidant activity
Different antioxidant activity assays have been developed and applied for evaluation of antioxidative potential of natural products. Depending upon the reactions involved, the antioxidant activity assays can roughly be classified into two types: assays based on electron transfer (ET) and assays based on hydrogen atom transfer (HAT) reactions. For evaluation of T. haussknechtii antioxidant effects the both type assays were applied; ET: total phenols assay by Folin-Ciocalteu reagent, DPPH test, and Trolox equivalent antioxidant capacity assay; HAT: β-Carotene bleaching test. The results of T. haussknechtii EOs and extracts effects in different antioxidant activity assays are summarized in .
Table 7. Antioxidant activity of T. haussknechtii§ essential oils and extracts.
Free radical scavenging activity
The EOs and the extracts of T. haussknechtii were evaluated for free radical scavenging ability using DPPH reagent. The decrease in the absorbance at 517 nm induced by antioxidants in the samples was recorded and used for calculation of IC50 values. In DPPH assay, all the methanol extracts that are found as the richest ones in phenolics (34.14; 36.0; and 34.82 mg GAE/g extr.) exhibited the highest free radical scavenging activity (IC50 0.12; 0.09; and 0.15 mg/mL). The extract obtained from the leaf+stem revealed the maximum activity with IC50 value 0.09 ± 0.01 mg/mL. The respective extracts obtained with ethyl acetate and water showed lower activity, and EOs were not active ().
Trolox equivalent antioxidant capacity
Antioxidant capacities of the EOs and the extracts were determined by their ABTS•+ free radical scavenging activity. The results were compared with Trolox and expressed as Trolox equivalents (TE). ABTS•+ radical scavenging test resulted with superiority of all methanol extracts (2.68; 2.64; and 2.58 TE mM) and the capitula ethyl acetate extract (2.64 ± 0.11 TE mM). However, EOs did not demonstrate protection effect against lipid peroxidation in this test ().
Inhibition of lipid peroxidation
The effects of T. haussknechtii EOs and the extracts on lipid peroxidation were evaluated with the β-carotene/linoleic acid system. The β-carotene bleaching test uses a model lipid substrate (linoleic acid) in an emulsified form, and the method lies in between methods employing only model substrates (e.g., DPPH) and those using real lipids. Antioxidant activities of the samples were expressed as percent inhibition of lipid oxidation. EOs were not able to inhibit linoleic acid peroxidation at concentration 1.0%, but all the extracts obtained with MeOH and all the capitula extracts demonstrated significant inhibitory effect on the lipid peroxidation. In β-carotene bleaching assay, linoleic acid was the best protected by the capitula ethyl acetate (53.68 ± 5.19%) and methanol (49.95 ± 4.35%) extracts against the oxidative decomposition. The leaf+stem methanol extract also demonstrated noticeable protection effect (46.89 ± 4.65%) ().
In general, the alcohol extracts of T. haussknechtii demonstrated the highest antioxidant activity making the biggest contribution into antioxidant profile of the plant. However, the essential oil was found to be weak on scavenging of free radicals and inhibition of lipid peroxidation. Among the phenolic compounds detected in the extracts of T. haussknechtii, the caffeoylquinic acid derivatives and flavonoids are the major components. All these constituents have earlier been mentioned to have approved antioxidant activity. Actually, luteolin[Citation92], quercetin[Citation93], apigenin[Citation94], caffeoylquinic acid derivatives[Citation95], and caffeic acid[Citation96] are important contributors into antioxidant status of the related extracts. In the literature, there are several reports about antioxidant properties of the above-mentioned phenols. [Citation97,Citation98]
Enzyme inhibition activity
Anti-acetylcholinesterase activity
The drugs approved and licensed for the treatment of neurodegenerative diseases inhibit AChE enzyme. Although, they belong to the same pharmacologically active group, they have different chemical structures: some of them possess some side effects. Besides, there is still no approved cure for the severe type of Alzheimer’s disease. Therefore, there is still demand for discovery of new anti-Alzheimer drug candidates. In our study, screening of the samples for anti-acetylcholinesterase activity was performed using both the TLC rapid test and the spectrophotometric evaluation. In our experiments, the “dot-blot” type examination was performed for preliminary TLC-test of anti-AChE activity of the extracts and EOs. The TLC application was chosen, because it gives quick access to information concerning the activity of the samples examined. The test relies on the cleavage by acetylcholinesterase of 1-naphthyl acetate to form 1-naphthol, which in turn reacts with Fast Blue B salt to give a purple-colored diazonium dye. The preliminary test revealed that the water extracts of T. haussknechtii did not demonstrate inhibitory effect on AChE. Therefore, they were eliminated and was not included in the second TLC-test. The second TLC-test revealed that the methanol and ethyl acetate extracts and essential oils were found to have inhibitory effect on enzyme ().
