ABSTRACT
In this study, the effects of low-temperature long-time (LTLT) processing on the quality of Patinopecten yessoensis adductor muscle (PYAM) were investigated at 55°C. The texture of processed PYAM was characterized by textural profile analysis (TPA), and significant increases of cook loss, hardness, and shear force with time during LTLT processing were observed. The degradation of structural proteins was analyzed by sodium dodecyl sulfate polyacrylamide gel electrophoresis (SDS-PAGE), and fragments with molecular weights of 208 kDa (myosin heavy chain, MHC), 97 kDa (paramyosin) and 35–40 kDa, respectively, were among the main products. Chemical characterization revealed elevated levels of activity in cathepsin L and caspase-3 and oxidation of proteins and lipids. Electron spin resonance spin trapping indicated reactive oxygen species (ROS) production in the PYAM during LTLT processing. Based on these results, it is proposed that the sequence of events in PYAM during LTLT processing includes ROS→ endogenous enzyme (involving caspase-3 and cathepsin L) activation →protein degradation→quality changes (texture and color). This revelation helps to further our understanding of the LTLT processing of PYAM, which would lead to better quality control for PYAM products.
Introduction
Scallop Patinopecten yessoensis (P. yessoensis) is one of the most important scallop species in coastal fisheries. The total scallop output of 2015 reached more than 1.78 million tons in China.[Citation1] On a weight basis, scallops are composed of shell (52%), adductor muscle (13%), mantle lobe (9%), and gonad (ovary and testis, 3–9%). [Citation2] The main edible part of P. yessoensis is the adductor muscle, which has high nutritional value and is traditionally regarded as a delicacy.[Citation3] High-temperature processing of P. yessoensis adductor muscle (PYAM) often yield products with high hardness, dark yellow color, and tough texture that are viewed negatively by consumers. As an alternative, low-temperature (LT) processing may be utilized to process PYAM. LT processing has been developed to improve the eating quality of beef and pork, such as consistency, texture, and color of the meats.[Citation4] It is reasonable to believe that LT processing may improve the quality of PYAM products as well. However, before LT can be established as a legitimate method for PYAM processing, its mechanism of affecting PYAM needs to be further clarified.
It has been reported that heat treatments may cause changes in hardness, taste, flavor, and nutritional value in animal foods.[Citation5] Previous studies found that endogenous enzymes could influence the oxidation of meat proteins and lipids; subsequently, they could greatly affect the hardness, the taste, and the flavor as well as the nutritional value of the meat.[Citation6] Endogenous enzymes of the cathepsin family and the caspase family have become a focus of research in recent years.[Citation7] Cathepsin L and S were shown to affect meat tenderness and elasticity in Italian white pig[Citation8]; Activation of caspase-3 was correlated to the degradation of chicken myofibrillar proteins and myofibrillar structure.[Citation9] Ultrasonic induced caspase-3 expression was suggested to promote myofibrillar degradation in slaughtered and cooked chicken.[Citation10] Furthermore, effects of lipid oxide and protein oxide on meat quality have been reported in beef,[Citation11] pork,[Citation12–Citation14] and fish.[Citation4,Citation15] Oxidative stresses are resulted from the accumulation of reactive oxygen species (ROS).[Citation16] ROS in turn, through the modification of cytoskeleton protein peptide and amino acid side chains to change protein cross-linking or degradation, determines the meat texture, flavor, and color characteristics[Citation17,Citation18] . ROS directly induced by cations, or indirectly induced through oxidative stresses, could lead to protein oxidation.[Citation19]
We believe that ROS plays a significant role in regulating endogenous enzymes and determining the meat quality such as texture and tenderness during the low-temperature long-time (LTLT) processing of PYAM. Therefore, the objective of this study was to characterize the ROS production, the activation of downstream caspase-3 and cathepsin L, and the formation of oxidants in PYAM during the LTLT processing.
Materials and methods
Materials and chemicals
P. yessoensis were purchased from a local aquatic market in Dalian, Liaoning province (China). Malondialdehyde (MDA) assay kit and total sulfhydryl assay kit were purchased from Nanjing Jiancheng Bioengineering Institute (Nanjing, China). All other chemicals were obtained from Sangon Biotech Co., Ltd. (Shanghai, China) or Sigma-Aldrich Chemical Co. (St Louis, Mo, USA). All of them were at least analytical grade and used without further purification.
LTLT processing and cook loss evaluation
PYAM cubes (about 1.5 cm × 1.5 cm × 1.5 cm) were dissected on ice from P. yessoensis. The samples were vacuum packed and heated in water baths (i.e., LTLT processing) at 55°C for 1/6, 1/2, 1, 2, 4, 6, 12, 18, 24, 27, 30, and 32 h, respectively. All cooked PYAM cubes as well as raw ones as controls were quickly frozen and stored at −80°C, until to be used for biochemical measurements outlined below. The LTLT process was arrested by soaking the cooked samples in icy water for 10 min.[Citation20] Cook loss was determined by calculating the weight lost during the LTLT process[Citation21] and expressed as a percentage of the initial weight.
