ABSTRACT
Traditionally fermented Pixian broad bean paste (PBP) is a traditional condiment in China and is recognized to have superior quality than the industrially fermented. This work aimed to identify the dominant microbes and unique aroma compounds in traditionally fermented PBP with respect to those in the industrially fermented one. Results showed that several species of lactic acid bacteria, yeasts, Aspergillus, molds, and Staphylococcus were only detected in traditionally fermented PBP, namely, Leuconostoc lactis, Staphylococcus xylosus, S. succinus, Amylomyces rouxii, Mucor genevensis, Absidia corymbifera, Issatchenkia orientalis, basidiomycete yeast sp., and Metschnikowia pulcherrima. Furthermore, the traditionally fermented PBP contained more compounds that contribute to its aroma than those in industrially fermented PBP. A total of 28 aroma substances only were detected in the traditionally fermented PBP. Among these substances, seven were considered aroma-active compounds, namely, ethyl caprylate, hexadecanoic acid methyl ester, 2-methyl-pyrazine, 2,6-dimethyl- pyrazine, 2-pentyl furan, 2-acetyl furan, and hexadecanoic acid. This work provides useful information on the microbial composition and aroma compounds of PBP that can be used to upgrade the quality of industrially fermented PBP.
Introduction
Pixian broad bean paste (PBP) is a famous Chinese condiment regarded as “the soul of Sichuan cuisine” due to its palatable taste, reddish-brown luster, and strong savory aroma.[Citation1] Given its origin in Pixian county, the PBP harbors a unique microbiological composition, which in turn affords the favorable taste and flavor. The microbial and aroma compounds present in the different types of fermented broad bean pastes exhibit similarities and differences to a certain extent. For example, lactic acid bacteria (LAB) and yeasts are the dominant microbes in traditional Korean fermented doenjang and Japanese miso, which are typical fermented soybean foods. However, the microbial and aroma compositions of fermented soybean foods vary because of regional and technical inheritance. In doenjiang, the LAB Leuconostoc mesenteroide, Tetragenococcus halophilus, and Enterococcus faecium, as well the fungi Mucor plumbeus, Aspergillus oryzae, and Debaryomyces hansenii, are the most common species.[Citation2] In Japanese miso, Weissella confusa, Lactobacillus fermentum, L. plantarum, Staphylococcus xylosus, T. halophilus, Zygosaccharomyces rouxii, and A. oryzae are the dominant microbes.[Citation3] In addition, the aroma compound profiles of the products vary with the microflora involved.[Citation4] The most common aroma compounds in Japanene miso and natto, Chinese sufu, and Thai thus nao include esters (ethyl 2-methyl butyrate and ethyl hexanoate), acids (acetic acid and 2/3-methyl butanoic acid), pyrazines, and phenolic compounds.[Citation5] Nearly 100 aroma compounds have been identified in these three fermented soybean products. Therefore, the fermented products from different countries possess unique sensory and textural properties due to the different microbes and aroma compounds enriched and produced in the production process. However, information on the diverse microbial community and the proportions of sauce-enhancing flavor compounds is limited for the traditionally fermented and industrially fermented PBP.
The traditional fermentation of PBP requires at least 2 years to achieve a desirable flavor and involves a high contamination risk. Inoculated industrial fermentation has been the most important strategy for solving this problem. The market share of traditionally fermented PBP has been continuously decreasing and gradually taken over by the industrially fermented PBP because of the long production period and low production efficiency of the former.[Citation6] Ancestral culture and intelligence should be effectively protected for their sustainable development. Industrial fermentation only needs 6 months for sale. However, the taste and quality of industrially fermented PBP cannot compare to its traditionally fermented counterpart.[Citation6] This difference is mainly due to the microbial assemblage and aroma compounds in the traditional fermentation process, which is more complex than the industrial process.[Citation5,Citation7] Although many researchers have focused on optimizing koji making, only few researchers have focused on identifying the microbial profile and flavor components in the traditionally fermented PBP.[Citation8,Citation9] In a previous study,[Citation8] adding A. oryzae while making koji can improve the amino-acid composition and flavor of PBP. Adding A. oryzae, Saccharomyces cerevisiae, Candida utilis, T. halophilus, and L. plantarum in the industrial fermentation of PBP can reduce the production time by half and increase the aroma fragrant components by 2–4 times.[Citation10]
To the best of our knowledge, this study could be the first to identify the microbial community and aroma compounds in traditionally fermented PBP and compare with those in the industrially fermented PBP. The results of this foundational work could help improve the quality and understanding of the characteristics of large-scale industrial processing.