Figure 3. TLC-autography for screening of T. haussknechtii essential oils and extracts for anti-AChE activity. A: Stem+Leaf EO; B: Flower EO; C: Methanol extract of the herb; D: Ethyl acetate extract of the herb; E: Methanol extract of the stem+leaf; F: Ethyl acetate extract of the stem+leaf; G: Methanol extract of the flower; H: Ethyl acetate extract of the flower; K: Galanthamine; L: Galanthamine; M: Methanol (5 uL).
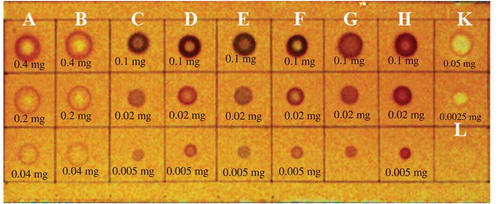
Microtiter plate assay was performed using Ellmans’s reagent. Electric eel AChE was used as the enzyme source, while ATCI was employed as substrate of the reaction. The hydrolysis of ATCI was monitored by the formation of the yellow 5-thio-2-nitrobenzoate anion as a result of the reaction of DTNB with thiocholines, catalyzed by enzymes at a wavelength of 412 nm utilizing a 96-well microplate reader. AChE enzyme inhibition effects were expressed as percent inhibition and compared with galanthamine hydrobromide. The capitula EO inhibited acetylcholinesterase activity (51.2 ± 2.5%) (). The EOs of T. haussknechtii were found to contain noticeable amounts of monoterpenoids with pinene and camphene skeletons, namely α-pinene (14.5–12.3%), β-pinene (7.2–6.4%), 1,8-cineole (3.6–3.1%) and borneol (17.2–20.5%). In a previous research, these constituents were found to be uncompetitive reversible inhibitors of AChE.[Citation99] Therefore, anti-AChE activity of the tested T. haussknechtii EOs may be related to the presence of these compounds. The extracts of T. haussknechtii demonstrated anti-AChE activity ranged between 19.2% and 37.1%. As resulted from LC-MS/MS analysis, the extracts contained caffeoylquinic acid and its derivatives as well as flavonoid constituents. These compounds have been earlier demonstrated to be cholinesterase inhibitors.[Citation98]
Table 8. Enzyme inhibitory activity of T. haussknechtii§ essential oils and extracts.
Anti-amylase activity
Antidiabetic effects of the EOs and the extracts were estimated by their α-amylase enzyme inhibitory properties. Only extracts obtained with methanol and ethyl acetate showed noticeable inhibition of the enzyme. The capitula ethyl acetate extract was found to be the most inhibited (356.9 ± 0.06 mg AEs/g Extr) of α-amylase activity. However, the water extracts have no effect on α-amylase enzyme (). It should be considered that ethyl acetate extract of T. haussknechtii contained derivatives of caffeoylquinic acid and flavonoids. In fact, the above-mentioned compounds have earlier been reported to have antidiabetic effect. [Citation100]
Anti-tyrosinase activity
Tyrosinase is a key enzyme in melanogenesis process as well as in pathogenesis of Parkinson’s disease. Melanin is responsible for the protection against harmful ultraviolet irradiation, which can cause significant pathological conditions, such as skin cancers. Natural inhibitors of tyrosinase are in demand. Today, tyrosinase inhibitors from natural sources attract more attention owing to their safety, cost, and availability compared to chemically synthesized compounds. Different types of phenolics, flavonoids, stilbenes, chalkones, and hydroquinones have been reported as effective inhibitors of tyrosinase. Also, the literature search revealed reports about the effect of Tanacetum parthenium extracts on tyrosinase activity.[Citation101] Considering the above mentioned, we investigated tyrosinase inhibitory activities (on L-DOPA) of T. haussknechtii EO and the extracts. The method described by Masuda with slight modifications was applied, and the activity was expressed as a well-known tyrosinase inhibitor kojic acid equivalents. The herb, capitula ethyl acetate extract, and the leaf+stem water extract possess the highest inhibitory effect (20.09–18.99 mg KAE/g extr) on tyrosinase. The extracts obtained with MeOH from the leaf+stem and capitula were not active ().