Instrumental textural analysis
After the LTLT processing, the PYAM cubes were trimmed into cylindrical shape with a height of 13.0 mm and a diameter of 12.7 mm and assayed by a TA-XT2i texture analyzer (Stable Micro Systems, Surrey, UK). P/100 probe was used to perform textural profile analysis (TPA), and the main parameter investigated was hardness. Each sample was subjected to two cycles of compression analysis with a compression speed of 1.0 mm/s and a compression level of 60%, with 5 s relaxation time between the cycles. Shear force was defined as mean maximum force required to shear through the samples, and was determined using Extended Craft Knife Blade (ECB) probe, with a test speed of 1 mm/s. Mean values from 10 repetitions were obtained for each LTLT treatment.
Color analysis
Color (L*, a*, and b* values) for each PYAM sample was measured 10 times on the fresh cut surface of each sample with a UltraScan PRO colorimeter (Hunter Lab, USA). Mean values from 10 repetitions were calculated for each LTLT treatment.
SDS-PAGE gel electrophoresis
Samples were lysed on ice in a lysis buffer (20 mM Tris–HCl (pH 7.4), 1% Triton X-100, 150 mM NaCl, 2 mM ethylenediaminetetraacetic acid ethylene glycol tetraacetic acid (EDTA), 2 mM EGTA, 1 mM Na3VO4, 10 mM NaF,10 mM DTT, 0.1% SDS, 10 μg/mL leupeptin, 10 μg/mL aprotinin, 1 mM phenylmethylsulfonyl fluorid (PMSF)) at 4°C by 1:3 (w/v). Lysates were centrifuged at 12,000 × g for 15 min, at 4°C and supernatants were collected and mixed with SDS-PAGE sample buffer (0.25 M Tris–HCl, pH 7.4, 5% SDS, 5% mercaptoethanol, 8 M urea) by 1:1 (v/v) and then boiled for 5 min. 5 μL samples were loaded onto the polyacrylamide gel made of 10% resolving gel and 5% polyacrylamide stacking gel.
Cathepsin L activity assay
Cathepsin L activity was measured following reported fluorescence method.[Citation22] Protein concentrations of sample supernatants used in the activity assays were determined using a Bradford assay.[Citation23] Results were expressed in U/mg protein where 1 unit (U) was defined as the increased one fluorescence value of sample compared to blank during the reaction at 37°C.
Caspase-3 activity assay
Caspase-3 activity was determined by colorimetric method.[Citation22,Citation24] Results were expressed in U/mg protein where 1 unit (U) was defined as the absorbance value increased by 1 under the reaction.
Protein oxidation assay
Protein carbonyl groups and sulfhydryl were measured to evaluate the protein oxidation level. Carbonyl groups were estimated based on the 2,4-dinitrophenylhydrazine (DNPH) colorimetric method[Citation25] with slight modification. Five PYAM samples (about 0.9 g in total mass) were homogenized in 4.5 mL Tris buffer (pH 7.4, 50 mM) containing 0.01% butylated hydroxy toluene (BHT) and 50 mM, 1 mM EDTA. After centrifugation (12,000 × g, 10 min), the supernatant was incubated with 500 µL DNPH dissolved in 2.5 M HCl, in the dark for 1 h. For each sample, a blank incubated in 400 µL 2.5 M HCl and without DNPH was run in parallel. The samples were precipitated with 20% trichloroacetic acid (TCA) (v/v) and the pellets were washed three times with 300 µL ethanol/ethyl acetate 1:1 (v/v). The pellet was redissolved in 2 mL 6 M guanidine hydrochloride at 37°C for 15 min[Citation11] . The carbonyl content was calculated using the absorbance measurements at 370 nm and expressed as nmol DNPH/mg protein.
Total sulfhydryl content was determined by using commercially available kits. The PYAM was homogenized in 0.9% NaCl solution at 4°C by 1:9 (w/v). The homogenates were centrifuged at 4000 rpm for 20 min at 4°C. The supernatants were collected and frozen at −30°C. All procedures completely complied with the manufacture’s instructions. Sulfhydryl was measured at a wavelength of 405 nm by reacting samples with 5,5ˊ-Dithiobis-(2-nitrobenzoic acid) DTNB to produce a yellow compound. Values of sulfhydryl level were expressed as nmol sulfhydryl/mg protein.
Lipid oxidation assay
The content of MDA was determined by using commercially available kits. Samples were homogenized in ice cold phosphate buffer (10 mM sodium phosphate at pH 7.0, 2% SDS, 0.01% BHT) at 4°C by 3:20 (w/v). The homogenates were centrifuged at 12,000 × g for 10 min at 4°C. The supernatants were collected and frozen at −30°C. MDA content was measured at a wavelength of 532 nm by reacting samples with thiobarbituric acid to form a stable chromophoric product.[Citation26]Values were expressed as nmol MDA/mg protein extracted.
Electron spin resonance spectroscopy
A modified version of an electron spin resonance (ESR)-based assay[Citation27] was used for evaluation of the ROS production in the PYAM. Fresh PYAM was homogenized with 50 mM phosphate buffer (pH 5.7) containing of 40 mM water-soluble spin trap α-(4-pyridyl n-oxide)-n-tert-butylnitrone (POBN, TCI). The emulsion with POBN was then treated at 55°C and at selected times, and then the samples were centrifuged at 12,000 × g for 10 min at 4°C. The supernatants were transferred immediately into capillaries, and the capillaries were placed in the cavity of an ESR spectrometer A200 (Bruker, Karisruhe, Germany). ESR conditions were as follows: sweep width 50 Gauss, microwave power of 1.99 mW, modulation amplitude 1.0 Gauss, modulation frequency 100 kHz, time constant 5242.88 ms, conversion time 360 ms, and number of scans per sample was three. The trapping of ROS in samples by POBN gave rise to adduct with six-line ESR spectra (triplets of doublets). The signal height of the first line of the center field doublet was used as a measure of the relative concentration of ROS adducts formed in samples at a given time.