Materials and methods
Fermentation and sampling
Industrially fermented PBP (sample A) was purchased from a company in Sichuan Province. Traditionally fermented PBP (sample B) was obtained from Chengdu Sihowow Food Stuff Co., Ltd., which is the oldest company brewing PBP in Sichuan, China. The compared fermentation processes share similar principles and include three key stages. The first stage is starter making. In industrial fermentation, the beans are inoculated with quality inocula (L. plantarum, W. confusa, A. oryzae, A. sojae, and Z. rouxii) for 4 days. This production method presents advantages, such as production time reduction, mass production, and strong controllability. However, the drawback is the inferior taste of the PBP. In traditional fermentation, the starters are mixed with flour for 7 days in open air. This production method allows the production of more microbes and better-tasting PBP. However, this method requires considerable time to achieve the desired effect. The second stage is the incipient fermentation, which lasts around 4/8–180 days. In this stage, two kinds of koji are mixed with 30%–40% (v/w) brine (saltiness: approximately 20%) before being converted into mature beans. The final stage is the late fermentation, which requires around 180–720 days. At this stage, chili paste is added during the post-ripening period. The entire procedure of industrial fermentation is carried out in a modern, controlled environment under strict sanitary regulations. By contrast, traditional fermentation is exposed to air.
DNA extraction and PCR–denaturing gradient gel electrophoresis (DGGE) analysis
The microbial DNA of the fermented sample was extracted from koji at the end of fermentation by the freeze–thaw method combined with the CTAB method with some modifications.[Citation11] The fungal total DNA extraction required bead-beating to disrupt the cell walls. The process of PCR–DGGE and analysis of the DGGE profile are the same as those reported by Yang et al.[Citation12] A cluster analysis of the band patterns was performed with the unweighted-pair-group method using arithmetic averages (UPGMA). The relative abundance of each band was calculated as the ratio of individual band intensity to the total band intensity in the same lane. Furthermore, the relative abundances of the main genera were obtained by band sequencing and congeneric summing.
Detection and identification of aroma compounds
The samples (5 g) were each placed in a 20-mL vial and equilibrated at 60°C for 15 min. The SPME tip (MPS 2; Gerstel, Mülheim, Germany) was inserted in the vessel and incubated at 60°C for 45 min. Then, the samples were withdrawn and injected into a GC chromatograph. The aromas were separated by a PEG 20M silica capillary column (30 m in length, 25 mm in diameter, 0.25-mm film in thickness, and TR-5MS), with helium as the carrier gas (flow rate of 1 mL/min). The injector and detector temperatures were set at 250°C. The oven temperature was set at 40°C and held for 5 min and then raised to 220°C at a rate of 5°C/min. The ionization energy was 70eV, and the range of molecular weight scanned was 33–450 amu. The column flow was split between an MS detector and a sniffing port at a ratio of 1:1. The constituents were identified by comparing their retention times with those of authentic samples. The constituents were also recognized by computer matching against commercial (NIST 98 and ADAMS) mass spectral libraries and a home-made mass spectral library constructed from pure substances, as well as MS literature data. The GC–MS data and a study of comparison of means were analyzed by one-way ANOVA (Tukey’s test) using SPSS 19.0.
Results and discussion
Dynamic succession of bacterial communities in two fermentation processes
The amount, nature, and distribution of the microorganisms determine the speed of fermentation, as well as the quality of fermented beans.[Citation6] Thus, a thorough understanding of the microbial communities and the aroma compounds is a prerequisite to producing high-quality PBP by industrial fermentation. For this purpose, the dynamic succession of microbes and aroma compounds in the 0-day to 360-day samples prepared through the two types of PBP fermentation, i.e., traditional and industrial, were analyzed.
The representative DGGE fingerprints are shown in , and the results of the sequencing of the highlighted bands are reported in . Ten and seventeen bacterial species were found in the traditionally and industrially fermented products, respectively ( and ). Among these species, nearly 50% of the bacteria were identified as LAB, which were widely distributed throughout the entire processes of the two fermentation types. Specifically, W. confusa and L. plantarum were found in the three fermentation stages of traditional and industrial fermentation. Thus, these species were the dominant bacteria in the PBP. Weissella confusa and L. plantarum were reported as the dominant bacteria in Chinese soy sauce, Korean dojiang, and other fermented foods. These bacteria are responsible for generating lactic acid, organic acid, and aroma compounds, as well as inhibiting the growth of pathogenic bacteria.[Citation3] Lactobacillus plantarum is the dominant bacterium in plant food fermentation during koji making and the initial stage for breakdown of sugar and synthesized amino acids, nucleotides, fatty acids.[Citation13] It laid a foundation for next two stages.
Table 1. Identification of DGGE bands of bacterial community from industrial and traditional fermentation.