Conclusion
The present work is the first investigation of Tanacetum haussknechtii essential oils and extracts obtained with different polarity solvents from different plant parts. We performed phytochemical investigations using GC–FID, GC/MS, LC–MS/MS, TLC, and spectrophotometric techniques. Based on biological activity assays, antioxidant properties and inhibitory effects of T. haussknechtii on acetylcholinesterase, α-amylase, and tyrosinase enzymes were investigated. The essential oils and extracts of the plant were found to contain valuable phytochemicals with significant biological activities. Our study is the first report about the antioxidant and inhibitory effects of this species against enzymes involved in important neurobiological, carbohydrate digestive, and skin pigmentation processes. The results provide strong scientific evidence for traditional uses of Tanacetum species. Finally, T. haussknechtii could be considered as the valuable source for isolation of active natural constituents for food supplements and therapeutic applications.
Declaration of interest
All authors of the manuscript declare that they do not have financial/commercial conflicts of interest.
LJFP_A_1370600_Tanacetum_Supplement_material.docx
Download MS Word (568.9 KB)Additional information
Funding
References
- Adewusi, E. A.; Steenkamp, V. In Vitro Screening for Acetylcholinesterase Inhibition and Antioxidant Activity of Medicinal Plants from Southern Africa. Asian Pac. J. Trop. Med. 2011, 4(10), 829.
- Pearson, V. E.;. Galantamine: A New Alzheimer Drug with a Past Life. Ann. Pharmacother. 2001, 35(11), 1406–1413.
- Mukherjee, P. K.;, et al. Acetylcholinesterase Inhibitors from Plants. Phytomedicine. 2007, 14(4), 289–300.
- Ashani, Y.; Peggins, J. O.; Doctor, B. P. Mechanism of Inhibition of Cholinesterases by Huperzine A. Biochem. Biophys. Res. Commun. 1992, 184(2), 719–726.
- IDF. In IDF Diabetes Atlas, 7th ed.; Cavan, D., Da Rocha Fernandes, J., Makaroff, L., Ogurtsova, K., Webber, S., ed.; Belgium:International Diabetes Federation. 2015.
- Xu, Y.;, et al. Tyrosine mRNA Is Expressed in Human Substantia Nigra. Mol. Brain Res. 1997, 45(1), 159–162.
- Aumeeruddy-Elalfi, Z.; Gurib-Fakim, A.; Mahomoodally, M. F. Kinetic Studies of Tyrosinase Inhibitory Activity of 19 Essential Oils Extracted from Endemic and Exotic Medicinal Plants. South. Afr. J. Bot. 2016, 103, 89–94.
- Dong, X.;, et al. Preparation of Tyrosinase Inhibitors and Antibrowning Agents Using Green Technology. Food Chem. 2016, 197, 589–596.
- Simonian, N.; Coyle, J. Oxidative Stress in Neurodegenerative Diseases. Annu. Rev. Pharmacol. Toxicol. 1996, 36(1), 83–106.
- Kumar, V.; Tyagi, D. Chemical Composition and Biological Activities of Essential Oils of Genus Tanacetum—A Review. J. Pharmacogn. Phytochem. 2013, 2(3), 155–159.
- Tekin, M.; Kartal, C. Comparative Anatomical Investigations on Six Endemic Tanacetum (Asteraceae) Taxa from Turkey. Pakistan J. Bot. 2016, 48(4), 1501–1515.
- Grieve, M.;. A Modern Herbal the Medicinal Culinary Cosmetic and Economic Properties Cultivation and Folk-Lore of Herbs, Grases Fungi, Shrubs & Trees with All Their Modern Uses; US, New York:Dover Publications Inc. 1970.