Statistical analysis
Statistical calculations were subjected to the statistical package SPSS 16.0 (SPSS Inc., 2001, Chicago, IL,USA) for One-way ANOVAs and p values <0.05 were considered significant.
Results
Changes in PYAM quality attributes during LTLT processing
In order to determine the effects of LTLT processing on the quality of PYAM, four attributes (i.e., cook loss, hardness, shearing force, and color) were investigated. Cook loss () of the PYMA increased with processing time from 6 to 32 h and reached a peak value of 33.29 ± 8.37% at 32 h, similar to the cook loss value of PYAM being boiled at 98°C for 14.5 h.[Citation28] Noticeably, no significant change was observed in cook loss between 6 and 27 h. The effect of LTLT treatment on hardness in the PYAM is shown in . The hardness value significantly increased from 2 to 18 h and reached the maximum value of 1670.19 ± 181.48 g at 18 h, more than two-fold than that of the raw sample, and then decreased from 18 to 32 h.
Figure 1. Changes in the quality of Patinopecten yessoensis adductor muscle (PYAM) during LTLT processing at 55°C. (A) Cooking loss;(B) hardness; (C) shear force; (D) L* (lightness); (E) a* (redness); and (F) b* (yellowness). Data are reported as mean ± SD based on 10 replicates. Different letters indicated significant differences (p < 0. 05).
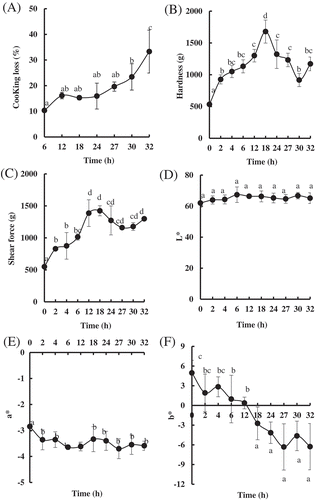
Similarly, the shear force went up to the maximum value of 1423.29 ± 78.88 g after 18 h of processing time, with >100% increase over that of the raw sample, and almost stayed stable from 18 to 32 h ().
, E, and F showed the effects of LTLT treatment on color in PYAM. L* values (lightness) were not affected by increasing cooking time, but a* values (redness) and b* (yellowness) were affected nonetheless. The a* values of the processed PYMA decreased over 15% from that of the raw sample, while the b* values decreased with cooking time and dropped down to the minimum value of −6.28 ± 3.53 from 4.95 ± 1.91. These results indicated that the qualities of PYMA were affected by the LTLT processing, to various extents.
Changes of protein degradation and cathepsin L and caspase-3 activities during LTLT processing
Because thermal treatment could lead to protein degradation, SDS-PAGE was conducted to detect the changes in protein content/composition in PYAM during LTLT processing. The results are shown in . The protein compositional pattern of the raw sample was in partial agreement with Goetz and Whisman,[Citation29] with higher protein content being observed. With extended LTLT treatments, extensive degradation in the PYAM proteins was observed, evidenced by the gradual reduction in densities of protein bands with molecular weights of 208 kDa (myosin heavy chain, MHC), 97 kDa (paramyosin), and 35–40 kDa, respectively, especially from 18 to 32 h. However, no significant difference in actin bands (43 kDa) was observed with/without LTLT.
Figure 2. Changes in protein degradation and endogenous enzyme activities in Patinopecten yessoensis adductor muscle (PYAM) during LTLT processing at 55°C. (A) SDS–PAGE photographs of protein degradation. 5 μL sample was loaded in 10% (w/v) SDS–PAGE gel; HM, high MARK; LM, low MARK; (B) cathepsin L activity measured by a fluorospectrophotometer; (C) caspase-3 activity measured by a spectrophotometry. Data are reported as mean ± SD based on three replicates. Different letters indicated significant differences (p < 0. 05).
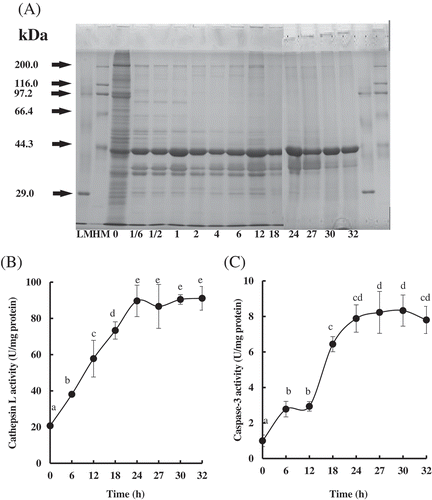
It has been reported that cathepsins play key roles in the proteolysis of marine organisms like salmon[Citation30] and sea cucumber.[Citation31] In the PYAM, the cathepsin L activity increased rapidly during LTLT from 6 to 24 h, and leveled off from 24 to 32 h (). The increase of cathepsin L activity was more than threefold in treated samples. It is likely that cathepsin L is released from the lysosome and then activated when the PYAM was subjected to LTLT processing.
