ABSTRACT
From an industrial perspective, fast characterization of raw materials provides an important tool for preventing manufacturing problems and contributes to assure the quality of the final products. In this work, several fast, cheap, and simple methods (Fourier transform infrared spectroscopy (FTIR) and various thermoanalytical techniques) were used for screening and characterization of crude and refined palm oils and other palm-derived products. The FTIR spectra allowed for ready distinction between mesocarp-derived products and those obtained from the kernel. The same applied to high-temperature differential scanning calorimetry (DSC) and differential thermal analysis (DTA) thermograms and the low-temperature DSC curves, in which the peak values and the presence or absence of certain peaks also permitted to differentiate among the various oils and fractions. Correspondences of mesocarp-derived oils with olein and kernel-derived oils with stearin were confirmed by both analytical methods and from the crystallization study. The relationship between the triglyceride composition and the FTIR and thermal profiles of the various palm-derived products has potential to be utilized as a facile quality control method in mill plants and laboratories.
Introduction
Fruits and seeds of Elaeis guineensis yield a range of valuable fractions and processed products by refining, fractionation and hydrogenation: viz., crude palm oil, neutralized palm oil, refined bleached and deodorized palm oil, palm olein, palm stearin, palm kernel oil and palm kernel cake or meal. These products represented a global market valued at USD 61.09 billion in 2014, foreseen to grow up to USD 88 billion by 2022. Apart from edible oil, other applications in surfactants, cosmetics, lubricants and biodiesels are also growing, making this crop the largest vegetable oil in the world in terms of produced volume, ahead of soybean oil.[Citation1]
Two main oils can be derived from the oil palm, either from the reddish pulp of the fruit or from the kernel of the same fruit. The former, palm fruit oil (or simply ‘palm oil’), is a higher acidity oil, therefore of a purer quality, and is extracted from the fibrous flesh (mesocarp) of the fruits after they have been hot-squeezed. On the other hand, palm kernel oil is obtained from the dull white endosperm of the kernel (the true seed), which is crushed to produce the oil and the press cake, which is used mostly for animal feed.[Citation2] According to the Foreign Agricultural Service/USDA Office of Global Analysis, the global palm oil and palm kernel oil productions in 2016/2017 were 62.88 and 7.42 million tons, respectively, and 6.23% and 5.79% increases (up to 66.80 and 7.85 million tons, respectively) have been forecast for 2017/2018, according to a May 2017 report.[Citation3]
Palm fruit oil and palm kernel oil give the name to the 16-carbon saturated fatty palmitic acid that both of them contain. Palm kernel oil also has a high content of lauric acid and does not contain trans fatty acids (FAs).[Citation2] The approximate concentrations of FAs in palm oil and in palm kernel oil are summarized in .[Citation4,Citation5]
Palm oil undergoes extensive processing before it reaches the consumer. One of those processes is fractionation, which separates liquid olein from solid stearin by semi-continuous crystallization and separation by a vacuum filter or a membrane filter press. The more saturated triglycerides are gradually concentrated in the solid phase (stearin), leaving behind a more liquid phase (olein).[Citation6] These two main fractions have also been studied herein, albeit further fractionations can be carried out to give such products as palm midfraction and ‘super olein’ or second olein.
In the literature there are studies in which the Fourier transform infrared spectroscopy (FTIR) technique has been used for the analysis of edible oil and fats, such as that by Guillén and Cabo[Citation7] or those by Rohman et al. on refined palm oil on the occasion of adulteration by lard in palm oil[Citation8] or by mixing with virgin olive oil.[Citation9] In fact, Man, Setiowaty, and Van de Voort[Citation10] showed that FTIR could be used to determine the iodine value (often used to guide fractionation processes in order to achieve the desired product quality at optimum operating conditions) of palm oil products with a total analysis time per sample of less than 2 min in substitution of titration methods. Nonetheless, to the best of our knowledge, a more comprehensive study that includes other palm oil products is still missing.