Figure 1. DGGE profiles of bacteria community in processes of industrial fermentation (a) and traditional fermentation (b). Sample A was collected on day 0, 1, 3, 4, 11, 25, 40, 60, 110, 180, 360, and 720 while B was on day 1, 4, 7, 14, 30, and 240.
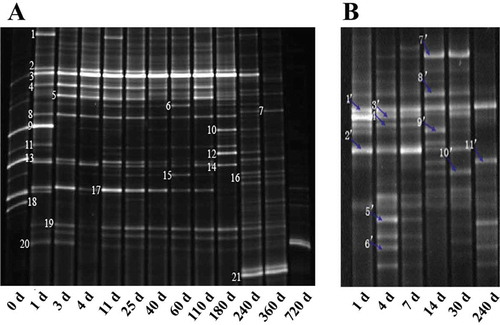
Besides, W. confusa and L. plantarum, other LAB, namely, L. lactis and L. namurensis, were found in the traditionally fermented PBP. These four bacterial species have been reported as the most dominant bacteria in traditional food fermentation.[Citation2,Citation14] In particular, L. lactis and L. namurensis are commonly reported in the fermentation of other traditional fermented foods. Lactobacillus lactis is the most important bacterial species in dairy fermentation because it promotes cheese maturation and generates aroma compounds from citric acid fermentation.[Citation15] In the current study, W. confusa and L. lactis were both detected in the 14-day to 30-day samples probably because both species belong to the family Leuconostocaceae[Citation16] and may thus act synergistically.
Furthermore, LAB S. xylosus and S. succinus were only found in traditional fermentation. These species were the dominant bacteria in traditional PBP fermentation because they occurred throughout the entire process of fermentation and were in greater abundance than the other species. Previous studies demonstrated that Staphylococcus is the dominant genus in the fermentation of other foods[Citation17] because the bacteria break down proteins into small polypeptides and amino acids and endow the product with unique flavor.Citation[18] Previous authors reported that staphylococci were used as starter culture in the Italian traditional sausage Felino salami without LAB addition. The staphylococci were adopted because their activity imparts a sweet rather than a sour taste to the sausage.[Citation19] Staphylococcus xylosus can produce 3-methyl butyl alcohol and endows fermented meat with special aroma. The amount of 3-methyl butyl alcohol in the traditionally fermented PBP was twice that in the industrially fermented PBP (). Staphylococcus xylosus has also been reported as an important contributor to the flavor in soy sauce,[Citation20] miso,[Citation21] and doenjiang.[Citation2] Therefore, staphylococci may not only improve the meat color, making it bright red, but also impart a sweet taste to PBP. Above all, S. xylosus and S. succinus may be direct or indirect causes of the improved quality of traditionally fermented PBP over those of industrially fermented PBP.
Table 2. Identification of DGGE bands of fungi community from industrial and traditional fermentation.
Table 3. Aroma compounds from industrial and traditional fermentation for 720 days.
Dynamic succession of fungi in two fermentation processes
Sixteen and nineteen fungal species were identified in traditional and industrial fermentation, respectively ( and ). The dominant fungi in the two fermentation processes were yeasts and Aspergillus, namely, A.oryzae, Rhiaopus oryzae, A. sojae, Pichia anomala, Debarryomyces hansenii, Z. rouxii, and Candida sp. These fungi have also been found in other fermented foods.[Citation2,Citation3,Citation6]
Figure 2. DGGE profiles of fungi community in processes of industrial fermentation processes (a) and traditional fermentation (b). Sample A was collected on day 0, 1, 3, 4, 11, 25, 40, 60, 110, 180, 360, and 720 while B was on day 1, 4, 7, 14, 30, and 240.
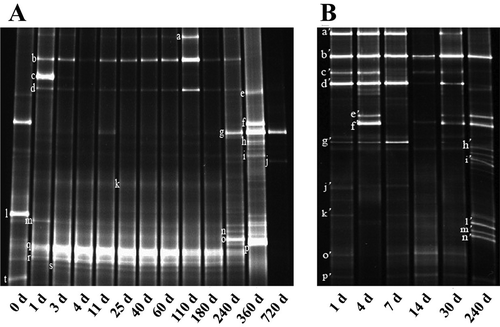
Three yeast species and three genera of molds were found only in traditional fermentation, namely, Issatchenkia orientalis, basidiomycete yeast species, Metschnikowia pulcherrima, Amylomyces rouxii, M. genevensis, and Absidia corymbifera. Among these species, I. orientalis and basidiomycete yeast sp. were isolated only in the later fermentation stage (day 240). Issatchenkia orientalis can produce organic acids and aroma compounds (butyric acid, isovaleric acid, hexanoic acid, and 2, 3-butanediol) and tolerate the associated toxicity.[Citation22,Citation23] Metschnikowia pulcherrima was found throughout the entire fermentation process. It is a common yeast in wine fermentation and other fermented foods.[Citation24] Such application is due to the yeast’s proteolytic activity for protein, which is broken down into many enzymes and amino acids,[Citation24] as well as its high antagonistic effect on A. flavus, Proteus vulgaris, Escherichia coli, and A. fumigates.[Citation25] Consistent with this finding, M. pulcherrima is an indispensable fungus that plays an important role in the fermentation process of PBP. Thus, this fungus merits considerable attention. Various fungi reached the maximum in days 240 and 360 under both traditional and industrial fermentation.