As activated caspase-3 is related to protein degradation,[Citation9] the activity of caspase-3 was also determined in this study. As shown in , the caspase-3 activity increased significantly with the increasing processing time and reached the maximum value of 8.33 ± 0.88 U/mg protein after 30 h. The increasing caspase-3 activity suggests elevated apoptosis level in the PYAM during LTLT processing, because the activation of caspase-3 is a critical indicator of apoptosis. Caspase-3 could also be involved in protein degradation of PYAM during the LTLT processing.
Oxidant production during LTLT processing
In order to further explore the underline mechanism for the change of the quality in the PYAM, the oxides and the peroxides produced during LTLT processing were determined. The level of protein oxidation was quantified by measuring the contents of carbonyl and sulfhydryl. As shown in , the carbonyl content in the PYAM increased significantly from 0.28 ± 0.18 to 2.15 ± 0.68 nmol/mg protein, while the sulfhydryl content decreased by 29.60% after LTLT treatment for 27 h (). Besides protein oxidation, lipid peroxidation was also monitored. The level of lipid oxidation was quantified by MDA. As shown in , no significant difference of the MDA content was observed before 12 h. However, the MDA content increased significantly (over onefold) after 27 h, compared to that of the unheated raw samples.
Figure 3. Changes in oxidation in Patinopecten yessoensis adductor muscle (PYAM) during LTLT processing at 55°C. (A) Carbonyl content; (B) total sulfhydryl group content; (C) MDA content. They were detected by a spectrophotometry. Data are reported as mean ± SD based on three replicates. Different letters indicated significant differences (p < 0. 05).
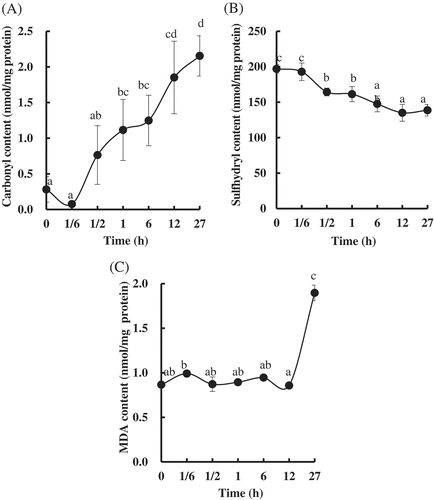
ROS formation during LTLT processing
The ROS content of the PYAM during LTLT processing was evaluated by ESR spin trapping. As shown in , the ESR spectra of the observed spin adducts had hyperfine coupling constants (aN = 15.8G, aH = 2.6G), which are typical values for spin adducts formed by addition of carbon-centered radicals to POBN.[Citation32] It indicated that ROS centered by carbon were produced in the processed PYAM. The ROS level improved lightly before 6 h and went up significantly after 12 h. The spin adducts reached the highest value of 1.9 × 106 at 32 h. Apparently, the ROS production started slowly and accelerated after extended heating. Presumably, the antioxidant enzymes in PYAM resists the ROS production in the initial stage (before 6 h), but their effect is not strong enough to clear out all the ROS. Furthermore, their activities decline as the accumulation of ROS goes up.
Figure 4. Changes in production of free radicals in Patinopecten yessoensis adductor muscle (PYAM) during LTLT processing at 55°C. (A) Development in electron spin resonance (ESR) spectroscopy absorption of the meat slurries with 40 mM α-(4-pyridyl-1-oxide)-N-tert-butylnitrone (POBN) added. (B) ESR spectra of the meat slurries with 40 mM POBN. (a) 0 h, (b) 1/6 h, (c) 1 h, (d) 2 h, (e) 4 h, (f) 6 h, (g) 12 h, (h) 18 h, (i) 24 h, (j) 27 h, and (k) 32h. Different letters mean significant (p < 0.05).
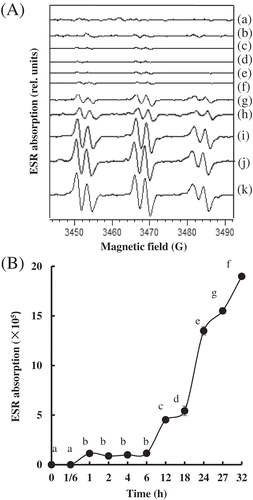
Scheme of signal cascade during LTLT cooking in the PYAM
shows a proposed signal pathway during the LTLT processing of PYAM at 55°C to illustrate cellular events. In summary, ROS are produced to induce protein oxidation and lipid peroxidation, which subsequently activate the downstream proteolytic enzymes such as cathpesin L and caspase-3. The proteolytic enzymes accelerate protein degradation, which in turn leads to the changes in qualities reflected in cook loss, texture, and color of the PYAM.