Likewise, thermal analysis has been employed for the characterization of oils from Elaeis guineensis and its products, but only by DSC.[Citation11–Citation16] For instance, Busfield and Proschogo[Citation11,Citation12] studied heating thermograms of palm stearin and its hydrogenated products, and Man and Swe[Citation14] used DSC to study the profile of crude palm oil and its products at low temperatures. However, complete profiles by TG/DTG/DTA and a comparative for the main palm oil products have not been reported so far.
Consequently, the purpose of the work presented herein was to complement and expand aforementioned studies by conducting an assessment of several fast, cheap, and simple methods, namely FTIR, TG/DTG/DTA, and DSC, to be used as a comprehensive guide for characterization purposes and in quality control.
Materials and methods
Samples
High-purity crude palm oil, refined palm oil, crude kernel oil, refined kernel oil, refined palm stearin, and refined palm olein were supplied by Cia Refinadora da Amazônia (Belém, Pará, Brazil), part of Agropalma group. Kernel cake was supplied by the same provider. Palmitic acid (>99%, CAS No. 57-10-3) was purchased from Sigma Aldrich Química S.A. (Tres Cantos, Madrid, Spain). Routine GC–MS analyses of the aforementioned products were conducted to confirm their identities, and results were in agreement with those reported in the literature.[Citation17–Citation19]
Apparatus
The GC–MS analysis was performed on a Varian Star 3400 gas chromatograph (Varian, Walnut Creek, CA, USA) coupled with an ion-trap detector (Varian Saturn 400) equipped with a high-temperature capillary column (DB-5HT, 15 m × 0.25 mm i.d., 0.1 m film thickness; J&W Scientific, Santa Clara, CA, USA). Further details can be found in [Citation20].
The vibrational spectra of the materials in the 400–4000 cm−1 spectral range were measured using a Thermo Scientific (Waltham, MA, USA) Nicolet iS50 FT-IR Spectrometer, equipped with an in-built diamond attenuated total reflection (ATR) system. Spectra were recorded at room temperature with 1 cm−1 spectral resolution, 64 scans.
TG/DTA and high-temperature DSC analyses were conducted with a Perkin–Elmer (Waltham, MA, USA) STA6000 simultaneous thermal analyzer by heating the samples in a slow stream of N2 (20 mL/min) from room temperature up to 500°C, with a heating rate of 20°C/min. Pyris v.11 software was used for data analysis.
The low-temperature DSC experiments were performed in a Perkin–Elmer Pyris 1 power compensation calorimeter. A liquid nitrogen Cryofill cooling unit was used, and helium at a 20 mL·min−1 flux was employed as the purge gas. Perkin–Elmer 30-μL aluminum pans suitable for volatile substances were used, the reference being an empty pan. Temperature calibration was performed using benzophenone, Tfus = 48.05°C;[Citation21] cyclohexane, Tfus = (6.66 ± 0.04)°C, Ttrs = (−86.93 ± 0.02)°C[Citation22] and cyclopentane, Ttrs = (−135.09 ± 0.05)°C; Tfus = (−93.43 ± 0.05)°C.[Citation22] Cyclohexane ΔtrsH = (6713 ± 74) J·mol−1 was used for enthalpy calibration.[Citation22]
Results and discussion
Vibrational characterization
The ATR–FTIR spectra profile for crude palm kernel oil was intermediate between those of palm kernel cake and refined palm kernel oil ()). In the same way, there was a good similarity between the spectra of crude palm oil and olein ()) on the one hand, and between refined kernel oil and stearin ()) on the other hand.
Figure 1. ATR-FTIR spectra of (a) palm kernel products and stearin, (b) palm fruit products and olein.