- Luczaj, L.;. Ethnobotanical Review of Wild Edible Plants of Slovakia. Acta Soc. Bot. Pol. 2012, 81(4), 245–255.
- Luczaj, L.;, et al. Wild Food Plant Use in 21 St Century Europe, the Disapperance of Old Traditions and the Search for New Ciusines Involving Wild Edibles. Acta Soc. Bot. Pol. 2012, 81(4), 359–370.
- Baytop, T.;. Treatment with Plants in Turkey (Past and Present), Istanbul University: Nobel Tıp Kitabevleri, Turkey, Istanbul. 1984; Vol. 2.
- Altundag, E.; Ozturk, M. Ethnomedicinal Studies on the Plant Resources of East Anatolia, Turkey. Procedia Soc. Behav. Sci. 2011, 19, 756–777.
- Tuzlacı, E.; Erol, M. K. Turkish Folk Medicinal Plants. Part II: Eğirdir (Isparta). Fitoterapia. 1999, 70(6), 593–610.
- Polatoglu, K.;, et al. Essential Oil Composition and Antibacterial Activity of Tanacetum Argenteum (Lam.) Willd. Ssp. Argenteum and T. Densum (Lab.) Schultz Bip. Ssp. Amani Heywood from Turkey. J. Oleo Sci. 2010, 59(7), 361–367.
- Bagci, E.; Kocak, A. Essential Oil Composition of Two Endemic Tanacetum (T. Nitens (Boiss. &Noe) Grierson and T. Argenteum (Lam.) Willd. Subsp. Argenteum)(Asteraceae) Taxa, Growing Wild in Turkey. Ind. Crops Prod. 2010, 31(3), 542–545.
- Polatoglu, K.;, et al. Essential Oil Composition and DPPH Scavenging Activity of Endemic Tanacetum Mucroniferum Hub.–Mor. & Grierson from Turkey. J. Ess. Oil Bear. Plants. 2012, 15(1), 66–74.
- Polatoğlu, K.;, et al. Essential Oil Composition and Antimicrobial Activities of Tanacetum Chiliophyllum (Fisch. & Mey.) Schultz Bip. Var. Monocephalum Grierson from Turkey. Nat. Prod. Res. 2012, 6(2), 184–188.
- Polatoglu, K.;, et al. Essential Oil Composition of Tanacetum Kotschyi from Turkey. Chem. Nat. Compd. 2011, 47(2), 297–299.
- Polatoğlu, K.;, et al. Essential Oil Composition of Endemic Tanacetum Zahlbruckneri (Náb.) And Tanacetum Tabrisianum (Boiss.) Sosn. And Takht. From Turkey. Nat. Prod. Res. 2011, 25(6), 576–584.
- Akpulat, H. A.;, et al. Composition of the Essential Oils of Tanacetum Argyrophyllum (C. Koch) Tvzel. Var. Argyrophyllum and Tanacetum Parthenium (L.) Schultz Bip. (Asteraceae) from Turkey. Biochem. Syst. Ecol. 2005, 33(5), 511–516.
- Hendriks, H.; Bos, R.; Woerdenbag, H. J. The Essential Oil of Tanacetum Parthenium (L.) Schultz‐Bip. Flavour Frag. J. 1996, 11(6), 367–371.
- Javidnia, K.;, et al. Antimicrobial and Antioxidant Activity and Chemical Composition of the Essential Oil of Tanacetum Macrophyllum (Waldst. Et Kit.) Schultz. Bip. J. Essent. Oil Res. 2010, 22(2), 186–188.
- Salamci, E.;, et al. Chemical Compositions, Antimicrobial and Herbicidal Effects of Essential Oils Isolated from Turkish Tanacetum Aucheranum and Tanacetum Chiliophyllum Var. Chiliophyllum. Biochem. Syst. Ecol. 2007, 35(9), 569–581.
- Özer, H.;, et al. Essential Oil Composition of Tanacetum Sorbifolium (Boiss.) Grierson from Turkey. Flavour Frag. J. 2006, 21(3), 543–545.
- Gören, N.; Demirci, B.; Başer, K. Composition of the Essential Oils of Tanacetum Spp. From Turkey. Flavour Frag. J. 2001, 16(3), 191–194.