Discussion
The sous-vide cooking technique has been applied to varieties of meat products, like beef,[Citation33,Citation34] pork,[Citation35] lamb,[Citation36] and chicken.[Citation37] Based on these studies, the temperature and holding time are critical to the products quality and shelf-life. In our research, 55°C was chosen since it provided moderate heating, yet enough for pathogen destruction.[Citation38] The current study revealed biochemical events that underline the factors that affect the quality of processed PYAM during LTLT processing, which include ROS formation, protein oxidation, lipid peroxidation, endogenous enzyme (caspase-3, cathepsin L) activation, and protein degradation. The cook loss gradually increased with heating time (), which was consistent with the increasing cook loss of skeletal muscle in pork[Citation39]and beef[Citation33] by LTLT processing. Meanwhile, Mortensen et al.[Citation40] reported that juiciness of beef could be classified into different groups by trained panelists when the beef encountered sous-vide cooking at 56, 58, and 60°C for 3, 6, 9, and 12 h, respectively. They also reported similar trends in tenderness evaluation. In this study, hardness and shear force of the PYAM were observed to increase constantly with heating time until 18 h (,C). A similar phenomenon that hardness increased at early stage and decreased with storage time extension was observed in instant scallop during storage at 20–40°C.[Citation20] Changes in meat juiciness and tenderness during cooking are attributed to heat-induced denaturation and shrinkage of myofibrillar proteins and collagen.[Citation41] It was reported that water loss mostly comes from water being expelled by protein denaturation and contraction.[Citation42] Zhu et al.[Citation21] has also reported shear force of the edible abalone meat increased rapidly from 50 to 70°C, but dropped gradually with further increase of temperature, which correlated to the degradation of MHC and paramyosin. Our study confirmed that MHC and paramyosin in PYAM were almost completely degraded after 18 h LTLT processing (). It is believed that both textural changes and color changes in LTLT-processed PYAM are related to the denaturation of myoglobin,[Citation27] which is confirmed by our current study.
Endogenous proteinase systems such as cathepsin, caspase, and calpain play significant roles in determining meat quality,[Citation43,Citation44] through their regulation of proteolysis of myofibrillar and cytoskeletal proteins. Cathepsin L is an endopeptidase located inside the lysosomes of the living muscle cell, and when active they catalyze hydrolysis of internal peptide bonds in meatproteins.[Citation45] It could be released into cytosol and has been detected in the cooking liquor.[Citation46] Caspase-3 plays a central role in signal transduction and final execution in the apoptotic process.[Citation47] Previous researches have revealed that caspase-3 is capable of degrading a number of proteins in muscle.[Citation9,Citation48] In the current study, it is shown that the activities of cathepsin L and caspase-3 were increased by increasing heating time, and the higher level of activity could be sustained after 24 h (,C), which may lead to the complete degradation of MHC and paramyosins, as shown in . In regard to the relationship of cathepsin L and caspase-3, several reports have shown that cathepsins might be downstream or upstream of the activation of caspases.[Citation49,Citation50] It was confirmed in this study that cathepsin L and caspase-3 were all activated together in PYAM during LTLT processing; however, further study is stilled needed to reveal the exact sequence of events that lead to their functionalities.
Another change that occurs during heating process is the oxidation of proteins and lipids. Protein oxidation level could be determined by formation of carbonyl and loss of sulfhydryl. Traore et al.[Citation12]observed a significant increasing in carbonyl content from pork longissimus thoracis muscle after heating. Santélhoutellier et al.[Citation51] also found a threefold increase in carbonyl content from bovine myofibrillar proteins after cooking (100°C, 45 min). Consistent with these early reports, an increase of nearly sevenfold in carbonyl level was detected in PYAM after 27 h heating (). It indicated that massive amount of amino acid residues like arginine, proline, and lysine in PYAM are converted to carbonyl derivatives like α-aminoadipic acids (AAS) and γ-glutamic senialdehydes (GGS).[Citation52] In addition, almost 30% sulfhydryl loss was detected in PYAM after 27 h (). Similar phenomena were observed in threadfin bream (Nemipterus bleekeri) actomyosin.[Citation53] Furthermore, it was also found that the reactive sulfhydryl of tilapia actomyosin would decline, by being oxidized to disulfides in inter- and intramolecules.[Citation54]
Both protein oxidation and lipid peroxidation are believed to be via a free radical chain reaction.[Citation55,Citation56] The abstraction of a hydrogen atom by an ROS leads to the generation of a carbon-centered radical. As shown in , the typical ESR spectra of carbon-centered radical strapping by POBN were detected, which indicated the formation of ROS. The ROS signal was measured after 1 h and rapidly enhanced from 6 to 32 h. Similar results were reported on processed pork,[Citation57] thermal treated beef, and chicken.[Citation58] Moreover, free radicals formation and carybonyl groups increases were detected on salted herrings during ripening,[Citation27] which was consistent with our results. It was also reported that the generation mechanism of specific carbonyl derivatives like AAS and GSS was initiated by ROS and transition metal ions.[Citation6] These results confirmed that ROS-initiated oxidation in PYAM was induced by LTLT treatment. In addition, there are numerous evidences suggesting that oxidation leads to the activation of endogenous proteinase such as cathepsin L or caspaese-3 by different signaling pathways, like mitogen-activated protein kinases pathway[Citation24] or the tumor necrosis factor receptor pathway.[Citation49] Presumably, ROS-initiated oxidation as the upstream signal might induce endogenous proteinase activation and protein degradation and ultimately might lead to quality changes of PYAM during LTLT processing at 55°C ().
Conclusion
In conclusion, we conclude that LTLT processing induced ROS production, protein and lipid oxidation as well as cathepsin L and caspase-3 activation, and the degradation of structural proteins, which eventually leads to changes in PYAM quality. However it needs to be further investigated how ROS and the downstream signals are involved in determining the texture of PYAM during LTLT treatment, by applying special antioxidants to block certain pathways to identify the roles of ROS components.