As unique fungi in traditional fermentation, molds are generally considered among the dominant microorganisms in koji,[Citation26] soybean paste,[Citation27] and other Asian fermented foods. Such dominance may be attributed to their ability to decompose broad bean into different glucose compounds. This decomposition is favorable to the subsequent organic reaction and starch saccharification.[Citation6,Citation28] Consistent with this finding, A. rouxii, M. genevensis, and A. corymbifera were found only in the koji-making stage of traditional fermentation in the current study. These species help facilitate the proteolysis of proteinsinto peptides and amino acids.[Citation28,Citation29] The growth of Mucoraceae can be inhibited by brine addition.[Citation30] Thus, in the current study, molds were all identified on days 1, 4, and 7 under the traditional fermentation but disappeared after day 7. Despite the short time, these molds still exert irreplaceable effects in establishing a good foundation for the succeeding stages.
Enterococcus faecium, Escherichia sp., Pantoea agglomerans, and Micrococcus sp., which are harmful microbes, covered almost the entire process of the industrial fermentation of PBP. By contrast, the uncultured Enterobacteriales bacterium and Enterobacter cloacae was only detected on day 4 and subsequently disappeared in traditional fermentation. Previous studies demonstrated that L. plantarum exerts antifungal effects on Penicillium sp.[Citation31] Saprophytic yeasts, such as Candida sp. and Pichia sp., can inhibit aflatoxin B1 production.[Citation32] Lactobacillus plantarum, Candida sp., and Pichia sp. existed in the two fermentation processes under study, but harmful bacteria were rarely identified in the traditional fermentation. This aspect requires further research.
The compositions of bacteria and fungi in the current study were generally similar to those of Chinese soy sauce.[Citation2] However, Staphylococcus sp. and molds, the dominant microbes in sausage and soybean fermentation, were identified as unique microorganisms in traditional fermentation. They likely contribute directly or indirectly to the final quality and flavor of traditionally fermented PBP. Thus, the traditional fermentation of PBP involves a highly complex biological community. The compositions of LAB, yeasts, and Aspergillus also showed significant variation. This variation may lead to differences in textures and flavors between industrial and traditional fermentation.
Cluster analysis of DGGE fingerprints
The other objective of this study was to compare between the microbial diversities in industrial and traditional fermentation of different durations. Two hierarchical clustering dendrograms based on the UPGMA clustering of the similarity coefficients of the normalized PCR–DGGE patterns are shown in and . The bacterial DGGE profiles of industrial fermentation were clustered into five sets on the basis of pairwise similarity coefficients over 0.50 (). Meanwhile, the bacteria in the late stage of industrial fermentation were divided into two groups that showed a low similarity coefficient. On day 720, all the bacteria were grouped into one cluster. Thus, the structure of the bacterial community under industrial fermentation was stable over the same period and underwent a remarkable succession in the later stage. By contrast, the bacterial community under traditional fermentation formed one cluster and showed a low similarity coefficient with the other two stages on day 240 (). This result indicates that the structure of the bacterial community varied across the three stages in traditional fermentation, but a homogeneous distribution appeared over the same period. Similar phenomena were observed in the fungal profile analyses of industrial fermentation () and traditional fermentation (). This phenomenon might be induced by the addition of brine and chili, which helped substantially alter microbial structures.
Figure 3. Cluster analysis of bacterial DGGE community in processes of industrial fermentation processes (a) and traditional fermentation (b). The bacterial DGGE profiles of sample A clustered into 5 sets (0 d; 1 d, 3 d; 4 d, 11 d, 25 d, 40 d, 60 d, 110 d, 180 d; 240 d, 360 d; 720 d), while sample B clustered into three sets (1 d, 4 d, 7 d; 14 d, 30 d; 240 d).