- Başer, K.;, et al. Composition of the Essential Oils of Tanacetum Armenum (DC.) Schultz Bip., T. Balsamita L., T. Chiliophyllum (Fisch. & Mey.) Schultz Bip. Var. Chiliophyllum and T. Haradjani (Rech. Fil.) Grierson and the Enantiomeric Distribution of Camphor and Carvone. Flavour Frag. J. 2001, 16(3), 195–200.
- Yousefzadi, M.;, et al. Cytotoxicity, Antimicrobial Activity and Composition of Essential Oil from Tanacetum Balsamita L. Subsp. Balsamita. Nat. Prod. Commun. 2009, 4(1), 119–122.
- Bagci, E.;, et al. Composition and Antimicrobial Activity of the Essential Oils of Tanacetum Balsamita L. Subsp. Balsamita and T. Chiliophyllum (Fisch. Et Mey.) Schultz Bip. Var. Chiliophyllum (Asteraceae) from Turkey. J. Ess. Oil Bear. Plants. 2008, 11(5), 476–484.
- Shafaghat, A.;. Antibacterial Activity and Sesquiterpenoid Contents of the Essential Oil of Tanacetum Punctatum (Desr.) Grierson. J. Ess. Oil Bear. Plants. 2012, 15(2), 270–275.
- Maxia, A.;, et al. Chemical Composition and Biological Activity of Tanacetum Audibertii (Req.) DC. (Asteraceae), an Endemic Species of Sardinia Island, Italy. Ind. Crops Prod. 2015, 65, 472–476.
- Gören, N.; Arda, N.; Çaliskan, Z. Chemical Characterization and Biological Activities of the Genus Tanacetum (Compositae). Stud. Nat. Prod. Chem. 2002, 27, 547–658.
- Abad, M. J.; Bermejo, P.; Villar, A. An Approach to the Genus Tanacetum L. (Compositae): Phytochemical and Pharmacological Review. Phytother. Res. 1995, 9(2), 79–92.
- Gören, N.;, et al. A Guaianolide from Tanacetum Argenteum Subsp. Flabellifolium. Phytochemistry. 1996, 42(3), 757–760.
- Sanz, J. F.; Marco, J. A. NMR Studies of Tatridin A and Some Related Sesquiterpene Lactones from Tanacetum Vulgare. J. Nat. Prod. 1991, 54(2), 591–596.
- Gonzalez, A. G.;, et al. Sesquiterpene Lactones from Tanacetum Ferulaceum. Phytochemistry. 1990, 29(7), 2339–2341.
- Milbrodt, M.; Schröder, F.; König, W. A. 3, 4-β-Epoxy-8-deoxycumambrin B, A Sesquiterpene Lactone from Tanacetum Parthenium. Phytochemistry. 1997, 44(3), 471–474.
- Gören, N.;, et al. Sesquiterpene Lactones with Antibacterial Activity fromTanacetum Densum Subsp. Sivasicum. Phytochemistry. 1992, 31(1), 101–104.
- Gören, N.; Tahtasakal, E. Constituents of Tanacetum Densum Subsp. Eginense. Phytochemistry. 1994, 36(5), 1281–1282.
- Bohlmann, F.; Zdero, C. Sesquiterpene Lactones and Other Constituents from Tanacetum Parthenium. Phytochemistry. 1982, 21(10), 2543–2549.
- De Vivar, A. R.; Jimenez, H. Structure of Santamarine, a New Sesquiterpene Lactone. Tetrahedron. 1965, 21(7), 1741–1745.
- González, A. G.;, et al. Sesquiterpene Lactones and Other Constituents of Tanacetum Species. Phytochemistry. 1992, 31(5), 1821–1822.
- Gören, N.;, et al. Sesquiterpene Lactones from Tanacetum Argenteum. Phytochemistry. 1994, 36(2), 389–392.
- Yunusov, A. I.; Sidyakin, G. P. Pyrethrin-A New Guaianolid from Pyrethrum Parthenifolium. Khim. Prir. Soedin. 1983, 19(4), 532–533.