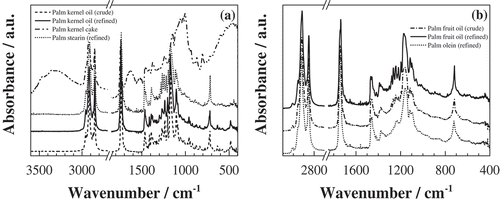
The ATR–FTIR spectra displayed the characteristic peaks (see ) of some functional groups common to the ester samples.[Citation7,Citation23–Citation28] The characteristic weak band at 3005 cm−1 (which only appeared in palm pulp oils and which was absent in kernel-derived products) is due to asymmetric olefinic C-H stretching vibration of the carbon–carbon double bond. The strong absorption band at 2921–2914 cm−1 (asymmetric aliphatic C-H stretching) shown by all samples also has a lipid origin, as it occurs for the absorption band at 2852–2847 cm−1 (symmetric aliphatic C-H stretching). A fourth lipid characteristic band at 1740 cm−1 is due to the stretching vibration of the triglyceride ester carbonyl (-C-C = O) group.[Citation29]
Table 2. Main absorption bands in the ATR–FTIR spectra of crude and refined oils and other products obtained from Elaeis guineensis (all wavenumbers are expressed in cm−1).
The iodine value (IV) is used as a parameter in process control, as well as a quality parameter in traded palm oil products. FTIR absorbance ratios A(3006 cm−1)/A(2919 cm−1), A(3006 cm−1)/A(2851 cm−1), and A(3006 cm−1)/A(1742 cm−1)[Citation26] were considered to measure the IVs for crude and refined palm pulp oil, olein, and stearin. The average of these ratios for each of the samples (0.13, 0.09, 0.09, and 0.07, respectively) revealed that the IV for these oils ranges between 30 and 55 mg I2/100 g, as expected from their low unsaturation, and are in good agreement with the standard reference values proposed in [Citation30]. Since the oxidative stability is dependent on the IV (the higher the IV, the less stable the oil is), a high stability could be expected.
The band at 1467–1454 cm−1 is due to ›CH2 scissoring deformation, δ(CH2). The band at 1279 cm−1 exhibited by refined palm pulp oil should be assigned to in plane = C-H deformation in an unconjugated cis double bond.[Citation27] The peak between 1172 and 1145 cm−1 (with its shoulders at 1115–1104 and 1243–1236 cm−1), which appeared in the spectra of all the samples, is a fingerprint of long-chain fatty acid methyl ester.[Citation16] The bands at 1115 and 1097 cm−1 come from the stretching vibration of ether linkage in triacylglycerols[Citation7] and their peak heights have been shown to be inversely related to the proportion of saturated acyl groups and oleic acid groups, respectively.[Citation28] The band at 960 cm−1 was present in kernel oils but not listed in the spectra of the oils extracted from the mesocarp of the palm fruits. Peaks around 870 cm−1 and 804 cm−1[Citation23] are due to the presence of epoxy group in the palm kernel cake. Finally, the bands at 720 cm−1 and 667 cm−1 can be assigned to = C-H planar bending and C-O out of plane bending, respectively.[Citation31]
Thermal behavior
TG/DTA and high-temperature DSC heating curves accurately expressed the thermal behavior of the various products, and the profiles may also be used as guidelines for characterization ( and , –). The first thermal effect was due to the melting of high melting point triacylglycerols, which was sensitized with a sharp endotherm for all the samples. The correspondence between DTA and DSC effects was excellent for our registers, and a reasonably good agreement was also attained in comparison to the literature: Nassu and Guaraldo Gonçalves[Citation32] reported melting points at 41.3°C for refined palm pulp oil, at 30.6°C for refined kernel oil, at 27.9°C for olein, and at 54.5°C for stearin (very close to the ones reported herein), while according to Che Man et al.[Citation15] melting would occur at 34.5°C for crude palm pulp oil, at 36.3°C for refined palm pulp oil, and at 57.6°C for stearin. Tarmizi et al.,[Citation30] from data obtained from 32 laboratories, indicated that the slip melting point for standard reference stearin would be 53.4°C (so the error would be <2%).
Table 3. High-temperature DSC thermal effects peaks (°C) for crude and refined oils from Elaeis guineensis. Palm kernel cake and palmitic acid were included for comparison purposes. Means (±SD) of triplicate analyses.
Table 4. DTA thermal effects peaks (°C) for crude and refined oils from Elaeis guineensis. Palm kernel cake and palmitic acid were included for comparison purposes. Means (±SD) of triplicate analyses.