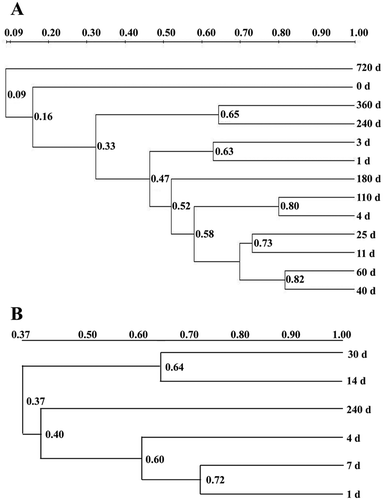
Figure 4. Cluster analysis of fungi DGGE community in processes of industrial fermentation (a) and traditional fermentation (b). The fungi DGGE profiles of sample A clustered into 4 sets (0 d; 1 d, 3 d; 4 d, 11 d, 25 d, 40 d, 60 d, 110 d, 180 d; 240 d, 360 d, 720 d), while sample B clustered into three sets (1 d, 4 d, 7 d; 14 d, 30 d; 240 d).
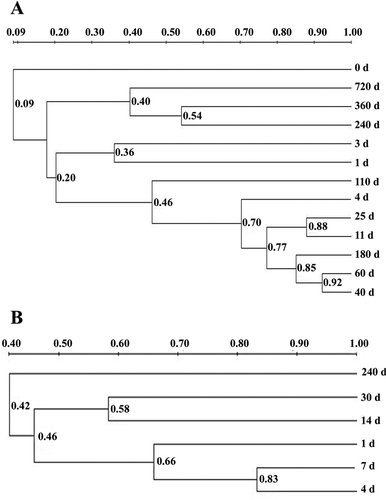
Analyses of aroma compounds in two fermentation processes
The difference in the aroma-active compounds between the two fermentation processes should be identified before the quality of industrially fermented PBP is improved. Most aroma compounds are produced in the late fermentation stage.[Citation33] Therefore, in this study, the aroma compounds were observed on day 720 of the traditional and industrial fermentation process.
Totals of 56 and 37 aroma compounds were identified in traditional and industrial fermentation, respectively (). These compounds were divided into esters, aldehydes, acids, alcohols, phenols, ketones, and pyrazines. Among them, esters, aldehydes, and alcohols were the largest groups in this study. Esters are highly important indicators of the quality grade of sauce fragrance. These compounds impart a unique potpourri and sweet fragrance to PBP, and the fragrance masks the amargoso and sharp smell from amino acids and fatty acids.[Citation5] The alkane and olefin contents were negligible and thus disregarded in this study. All aroma compounds in this study were detected in other fermented foods, such as miso and soy sauce.[Citation2,Citation5] Furthermore, 28 of the aroma compounds were identified in the two types of fermented PBP (). Among these 28 aroma compounds, on the basis of previous studies of other fermented foods, the aroma-active compounds were ethyl ester, ethyl palmitate, ethyl linoleate, ethyl oleate, ethyl laurate, tetradecanoic acid, ethyl ester, ethyl pentadecanoate, 4-vinyl guaiacol, 4-ethyl phenol, 2-methoxy phenol, and 4-hydroxy-2,5-dimethyl-3(2H)-furanone.[Citation9,Citation34–Citation37] The top three components in terms of relative abundance in the two fermentation processes were ethyl palmitate, ethyl linoleate, and ethyl oleate. These three compounds also presented widely in liquor[Citation38] and sufu.[Citation35] Huang found similar results.[Citation9] Ethyl palmitate has a wax-like odor; ethyl oleate has a floral scent; ethyl linoleate has a slight astringency in sausage fermentation.[Citation39] Thus, these compounds substantially contribute to the aroma of PBP.
Remarkably, traditional fermentation presented with more abundant aromas than industrial fermentation. This difference might explain why the former process produces a better scent. Differences in aroma compounds were observed between the two fermentation processes, and roughly 28 unique aromas were identified in traditional fermentation. The major difference between the aromas of the traditionally and industrially fermented PBPs could be attributed to these 28 substances. Among the 28 substances, only 7 were reported as the main aroma substances in previous study, namely, ethyl caprylate, hexadecanoic acid methyl ester, 2-methyl-pyrazine, 2,6-dimethyl-pyrazine, 2-pentyl furan, 2-acetyl furan, and hexadecanoic acid. Ethyl caprylate and hexadecanoic acid methyl ester, were reported as the main aroma substances in wine.[Citation40] Most acids release irritating odors, which partly play in compromising the taste. Hexadecanoic acid, which is a major acid in Chinese soy sauce,[Citation36] has been described as having a “sweat-like,” cheesy, fatty, sour, rancid, and pungent-like odor.[Citation41]
Moreover, 2-methyl-pyrazine, 2,6-dimethyl-pyrazine, 2-pentyl furan, and 2-acetyl furan, which are commonly found in other fermented foods, were only detected in traditional fermentation. The two detected pyrazines affect the taste of Chinese rice wine.[Citation42] The two detected furans exhibited sweet, fruity, nutty, and caramel-like odors produced by the Maillard reaction in other fermented bean products.[Citation43] Despite their low contents, ethyl caprylate, 2-pentyl furan, and 2-acetyl furan significantly contributed to the aroma of the traditionally fermented PBP.