- Romo, J.;, et al. Constituents of Artemisia and Chrysanthemum Species-The Structures of Chrysartemins A and B. Phytochemistry. 1970, 9(7), 1615–1621.
- Gören, N.; Jakupovic, J. Glaucolide-Like Sesquiterpene Lactones from Tanacetum Albipannosum. Phytochemistry. 1990, 29(9), 3031–3032.
- Sashida, Y.;, et al. Sesquiterpene Lactones from Pyrethrum Flowers. Phytochemistry. 1983, 22(5), 1219–1222.
- Stepanov, T. A.;, et al. Tanacetum Sibiricum Flavonoids. Khim. Prir. Soedin. 1981, 4, 519–520.
- Adikhodzhaeva, K. B.;, et al. Chemical Composition of the Preparation Tanacin from the Flowers of Tanacetum Vulgare L. Farmatsiia. 1977, 27(3), 24–28.
- Stepanov, T. A.; Sheichenko, V. I.; Smirnova, L. P. Tanacetum Sibiricum Flavanones. Khim. Prir. Soedin. 1981, 6, 721–728.
- Schepetkin, I. A.;, et al. Inhibition of Human Neutrophil Responses by the Essential Oil of Artemisia Kotuchovii and Its Constituents. J. Agric. Food Chem. 2015, 63(20), 4999–5007.
- Haliloglu, Y.;, et al. Phytochemicals, Antioxidant and Antityrosinase Activities of Achillea Sivasica Celik and Akpulat. Int. J. Food Prop. 2017., 20 (S1), S693-S706.
- Noorizadeh, H.; Farmany, A.; Noorizadeh, M. Application of GA-PLS and GA-KPLS Calculations for the Prediction of the Retention Indices of Essential Oils. Química Nova. 2011, 34(8), 1398–1404.
- Liu, K.;, et al. Composition, Irregular Terpenoids, Chemical Variability and Antibacterial Activity of the Essential Oil from Santolina Corsica Jordan Et Fourr. Phytochemistry. 2007, 68(12), 1698–1705.
- Maggio, A.;, et al. Chemical Composition of Essential Oil from Italian Populations of Artemisia Alba Turra (Asteraceae). Molecules. 2012, 17(9), 10232–10241.
- Palá-Paúl, J.;, et al. Analysis of the Volatile Components of Lavandula Canariensis (L.) Mill., A Canary Islands Endemic Species, Growing in Australia. Biochem. Syst. Ecol. 2004, 32(1), 55–62.
- Noorizadeh, H.; Farmany, A. Exploration of Linear and Nonlinear Modeling Techniques to Predict of Retention Index of Essential Oils. J. Chin. Chem. Soc. 2010, 57(6), 1268–1277.
- Cavalli, J. F.;, et al. Composition and Chemical Variability of the Bark Oil of Cedrelopsis Grevei H. Baillon from Madagascar. Flavour Fragr J. 2003, 18(6), 532–538.
- Cheraif, I.;, et al. Chemical Composition and Antimicrobial Activity of Essential Oils of Cupressus Arizonica Greene. Biochem. Syst. Ecol. 2007, 35, 813–820.
- Bastos, D. H.;, et al. Phenolic Antioxidants Identified by ESI-MS from Yerba Mate (Ilex Paraguariensis) and Green Tea (Camelia Sinensis) Extracts. Molecules. 2007, 12(3), 423–432.
- Clifford, M. N.;, et al. Characterization by LC-MS N of Four New Classes of Chlorogenic Acids in Green Coffee Beans: Dimethoxycinnamoylquinic Acids, Diferuloylquinic Acids, Caffeoyl-Dimethoxycinnamoylquinic Acids, and Feruloyl-Dimethoxycinnamoylquinic Acids. J. Agric. Food Chem. 2006, 54(6), 1957–1969.
- Spínola, V.; Pinto, J.; Castilho, P. C. Identification and Quantification of Phenolic Compounds of Selected Fruits from Madeira Island by HPLC-DAD–ESI-MSn and Screening for Their Antioxidant Activity. Food Chem. 2015, 173, 14–30.
- Lin, L.-Z.; Harnly, J. M. A Screening Method for the Identification of Glycosylated Flavonoids and Other Phenolic Compounds Using A Standard Analytical Approach for All Plant Materials. J. Agric. Food Chem. 2007, 55(4), 1084–1096.