Additional information
Funding
References
- Bureau of Fisheries in Ministry of Agriculture. China Fishery Statistical Yearbook; China Agriculture press: Beijing, 2016; 29.
- Oyamada, C.; Kaneniwa, M.; Ebitani, K.; Murata, M.; Ishihara, K. Mycosporine-Like Amino Acids Extracted from Scallop (Patinopecten yessoensis) Ovaries: UV Protection and Growth Stimulation Activities on Human Cells. Marine Biotechnology 2008, 10 (2), 141–150.
- Wu, H. T.; Jin, W. G.; Sun, S. G.; Li, X. S.; Duan, X. H.; Li, Y.; Zhu, B. W. Identification of Antioxidant Peptides from Protein Hydrolysates of Scallop (Patinopecten yessoensis) Female Gonads. European Food Research and Technology 2015, 242 (5), 713–722.
- Christensen, M.; Andersen, E.; Christensen, L.; Andersen, M. L.; Baron, C. P. Textural and Biochemical Changes during Ripening of Old-Fashioned Salted Herrings. Journal of the Science of Food and Agriculture 2011, 91 (2), 330.
- Xiong, Y. L.;. Protein Oxidation and Implications for Muscle Food Quality. In Eric A. Decker, Cameron Faustman, Clemente J. Lopez-Bote Antioxidants in Muscle Foods Nutritional Strategies to Improve Quality; Wiley and Sons: New York, 2000; 85–90.
- Lund, M. N.; Heinonen, M.; Baron, C. P.; Estévez, M. Protein Oxidation in Muscle Foods: A Review. Molecular Nutrition and Food Research 2011, 55 (1), 83–95.
- Ouali, A.; Gagaoua, M.; Boudida, Y.; Becila, S.; Boudjellal, A.; Herreramendez, C. H.; Sentandreu, M. A. Biomarkers of Meat Tenderness: Present Knowledge and Perspectives in Regards to Our Current Understanding of the Mechanisms Involved. Meat Science 2013, 95 (4), 854–870.
- Fontanesi, L.; Speroni, C.; Buttazzoni, L.; Scotti, E.; Costa, L. N.; Davoli, R.; Russo, V. Association between Cathepsin L (CTSL) and Cathepsin S (CTSS) Polymorphisms and Meat Production and Carcass Traits in Italian Large White Pigs. Meat Science 2010, 85 (2), 331–338.
- Huang, M.; Huang, F.; Xue, M.; Xu, X.; Zhou, G. The Effect of Active Caspase-3 on Degradation of Chicken Myofibrillar Proteins and Structure of Myofibrils. Food Chemistry 2011, 128 (1), 22–27.
- Chen, L.; Feng, X. C.; Zhang, Y. Y.; Liu, X. B.; Zhang, W. G.; Li, C. B.; Zhou, G. H. Effects of Ultrasonic Processing on Caspase-3, Calpain Expression and Myofibrillar Structure of Chicken during Post-Mortem Ageing. Food Chemistry 2015, 177 (12), 280–287.
- Zakrys-Waliwander, P. I.; O’Sullivan, M. G.; O’Neill, E. E.; Kerry, J. P. The Effects of High Oxygen Modified Atmosphere Packaging on Protein Oxidation of Bovine M. Longissimus Dorsi Muscle during Chilled Storage. Food Chemistry 2012, 131 (2), 527–532.
- Traore, S.; Aubry, L.; Gatellier, P.; Przybylski, W.; Jaworska, D.; Kajak-Siemaszko, K.; Santé-Lhoutellier, V. Effect of Heat Treatment on Protein Oxidation in Pig Meat. Meat Science 2012, 91 (1), 14–21.
- Utrera, M.; Rodríguezcarpena, J. G.; Morcuende, D.; Estévez, M. Formation of Lysine-Derived Oxidation Products and Loss of Tryptophan during Processing of Porcine Patties with Added Avocado Byproducts. Journal of Agricultural and Food Chemistry 2012, 60 (15), 3917–3926.
- Nieto, G.; Jongberg, S.; Andersen, M. L.; Skibsted, L. H. Thiol Oxidation and Protein Cross-Link Formation during Chill Storage of Pork Patties Added Essential Oil of Oregano, Rosemary, or Garlic. Meat Science 2013, 95 (95), 177–184.
- Iglesias, J.; Pazos, M.; Andersen, M. L.; Skibsted, L. H.; Medina, I. Caffeic Acid as Antioxidant in Fish Muscle: Mechanism of Synergism with Endogenous Ascorbic Acid and α-tocopherol. Journal of Agricultural and Food Chemistry 2009, 57 (2), 675–681.
- Lesser, M. P.;. Oxidative Stress in Marine Environments: Biochemistry and Physiological Ecology. Annual Review of Physiology 2006, 68 (1), 253–278.
- Morzel, M.; Gatellier, P.; Sayd, T.; Renerre, M.; Laville, E. Chemical Oxidation Decreases Proteolytic Susceptibility of Skeletal Muscle Myofibrillar Proteins. Meat Science 2006, 73 (3), 536–543.