Figure 2. TG/DTG and high-temperature DSC curves for crude (top) and refined (bottom) palm kernel oils.
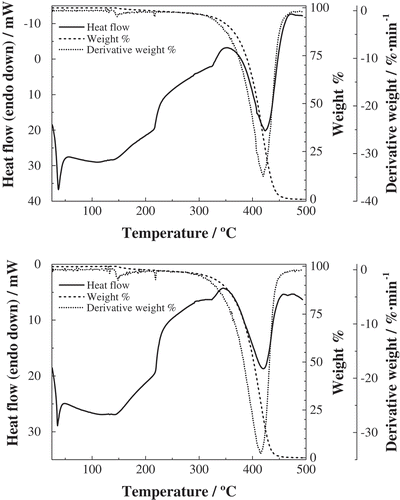
Figure 3. TG/DTG and high-temperature DSC curves for crude (top) and refined (bottom) palm pulp oils.
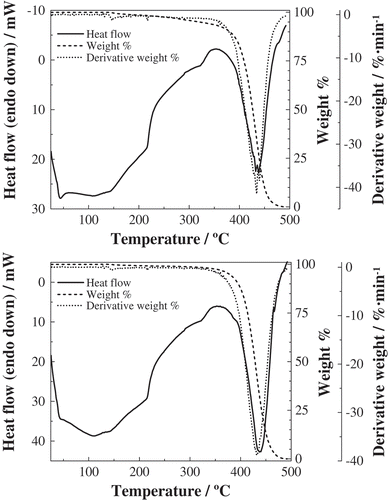
Aforementioned temperatures were also in agreement with those expected on the basis of the triglyceride composition of the different Elaeis guineensis products and the temperature of the peaks of triglyceride standards on DSC curves (). Palm oils (both pulp and kernel ones) and olein—which consists mainly of monosaturated (POO) and disaturated (POP and POS) triglycerides—showed melting points below 45°C, while stearin—which consists mainly of disaturated (PPO) and trisaturated (PPP) triglycerides—showed the corresponding endothermic effect at a higher temperature (above 50°C), in analogy with palmitic acid.
Table 5. Temperature of the peaks of triglyceride standard on DSC curves.[Citation15]
Whereas the peaks at around 216°C and 323°C were shared by all the oils and fractions, the temperatures of peaks at around 40°C and 430°C allowed to readily distinguish the origin of the products: (42.8 ± 0.5)°C and (439 ± 1)°C for mesocarp-derived oils (and olein) vs. (36 ± 1)°C and (422 ± 1)°C for kernel-derived products (and stearin). This result confirms the tentative grouping obtained from FTIR results.
In connection with the last effect in the DSC and DTA curves, attributable to pyrolysis, it should be noted that it corresponded to a virtually entire mass loss. Such mass loss was observable from the TG curves (dashed lines) and the maximum decomposition rate was expressed by the DTG (dotted lines) peaks (summarized in ). The temperatures of these peaks were in good agreement with those shown in and for DSC and DTA, respectively, albeit with a ca. 5°C shift to higher temperatures.
Table 6. TG curves: decomposition peaks for crude and refined oils from Elaeis guineensis. Maximum decomposition rate temperatures (in °C) were estimated from the DTG peaks for the pyrolysis of the respective products. Means (±SD) of triplicate analyses.
Crystallization behavior
The crystallization processes of palm oils are observed on the cooling DSC curves after a first heating from room temperature to 60°C (). The effects, summarized in , are all of exothermic nature and some of them correspond (with a temperature shift) to the endothermic effects attributed to softening (1–8°C) and melting (ca. 40°C) in the heating cycle.
Table 7. Thermal effects of the E. guineensis products in the low-temperature DSC thermograms. All temperatures are in °C. Means (±SD) of triplicate analyses.
Figure 6. Low-temperature DSC curves for various palm oil products: (a) crude palm pulp oil; (b) refined palm pulp oil; (c) crude palm kernel oil; (d) refined palm kernel oil; (e) palm olein; and (f) palm stearin). First heating cycle is shown as a blue solid line, the cooling cycle is shown in black, and the second heating cycle in red.