- Clifford, M. N.;, et al. Hierarchical Scheme for LC-MS N Identification of Chlorogenic Acids. J. Agric. Food Chem. 2003, 51(10), 2900–2911.
- Kapp, K.;, et al. Commercial Peppermint (Mentha× Piperita L.) Teas: Antichlamydial Effect and Polyphenolic Composition. Food Res. Int. 2013, 53(2), 758–766.
- Navarro-González, I.;, et al. Nutritional Composition and Antioxidant Capacity in Edible Flowers: Characterisation of Phenolic Compounds by HPLC-DAD-ESI/MS. Int. J. Mol. Sci. 2015, 16(1), 805–822.
- Lin, L.-Z.; Harnly, J. M. Identification of the Phenolic Components of Chrysanthemum Flower (Chrysanthemum Morifolium Ramat). Food Chem. 2010, 120(1), 319–326.
- Muresan, M.;, et al. Screening of Polyphenolic Compounds, Antioxidant and Antimicrobial Properties of Tanacetum Vulgare from Transylvania. Studia Universitatis Babes-Bolyai, Chemia. 2015, 60(1), 127-138.
- Bohm, B. A.; Stuessy, T. F. Flavonoids of the Sunflower Family (Asteraceae); Austria, Wien: Springer Science & Business Media, 2001.
- Clifford, M. N.; Knight, S.; Kuhnert, N. Discriminating between the Six Isomers of Dicaffeoylquinic Acid by LC-MS N. J. Agric. Food Chem. 2005, 53(10), 3821–3832.
- Guimarães, R.;, et al. Nutrients, Phytochemicals and Bioactivity of Wild Roman Chamomile: A Comparison between the Herb and Its Preparations. Food Chem. 2013, 136(2), 718–725.
- Barros, L.;, et al. Characterization of Phenolic Compounds in Wild Medicinal Flowers from Portugal by HPLC–DAD–ESI/MS and Evaluation of Antifungal Properties. Ind. Crops Prod. 2013, 44, 104–110.
- Schinella, G. R.;, et al. Anti‐Inflammatory Effects of South American Tanacetum Vulgare. J. Pharm. Pharmacol. 1998, 50(9), 1069–1074.
- Long, C.;, et al. Bioactive Flavonoids of Tanacetum Parthenium Revisited. Phytochemistry. 2003, 64(2), 567–569.
- Mitreski, I.;, et al. Polyphenols in Representative Teucrium Species in the Flora of R. Macedonia: LC/DAD/ESI-MS (N) Profile and Content. Nat. Prod. Commun. 2014, 9(2), 175–180.
- Abad, M. J.;, et al. Flavonoids and a Sesquiterpene Lactone from Tanacetum Microphyllum Inhibit Anti-Inflammatory Mediators in LPS-stimulated Mouse Peritoneal Macrophages. Planta Med. 2004, 70(01), 34–38.
- Brand-Williams, W.; Cuvelier, M. E.; Berset, C. L. W. T. Use of a Free Radical Method to Evaluate Antioxidant Activity. LWT-Food Sci. Technol. 1995, 28(1), 25–30.
- Re, R.;, et al. Antioxidant Activity Applying an Improved ABTS Radical Cation Decolorization Assay. Free Radic. Biol. Med. 1999, 26(9), 1231–1237.
- Baderschneider, B.;, et al. Antioxidants in White Wine (Cv. Riesling): I. Comparison of Different Testing Methods for Antioxidant Activity. VITIS-J. Grapevine Res. 2015, 38(3), 127.
- Singleton, V. L.; Orthofer, R.; Lamuela-Raventos, R. M. Analysis of Total Phenols and Other Oxidation Substrates and Antioxidants by Means of Folin-Ciocalteu Reagent. Meth. Enzymol. 1999, 299, 152–178.
- Miliauskas, G.; Venskutonis, P. R.; Van Beek, T. A. Screening of Radical Scavenging Activity of Some Medicinal and Aromatic Plant Extracts. Food Chem. 2004, 85(2), 231–237.