Our results showed that the aroma compounds in the traditionally fermented PBP were similar to those of rice wine and Chinese soy sauce. Variations in the aroma compounds, such as hexadecanoic acid, 2-methyl-pyrazine, and 2,6-dimethyl-pyrazine, were only observed in the traditionally fermented PBP. The application of the industrial fermentation technique could significantly reduce production time and cost, as well as enable mass production. Even so, industrial fermentation cannot duplicate the aroma profiles of the traditionally fermented PBP. The unique aroma compounds could be the key odorants responsible for the superior taste of traditionally fermented PBP to that of industrially fermented PBP. This study could serve as reference for the improvement and enhancement of the quality of industrially fermented PBP.
Conclusions
This study is the first investigation on the unique microbial community and aroma compounds of traditionally fermented PBP relative to those of industrially fermented PBP. The microbial and aroma compounds in PBP are similar to those in other fermented foods, such as Chinese soy sauce and Chinese rice wine. Furthermore, LAB, yeasts, Aspergillus, and 28 aroma substances are dominant in the two fermentation processes during the 2-year-long fermentation. However, several species of LAB, yeasts, Aspergillus, molds, Staphylococcus, and 28 unique aroma substances were only detected in the traditional fermentation of PBP. These microbes and aroma compounds may be the principal contributors to the superior taste of traditionally fermented PBP to that of industrially fermented PBP. This study could serve as reference for the improvement and enhancement of the quality of industrially fermented PBP. Further work should be conducted to investigate the influences of the detected microbes on the aroma substances.
Funding
This work was financially supported by the Ministry of Science and Technology of China [grant number 2015DFR31060] and the Department of Science and Technology of Sichuan [grant number 2016SZ0068].
Additional information
Funding
References
- Youjun, L. Analysis of “Pixian Soybean Paste”. China Brewing 2008, 2008 (1), 19–23.
- TaeWoon, K.; Junhwa, L.; Sunggeon, K.; Minhee, P.; Haechoon, C.; Haeyeong, K. Analysis of Microbial Communities in Doenjang, a Korean Fermented Soybean Paste, Using Nested PCR-denaturing Gradient Gel Electrophoresis. International Journal of Food Microbiology 2009, 131, 265–271.
- Tanaka, Y.; Watanabe, J.; Mogi, Y. Monitoring of the Microbial Communities Involved in the Soy Sauce Manufacturing Process by PCR-denaturing Gradient Gel Electrophoresis. Food Microbiology 2012, 31 (1), 100–106.
- Tian, Y.; Zhao, Y.; Huang, J.; Zeng, H.; Zheng, B. Effects of Different Drying Methods on the Product Quality and Volatile Compounds of Whole Shiitake Mushrooms. Food Chemistry 2016, 197 (Pt A), 714–722.
- Seungjoo, L.; Bomi, A. Comparison of Volatile Components in Fermented Soybean Pastes Using Simultaneous Distillation and Extraction (SDE) with Sensory Characterisation. Food Chemistry 2009, 114, 600–609.
- Xiuzhi, G.; Hui, L.; Xinxin, Y.; Yiqian, L.; Xiaodong, W.; Wensheng, X.; Qigen, T.; Zongjun, C. Microbial Floral Dynamics of Chinese Traditional Soybean Paste (Doujiang) and Commercial Soybean Paste. Journal of Microbiology and Biotechnology 2013, 23 (12), 1717–1725.
- Ercolini, D.;. High-Throughput Sequencing and Metagenomics: Moving Forward in the Culture-Independent Analysis of Food Microbial Ecology. Applied and Environmental Microbiology 2013, 79 (10), 3148–3155.
- Feng, L.; Zhao, P.; Zhou, C.; Xie, B. Pixian Soybean Paste Compound Bacteria Starter-Making Improve Enzyme System Composition Research. China Brewing 2012, 31 (12), 30–32.
- Zhu, H.; Ximin, P.; Chaolan, L.; Ruizhi, H.; Rongqing, Z. The Analysis on Volatile Aroma Compounds and Their Variation Regulation during Ripening Process of Pixian Horsebean Chili Paste. China Condiment 2009, 3 (34), 106–111.
- Dafeng, Z.; Yanling, Z.; Mingyuan, L. The Development of Compound Microorganism Bacterium Agent Using in the Production of Pixian Bean Paste. China Condiment 2012, 37 (7), 62–68.
- Murray, M. G.; Thompson, W. F. Rapid Isolation of High Molecular Weight Plant DNA. 1980, 8 (19), 4321–4326.