- Lund, M. N.; Lametsch, R.; Hviid, M. S.; Jensen, O. N.; Skibsted, L. H. High-Oxygen Packaging Atmosphere Influences Protein Oxidation and Tenderness of Porcine Longissimus Dorsi during Chill Storage. Meat Science 2007, 77 (3), 295–303.
- Davies, M. J.;. The Oxidative Environment and Protein Damage. Biochimica Et Biophysica Acta 2005, 1703 (2), 93–109.
- Li, W.; Wang, J.; Sun, J.; Li, W.; Wang, Y.; Zhang, G. Shelf Life Prediction Modeling of Vacuum-Packaged Scallops on the Kinetics of Total Volatile Base Nitrogen. International Journal of Food Engineering 2011, 7 (4), 457–461.
- Zhu, B.; Dong, X.; Sun, L.; Xiao, G.; Chen, X.; Murata, Y.; Yu, C. Effect of Thermal Treatment on the Texture and Microstructure of Abalone Muscle (Haliotis discus). Food Science and Biotechnology 2011, 20 (6), 394–412.
- Dong, X.; Zhang, J.; Xi, Q.; Liu, L.; Li, N.; Fu, H.; Qi, H. Photooxidation and Antioxidant Responses in the Gut of Sea Cucumber Stichopus japonicus Autolysis Exposed to UVC Radiation. Journal of Food Agriculture and Environment 2014, 12 (2), 207–211.
- Bradford, M. M.;. A Rapid and Sensitive Method for the Quantitation of Microgram Quantities of Protein Utilizing the Principle of Protein-Dye Binding. Analytical Biochemistry 1976, 72 (s 1–2), 248–254.
- Qi, H.; Fu, H.; Dong, X.; Feng, D.; Li, N.; Wen, C.; Zhu, B. Apoptosis Induction Is Involved in UVA-induced Autolysis in Sea Cucumber Stichopus japonicus. Journal of Photochemistry and Photobiology B Biology 2016, 158, 130–135.
- Oliver, C. N.; Ahn, B. W.; Moerman, E. J.; Goldstein, S.; Stadtman, E. R. Age-Related Changes in Oxidized Proteins. Journal of Biological Chemistry 1987, 262 (12), 5488–5491.
- Lepperblilie, A. N.; Berg, E. P.; Buchanan, D. S.; Keller, W. L.; Maddockcarlin, K. R.; Berg, P. T. Effectiveness of Oxygen Barrier Oven Bags in Low Temperature Cooking on Reduction of Warmed-Over Flavor in Beef Roasts. Meat Science 2014, 96 (96), 1361–1364.
- Christensen, L.; Ertbjerg, P.; Aaslyng, M. D.; Christensen, M. Effect of Prolonged Heat Treatment from 48°C to 63°C on Toughness, Cooking Loss and Color of Pork. Meat Science 2011, 88 (2), 280–285.
- Abe, T.; Miyashita, K. Heat Treatment of Scallop Adductor Muscle Using Super Heated Steam. Journal of Food Science 2007, 72 (6), E345.
- Goetz, D. W.; Whisman, B. A. Occupational Asthma in a Seafood Restaurant Worker: Cross-Reactivity of Shrimp and Scallops. Annals of Allergy Asthma and Immunology 2001, 85 (6 Pt 1), 461–466.
- Bahuaud, D.; Mørkøre, T.; Østbye, T. K.; Veiseth-Kent, E.; Thomassen, M. S.; Ofstad, R. Muscle Structure Responses and Lysosomal Cathepsins B and L in Farmed Atlantic Salmon (Salmo salar L.) Pre- and Post-Rigor Fillets Exposed to Short and Long-Term Crowding Stress. Food Chemistry 2010, 118 (3), 602–615.
- Zhou, D. Y.; Chang, X. N.; Bao, S. S.; Song, L.; Zhu, B. W.; Dong, X. P.; Liu, Y. X. Purification and Partial Characterisation of a Cathepsin L-Like Proteinase from Sea Cucumber (Stichopus japonicus) and Its Tissue Distribution in Body Wall. Food Chemistry 2014, 158 (8), 192–199.
- Buettner, G. R.;. Spin Trapping: ESR Parameters of Spin Adducts. Free Radical Biology and Medicine 1987, 3 (3), 259–303.
- Vaudagna, S. R.; Sánchez, G.; Neira, M. S.; Insani, E. M.; Picallo, A. B.; Gallinger, M. M.; Lasta, J. A. Sous Vide Cooked Beef Muscles: Effects of Low Temperature–Long Time (LT–LT) Treatments on Their Quality Characteristics and Storage Stability. International Journal of Food Science and Technology 2002, 37 (4), 425–441.
- Rinaldi, M.; Dall’Asta, C.; Paciulli, M.; Cirlini, M.; Manzi, C.; Chiavaro, E. A Novel Time/Temperature Approach to Sous Vide Cooking of Beef Muscle. Food and Bioprocess Technology 2014, 7 (10), 2969–2977.
- Christensen, M.; Purslow, P. P.; Larsen, L. M. The Effect of Cooking Temperature on Mechanical Properties of Whole Meat, Single Muscle Fibres and Perimysial Connective Tissue. Meat Science 2000, 55 (3), 301–307.
- Roldán, M.; Antequera, T.; Hernández, A.; Ruiz, J. Physicochemical and Microbiological Changes during the Refrigerated Storage of Lamb Loins Sous-Vide Cooked at Different Combinations of Time and temperature.[J]. Food Science and Technology International 2015, 21 (7), 512–522.