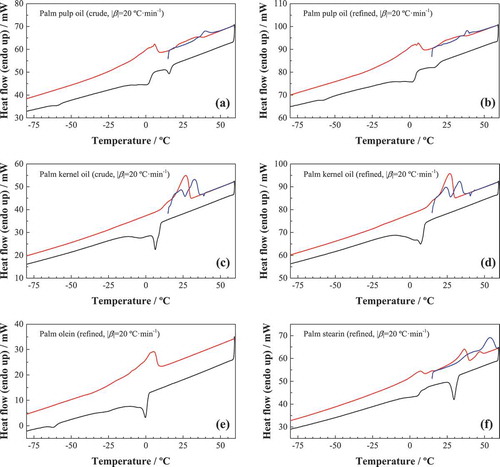
Exotherms at temperatures slightly below zero or above zero can be put in relation with softening and melting endothermic effects in the heating cycles, for instance: the exotherms at −3.3°C and 6.3°C in the cooling cycle for crude kernel oil would be associated to the softening point at 23.5°C and the melting point at 32.7°C,[Citation32] respectively, with a ca. 26°C shift towards lower temperatures. The exothermic peaks at the lowest temperatures (−59.5° in crude palm oil, −58.3°C in refined palm pulp oil, and −62.3°C in olein) can be ascribed to triunsaturated triglycerides (OOO): the triunsaturated (OOO) standard shows its characteristic peak at −52.7°C.[Citation15] This peak was absent in the kernel-derived oils and in stearin, confirming the differential behavior discussed above. The absence of this peak also implies that the fractionation that produced stearin was optimal.
It is worth noting that the position of the exotherms is dependent on the cooling rate, as indicated by Zhang et al.[Citation16] in their study on olein and stearin: as the cooling rate is increased they arrive at lower temperatures and get broadened. At a high cooling rate (20°C·min−1 in this study) higher melting triacylglycerols will be rapidly undercooled and initially crystallized, developing a solid within the liquid phase, and the polymer chains that come into crystalline lattice require a certain “relaxation” time, resulting in a “lag period” compared to the cooling process, which increases as the cooling rate is increased. In addition, this is accompanied by a rapid increase in viscosity, thus heat transfer and mass transfer may be limited. The lower mobility of molecular chains would prevent rearrangement of the crystal, thus forming unstable crystals.
Conclusion
ATR–FTIR spectroscopy and thermoanalytical techniques were used to characterize various E. guineensis oils and fractions: crude and refined palm kernel oils, crude and refined palm pulp oils, palm olein, palm stearin, and palm kernel cake. ATR–FTIR allowed a facile differentiation of the origin (fruit pulp products vs. kernel products): the band at 3006 cm−1 that appeared in the former was absent in later, while the band at 960 cm−1 was characteristic of kernel oils. Likewise, valuable information on the degree of unsaturation (iodine values) and the oxidation stability degree for crude and refined pulp oils, olein, and stearin was retrieved from the bands at 3006, 2919, 2851, and 1742 cm−1. High-temperature DTA or DSC thermograms also allowed to distinguish the origin of the products through the peaks at around 40°C and 430°C ((36 ± 1)°C and (422 ± 1)°C, present only in kernel-derived products, and those at (42.8 ± 0.5)°C and (439 ± 1)°C, associated with mesocarp oils). Further, low-temperature DSCs cooling curves enabled classification on the basis of the presence of an exothermic effect at ca. −60°C, only present in palm pulp oils. Both ATR–FTIR and thermal analyses link palm pulp oils and olein on the one hand, and kernel oil and stearin on the other hand. Thus, these techniques can be deemed as low-cost, fast and facile screening methods, complementary to the routine GC/MS quality control tests. The work presented herein represents the first comprehensive guide to the use of these methods for characterization purposes and in quality control.