- Yang, Y.; Deng, Y.; Jin, Y.; Liu, Y.; Xia, B.; Sun, Q. Dynamics of Microbial Community during the Extremely Long-Term Fermentation Process of a Traditional Soy Sauce. Journal of the Science of Food and Agriculture 2017, 97 (10), 3220–3227.
- Jos, B.; Roland, S.; Marie-Camille, Z.; David, V.; Raymond, D. P.; Annick, M.; Michiel, K.; De Vos, W. M.; Harald, B.; Frank, D. The Complete Genomes of Lactobacillus Plantarum and Lactobacillus Johnsonii Reveal Extensive Differences in Chromosome Organization and Gene Content. Micobiology 2004, 150, 3601–3611.
- Tabanelli, G.; Torriani, S.; Rossi, F.; Rizzotti, L.; Gardini, F. Effect of Chemico-Physical Parameters on the Histidine Decarboxylase (Hdca) Enzymatic Activity in Streptococcus Thermophilus PRI60. Journal of Food Science 2012, 77 (4), M231–M237.
- Hemme, D.; Foucaud-Scheunemann, C. Leuconostoc, Characteristics, Use in Dairy Technology and Prospects in Functional Foods. International Dairy Journal 2004, 14 (6), 467–494.
- Katina, K.; Maina, N. H.; Juvonen, R.; Flander, R.; Johansson, L.; Virkki, L. In Situ Production and Analysis of Weissella Confusa Dextran in Wheat Sourdough. Food Microbiology 2009, 26 (7), 734–743.
- Yinzhuo, Y.; Yulin, Q.; Diji, F.; Jingyu, C.; Beizhong, H. Microbial Composition during Chinese Soy Sauce Koji-Making Based on Culture Dependent and Independent Methods. Food Microbiology 2013, 34 (1), 189–195.
- Dalmis, U.; Soyer, A. Effect of Processing Methods and Starter Culture (Staphylococcus Xylosus and Pediococcus Pentosaceus) on Proteolytic Changes in Turkish Sausages (Sucuk) during Ripening and Storage. Meat Science 2008, 80 (2), 345–354.
- Urso, R.; Rantsiou, K.; Dolci, P.; Rolle, L.; Comi, G.; Cocolin, L. Yeast Biodiversity and Dynamics during Sweet Wine Production as Determined by Molecular Methods. FEMS Yeast Research 2008, 8 (7), 1053–1062.
- Gunilla, J.; Jeanlous, B.; Mats, L.; Natualie, T.; Elisabeth, B. Lipolysis Proteolysis and Formation of Volatile Components during Ripening of a Fermented Sausage with Pediococcus Pentosaceus and Staphylococcu Sxylosus as Starter Culture. Meat Science 1994, 38 (2), 203–218.
- Beck, H. C.; Hansen, A. M.; Lauritsen, F. R. Metabolite Production and Kinetics of Branched-Chain Aldehyde Oxidation in Staphylococcus Xylosus. Enzyme and Microbial Technology 2002, 31, 94–101.
- Han, X.; Zengyi, S.; Yu, J.; Sudhanshu, D.; Huimin, Z. Exploiting Issatchenkia Orientalis SD108 for Succinic Acid Production. Microbial Cell Factories 2014, 13, 1–11.
- Qun, W.; Jing, L.; Yan, X. Starter Culture Selection for Making Chinese Seasome-Flavored Liquor Based on Microbial Metabolic Activity in Mixed-Culture Fermentation. Applied and Enviromental Microbiology 2014, 80, 4450–4459.
- Oro, L.; Ciani, M.; Comitini, F. Antimicrobial Activity of Metschnikowia Pulcherrima on Wine Yeasts. Journal of Applied Microbiology 2014, 116 (5), 1209–1217.
- Sezai, T.; Beyza, E. Isolation and Characterization of New Metschnikowia Pulcherrima Strains as Producers of the Antimicrobial Pigment Pulcherrimin. Verlag Der Zeitschrift Für Naturforschung 2009, 64c, 405–410.
- Bulut, S.; Elibol, M.; Ozer, D. Effect of Different Carbon Sources on L(+) -Lactic Acid Production by Rhizopus Oryzae. Biochemical Engineering Journal 2004, 21 (1), 33–37.
- Seungbeon, H.; Daeho, K.; Mina, L.; Seongyeol, B.; Soomwo, K.; Jos, H.; Robert, A. S. Aspergillus Associated with Meju, a Fermented Soybean Starting Material for Traditional Soy Sauce and Soybean Paste in Korea. Mycobiology 2015, 43 (3), 218–224.