- Naveena, B. M.; Khansole, P. S.; Shashi, K. M.; Krishnaiah, N.; Kulkarni, V. V.; Deepak, S. J. Effect of Sous Vide Processing on Physicochemical, Ultrastructural, Microbial and Sensory Changes in Vacuum Packaged Chicken Sausages. Food Science and Technology International 2016, 23 (1), 75–85.
- Baldwin, D. E.;. Sous Vide Cooking: A Review. International Journal of Gastronomy and Food Science 2012, 1 (1), 15–30.
- Becker, A.; Boulaaba, A.; Pingen, S.; Röhner, A.; Klein, G. Low Temperature, Long Time Treatment of Porcine M. Longissimus Thoracis Et Lumborum in a Combi Steamer under Commercial Conditions. Meat Science 2015, 110, 230–235.
- Mortensen, L. M.; Frøst, M. B.; Skibsted, L. H.; Risbo, J. Long-Time Low-Temperature Cooking of Beef: Three Dominant Time-Temperature Behaviours of Sensory Properties. Flavour 2015, 4 (1), 1–10.
- Sánchez, D. P. J.; Gázquez, A.; Ruiz-Carrascal, J. Physico-Chemical, Textural and Structural Characteristics of Sous-Vide Cooked Pork Cheeks as Affected by Vacuum, Cooking Temperature, and Cooking Time. Meat Science 2012, 90 (3), 828–835.
- Kondjoyan, A.; Oillic, S.; Portanguen, S.; Gros, J. B. Combined Heat Transfer and Kinetic Models to Predict Cooking Loss during Heat Treatment of Beef Meat. Meat Science 2013, 95 (2), 336–344.
- Etherington, D. J.;. The Contribution of Proteolytic Enzymes to Postmortem Changes in Muscle. Journal of Animalence 1984, 59 (6), 1644–1650.
- Lonergan, E. H.;. Biochemistry of Postmortem Muscle - Lessons on Mechanisms of Meat Tenderization. Meat Science 2010, 86 (1), 184–195.
- Agarwal, S. K.;. Proteases Cathepsins — A View. Biochemical Education 2010, 18 (2), 67–72.
- Christensen, L.; Ertbjerg, P.; Løje, H.; Risbo, J.; Fw, V. D. B.; Christensen, M. Relationship between Meat Toughness and Properties of Connective Tissue from Cows and Young Bulls Heat Treated at Low Temperatures for Prolonged Times. Meat Science 2012, 93 (4), 787–795.
- Adams, J. M.;. Ways of Dying: Multiple Pathways to Apoptosis. Genes and Development 2003, 17 (20), 2481–2495.
- Kemp, C. M.; Wheeler, T. L. Effects of Manipulation of the Caspase System on Myofibrillar Protein Degradation in Vitro. Journal of Animal Science 2011, 89 (10), 3262–3271.
- Jäättelä, M.; Tschopp, J. Caspase-Independent Cell Death in T Lymphocytes. Nature Immunology 2003, 4 (5), 416–423.
- Cailhier, J. F.; Sirois, I.; Laplante, P.; Lepage, S.; Raymond, M. A.; Brassard, N.; Hébert, M. J. Caspase-3 Activation Triggers Extracellular Cathepsin L Release and Endorepellin Proteolysis. Journal of Biological Chemistry 2008, 283 (40), 27220–27229.
- Santélhoutellier, V.; Astruc, T.; Marinova, P.; Greve, E.; Gatellier, P. Effect of Meat Cooking on Physicochemical State and in Vitro Digestibility of Myofibrillar Proteins. Journal of Agricultural and Food Chemistry 2008, 56 (4), 1488–1494.
- Roldan, M.; Antequera, T.; Armenteros, M.; Ruiz, J. Effect of Different Temperature-Time Combinations on Lipid and Protein Oxidation of Sous-Vide Cooked Lamb Loins. Food Chemistry 2014, 149 (149), 129–136.
- Yongsawatdigul, J.; Park, J. W. Thermal Denaturation and Aggregation of Threadfin Bream Actomyosin. Food Chemistry 2003, 83 (3), 409–416.
- Ko, W. C.; Yu, C. C.; Hsu, K. C. Changes in Conformation and Sulfhydryl Groups of Tilapia Actomyosin by Thermal Treatment. LWT - Food Science and Technology 2007, 40 (8), 1316–1320.
- Caroline, P. B.; Henrik, J. A. Myoglobin-Induced Lipid Oxidation. A Review. Journal of Agricultural and Food Chemistry 2002, 50 (14), 3887–3897.
- Michael, J. D.;. Protein Oxidation and Peroxidation. Biochemical Journal 2016, 473 (7), 805–825.
- Carlsen, C. U.; Andersen, M. L.; Skibsted, L. H. Oxidative Stability of Processed Pork. Assay Based on ESR-detection of Radicals. European Food Research and Technology 2001, 213 (3), 170–173.
- Ma, H. J.; Ledward, D. A.; Zamri, A. I.; Frazier, R. A.; Zhou, G. H. Effects of High Pressure/Thermal Treatment on Lipid Oxidation in Beef and Chicken Muscle. Food Chemistry 2007, 104 (4), 1575–1579.