Acknowledgments
The authors would like to thank the financial support given by the Fundação para a Ciência e a Tecnologia (FCT), the Portuguese Agency for Scientific Research, under project UID/QUI/UI0313/2013, and the COMPETE Programme (Operational Programme for Competitiveness). Access to the TAIL-UC facility, funded under QREN-Mais Centro Project ICT_2009_02_012_1890 is also gratefully acknowledged. The authors have declared no conflict of interest.
References
- Grand View Research. Palm Oil Market Analysis by Derivative (Crude Palm Oil, Palm Kernel Oil, Palm Kernel Cake), by Application (Edible Oil, Cosmetics, Bio-Diesel, Lubricants, Surfactants) and Segment Forecasts to 2022; Grand View Research Inc.: San Francisco, CA, USA, 2016; 100 pp.
- Corley, R. H. V.; Tinker, P. B. The Oil Palm; John Wiley & Sons: Hoboken, NJ, USA, 2016, 680 pp.
- USDA/FAS. Oilseeds: World Markets and Trade (May 2017); United States Department of Agriculture/Foreign Agricultural Service: Washington DC, USA, 2017; 49 pp.
- Ang, C. Y. W.; Liu, K.; Huang, Y.-W. Asian Foods: Science & Technology; Technomic Pub. Co.: Lancaster, PA, USA, 1999, 546 pp.
- Obahiagbon, F. I.;. A Review: Aspects of the African Oil Palm (Elaeis Guineensis Jacq.) And the Implications of Its Bioactives in Human Health. Am. J. Biochem. Mol. Biol. 2012, 2, 106–119.
- Lai, O.-M.; Tan, C.-P.; Akoh, C. C. Palm Oil : Production, Processing, Characterization, and Uses; AOCS Press: Urbana, IL, USA, 2012, 838 pp.
- Guillén, M. D.; Cabo, N. Infrared Spectroscopy in the Study of Edible Oils and Fats. J. Sci. Food Agric. 1997, 75, 1–11.
- Rohman, A.; Kuwat, T.; Retno, S.; Sismindari,; Yuny, E.; Tridjoko, W. Fourier Transform Infrared Spectroscopy Applied for Rapid Analysis of Lard in Palm Oil. Int. Food. Res. J. 2012, 19, 1161–1165.
- Rohman, A.; Man, Y. B. C. Fourier Transform Infrared (FTIR) Spectroscopy for Analysis of Extra Virgin Olive Oil Adulterated with Palm Oil. Food Res. Int. 2010, 43, 886–892.
- Man, Y. C.; Setiowaty, G.; Van de Voort, F. Determination of Iodine Value of Palm Oil by Fourier Transform Infrared Spectroscopy. J. Am. Oil Chem. Soc. 1999, 76, 693–699.
- Busfield, W. K.; Proschogo, P. N. Thermal Analysis of Palm Stearine by DSC. J. Am. Oil Chem. Soc. 1990, 67, 171–175.
- Busfield, W. K.; Proschogo, P. N. Hydrogenation of Palm Stearine: Changes in Chemical Composition and Thermal Properties. J. Am. Oil Chem. Soc. 1990, 67, 176–181.
- Yap, P.; De Man, J. M.; De Man, L. Polymorphism of Palm Oil and Palm Oil Products. J. Am. Oil Chem. Soc. 1989, 66, 693–697.
- Man, Y. B. C.; Swe, P. Z. Thermal Analysis of Failed-Batch Palm Oil by Differential Scanning Calorimetry. J. Am. Oil Chem. Soc. 1995, 72, 1529–1532.
- Che Man, Y. B.; Haryati, T.; Ghazali, H. M.; Asbi, B. A. Composition and Thermal Profile of Crude Palm Oil and Its Products. J. Am. Oil Chem. Soc. 1999, 76, 237–242.
- Zhang, X.; Li, L.; Xie, H.; Liang, Z.; Su, J.; Liu, G.; Li, B. Comparative Analysis of Thermal Behavior, Isothermal Crystallization Kinetics and Polymorphism of Palm Oil Fractions. Molecules 2013, 18, 1036–1052.