- Katsuichi, S.; Ayumi, A.; I-Nengah, S.; Teruo, S.; Yuji, O. Comparison of Amylomyces Rouxii and Rhizopus Oryzae in Lactic Acid Fermentation of Potato Pulp. Food Science Technology Research 2004, 10 (2), 224–226.
- Carolina, H. P.; Alfred, B.; Johan, L. F. K.; Santosh, N. Oxylipin Formation in Fungi: Biotransformation of Arachidonic Acid to 3-Hydroxy-5,8-Tetradecadienoic Acid by Mucor Genevensis B. Biochemical & Biophysical Research Communications 1998, 253 (3), 703–706.
- Zhuying, L.;. Experiment Comparison of Manual Mucor Inoculation and Natural Mucor Inoculation in Fermented Bean Curd Production. China Brewing 2008, 18 (195), 72–72.
- Carlaluciana, G.; Torres, M. J.; Gener, M. V.; Graciela, R. Control of Spoilage Fungi by Lactic Acid Bacteria. Biological Control 2013, 64 (3), 231–237.
- Suisheng, H.; Beck, J. B.; Siovbouy, L. S.; Gee, W. The Major Volatile Compound 2-Phenylethanol from the Biocontrol Yeast, Pichia Anomala, Inhibits Growth and Expression of Aflatoxin Biosynthetic Genes of Aspergillus Flavus. Mycotoxin Research 2014, 30 (2), 71–78.
- Jianhao, Z.; Guanghong, Z.; Jianhui, Z.; Aifeng, M. Changes of Free Amino Acid and Volatile Flavor Compound in the Process of Aging of Broadbean Sauce. China Condiment 2006, 9 (331), 21–25.
- Feng, Y.; Cui, C.; Zhao, H.; Gao, X.; Zhao, M.; Sun, W. Effect Ofkojifermentation on Generation of Volatile Compounds in Soy Sauce Production. International Journal of Food Science & Technology 2013, 48 (3), 609–619.
- Yinsoon, M.; Tingjang, L.; Chunchou, C. Volatile Components of the Enzyme-Ripened Sufu, a Chinese Traditional Fermented Product of Soy Bean. Journal of Bioscience & Bioengineering 2012, 113 (2), 196–201.
- Jianxin, Z.; Xiaojun, D.; Xiaoming, L.; Hao, Z.; Jian, T.; Wei, C. Comparison of Aroma Compounds in Naturally Fermented and Inoculated Chinese Soybean Pastes by GC-MS and GC-Olfactometry Analysis. Food Control 2011, 22, 1008–1013.
- Coghe, S.; Benoot, K.; Delvaux, F.; Vanderhaegen, B.; Delvaux, F. R. Ferulic Acid Release and 4-Vinylguaiacol Formation during Brewing and Fermentation: Indications for Feruloyl Esterase Activity in Saccharomyces Cerevisiae. Journal of Agricultural and Food Chemistry 2004, 52 (3), 602–608.
- Xia, Z.; Zhifang, W.; Shengchao, Z.; Cheng, H.; Wenxue, Z. Phylogenetic Analysis of 18S rDNA Sequence of Mold from Luzhou-Flavor Daqu. Chinese Journal of Applied & Environmental Biology 2012, 17 (3), 334–337.
- Suezawa, Y.; Suzuki, M. Bioconversion of Ferulic Acid to 4-Vinylguaiacol and 4-Ethylguaiacol and of 4-Vinylguaiacol to 4-Ethylguaiacol by Halotolerant Yeasts Belonging to the Genus Candida. Bioscience, Biotechnology, and Biochemistry 2007, 71 (4), 1058–1062.
- Xiaowei, Z.; Yan, Z.; Beizhong, H.; Marcel, H. Z.; Robert, A. S.; Teun, B.; Robert N, M. J. Complex Microbiota of a Chinese “Fen” Liquor Fermentation Starter (Fen-Daqu), Revealed by Culture-Dependent and Culture-Independent Methods. Food Microbiology 2012, 31 (2), 293–300.
- Hau Yin, C.; Puikwan, F.; Joo-Shin, K. Aroma Impact Components in Commercial Plain Sufu. Journal of Agricultural and Food Chemistry 2005, 53, 1684–1691.
- Shuang, C.; Yan, X.; Qian Michael, C. Q. Aroma Characterization of Chinese Rice Wine by Gas Chromatography-Olfactometry, Chemical Quantitative Analysis, and Aroma Reconstitution. Journal of Agricultural and Food Chemistry 2013, 61 (47), 11295–11302.
- Susan, M. F.; Bertil, K. O. Alkypyrazines, Volatiles Formed in the Maillard Reaction. Determination of Odour Detection Thresholds and Odour Intensity Functions by Dynamic Olfactometry. Chemical Senses 1985, 10 (3), 287–296.