- Marston, A.; Kissling, J.; Hostettmann, K. A Rapid TLC Bioautographic Method for the Detection of Acetylcholinesterase and Butyrylcholinesterase Inhibitors in Plants. Phytochemical Anal. 2002, 13(1), 51–54.
- Ellman, G. L.;, et al. A New and Rapid Colorimetric Determination of Acetylcholinesterase Activity. Biochem. Pharmacol. 1961, 7(2), 88–95.
- Masuda, T.;, et al. Screening for Tyrosinase Inhibitors among Extracts of Seashore Plants and Identification of Potent Inhibitors from Garcinia Subelliptica. Biosci. Biotechnol. Biochem. 2005, 69(1), 197–201.
- Zengin, G.;, et al. Sideritis Galatica Bornm.: A Source of Multifunctional Agents for the Management of Oxidative Damage, Alzheimer’s’s and Diabetes Mellitus. J. Funct. Foods. 2014, 11, 538–547.
- Ali, A.;, et al. Chemical Composition, Larvicidal, and Biting Deterrent Activity of Essential Oils of Two Subspecies of Tanacetum Argenteum (Asterales: Asteraceae) and Individual Constituents against Aedes Aegypti (Diptera: Culicidae). J. Med. Entomol. 2014, 51(4), 824–830.
- Bagci, E.;. Composition of the Essential Oils of Tanacetum Densum (Lab.) Schultz Bip. Subsp. Amani and T. Densum (Lab.) Schultz Bip. Subsp. Laxum (Asteraceae) from Turkey. Asian J. Chem. 2009, 21, 6547–6554.
- Polatoglu, K.;, et al. Antibacterial Activity and the Variation of Tanacetum Parthenium (L.) Schultz Bip. Essential Oils from Turkey. J. Oleo. Sci. 2010, 59(4), 177–184.
- Seelinger, G.; Merfort, I.; Schempp, C. M. Anti-Oxidant, Anti-Inflammatory and Anti-Allergic Activities of Luteolin. Planta Med. 2008, 74(14), 1667–1677.
- Baghel, S. S.;, et al. A Review of Quercetin: Antioxidant and Anticancer Properties. World J. Pharm. Pharmaceutical Sci. 2012, 1(1), 146–160.
- Singh, J. P. V.;, et al. Protective Role of Apigenin on the Status of Lipid Peroxidation and Antioxidant Defense against Hepatocarcinogenesis in Wistar Albino Rats. Phytomedicine. 2004, 11(4), 309–314.
- Nakajima, Y.;, et al. Water Extract of Propolis and Its Main Constituents, Caffeoylquinic Acid Derivatives, Exert Neuroprotective Effects via Antioxidant Actions. Life Sci. 2007, 80(4), 370–377.
- Sato, Y.;, et al. In Vitro and in Vivo Antioxidant Properties of Chlorogenic Acid and Caffeic Acid. Int. J. Pharm. 2011, 403(1), 136–138.
- Lin, Y. L.;, et al. Antioxidative Caffeoylquinic Acids and Flavonoids from Hemerocallis Fulva Flowers. J. Agric. Food Chem. 2011, 59(16), 8789–8795.
- Girones-Vilaplana, A.;, et al. Phytochemical Profile of a Blend of Black Chokeberry and Lemon Juice with Cholinesterase Inhibitory Effect and Antioxidant Potential. Food Chem. 2012, 134(4), 2090–2096.
- Perry, N. S.;, et al. In‐Vitro Inhibition of Human Erythrocyte Acetylcholinesterase by Salvia Lavandulaefolia Essential Oil and Constituent Terpenes. J. Pharm. Pharmacol. 2000, 52(7), 895–902.
- Islam, M. N.;, et al. Potent α-glucosidase and Protein Tyrosine Phosphatase 1B Inhibitors from Artemisia Capillaris. Arch. Pharm. Res. 2013, 36(5), 542–552.
- Ghandadi Hossein Abadi, F.;. Evaluation of Tyrosinase Enzyme Inhibitory Effect of Four Medicinal Plant Extract Zataria Multiflora, Nigella Sativa, Tripleurospermum Disiforme and Tanacetum Parthenium as Proposed Lightening Compounds; School of Pharmacy, Kerman University of Medical Sciences: Kerman, Iran, 2015.