- Berger, K. G.;. Food Uses of Palm Oil; Malaysian Palm Oil Promotion Council: Kuala Lumpur, Malaysia, 1996, 25 pp.
- Sambanthamurthi, R.; Oo, K.-C.; Parman, S.-H. Factors Affecting Lipase Activity in Elaeis Guineensis Mesocarp. Plant Physiol. Biochem. 1995, 33, 353–359.
- Tang, T. S.;. Composition and Properties of Palm Oil Products. In Advances in Oil Palm Research, Vol. 2; MPOB, Ed.; Malaysian Palm Oil Board: Kuala Lumpur, Malaysia, 2000; pp. 845–894.
- Del Río, J. C.; Evaristo, A. B.; Marques, G.; Martín-Ramos, P.; Martín-Gil, J.; Gutiérrez, A. Chemical Composition and Thermal Behavior of the Pulp and Kernel Oils from Macauba Palm (Acrocomia Aculeata) Fruit. Ind. Crop. Prod. 2016, 84, 294–304.
- Chirico, R. D.; Knipmeyer, S. E.; Steele, W. V. Heat Capacities, Enthalpy Increments, and Derived Thermodynamic Functions for Benzophenone between the Temperatures 5K and 440K. J. Chem. Thermodyn. 2002, 34, 1885–1895.
- Sabbah, R.; Xu-Wu, A.; Chickos, J. S.; Leitão, M. L. P.; Roux, M. V.; Torres, L. A. Reference Materials for Calorimetry and Differential Thermal Analysis. Thermochim. Acta 1999, 331, 93–204.
- Silverstein, R. M.; Webster, F. X.; Kiemle, D. J. Spectrometric Identification of Organic Compounds; John Wiley & Sons: Hoboken, NJ, USA, 2005, 502 pp.
- Abdelmalik, A. A.; Abbott, A. P.; Fothergill, J. C.; Dodd, S.; Harris, R. C. Synthesis of a Base-Stock for Electrical Insulating Fluid Based on Palm Kernel Oil. Ind. Crop. Prod. 2011, 33, 532–536.
- Yoshida, S.; Yoshida, H. Nondestructive Analyses of Unsaturated Fatty Acid Species in Dietary Oils by Attenuated Total Reflectance with Fourier Transform IR Spectroscopy. Biopolymers 2003, 70, 604–613.
- El-Bahy, G. M. S.;. FTIR and Raman Spectroscopic Study of Fenugreek (Trigonella Foenum Graecum L.) Seeds. J. Appl. Spectrosc. 2005, 72, 111–116.
- Sadeghi-Jorabchi, H.; Hendra, P. J.; Wilson, R. H.; Belton, P. S. Determination of the Total Unsaturation in Oils and Margarines by Fourier Transform Raman Spectroscopy. J. Am. Oil Chem. Soc. 1990, 67, 483–486.
- Jaswir, I.; Mirghani, M. E. S.; Hassan, T. H.; Said, M. Z. M. Determination of Lard in Mixture of Body Fats of Mutton and Cow by Fourier Transform Infrared Spectroscopy. J. Oleo Sci. 2003, 52, 633–638.
- Nordin, N. A.; Sulaiman, O.; Hashim, R.; Mohamad Kassim, M. H. Characterization of Different Parts of Oil Palm Fronds (Elaeis Guineensis) and Its Properties. Int. J. Adv. Sci, Eng. Inf. Technol. 2016, 6, 74–76.
- Tarmizi, A. H. A.; Lin, S. W.; Kuntom, A. Palm-Based Standard Reference Materials for Iodine Value and Slip Melting Point. Anal. Chem. Insights 2008, 3, 127–133.
- Rosli, N. S.; Harun, S.; Jahim, J. M.; Othaman, R. Chemical and Physical Characterization of Oil Palm Empty Fruit Bunch. Mjas 2017, 21, 188–196.
- Nassu, R. T.; Guaraldo Gonçalves, L. A. Determination of Melting Point of Vegetable Oils and Fats by Differential Scanning Calorimetry (DSC) Technique. Grasas Aceites 1999, 50, 16–21.