ABSTRACT
The knowledge of the effect of polysaccharide addition on the textural properties of milk protein gels is important for foodstuff engineering design. Therefore, the microstructure, texture, and water-holding capacity of rennet-induced milk protein gel with and without the addition of different concentrations of guar gum (GG) were determined. It was found that the presence of GG changed the microstructure of rennet-induced milk gel. The addition of a GG concentration higher than 0.075% w/v led to a discontinuous protein network. Changes in the structure of the gel samples are reflected in the texture perception and their capability of water retention. When a limit GG concentration of 0.15% w/v was exceeded, a very weak gel sample was obtained. The results demonstrated that different milk protein gel microstructures can be created by the addition of different concentrations of GG.
Introduction
Milk protein gel (MPG) formation is a crucial first step in the manufacturing of cheese, yoghurt, and many other dairy products. MPGs are generally classified according to the way in which they are formed: by enzyme action, by acidification, or by a combination of both. Rennet-induced gels are formed by the addition of rennet that hydrolyses the C-terminal region of the ҡ-casein, thereby reducing electrostatic and steric stabilization of the casein micelles (CM) and inducing the spontaneous aggregation of proteolized CM.[Citation1,Citation2]
Many polysaccharides are added to different dairy products (cheese, yoghurt, ice cream) because they render a desirable texture due to their stabilizing, thickening, and gelling properties[Citation3] or because they can be used as fat replacers.[Citation4,Citation5] The characteristics of the final products emerge as a consequence of the effect of the polysaccharide on their microstructure.
Texture, defined as a sensory property by the International Organization for Standardization, is one of the basic quality determinators for fermented dairy products, particularly yoghurt and spread cheese.[Citation6] Instrumental texture analysis, coming from penetrometric methods, is one of the main methods used for texture determination. In addition, the ability of gels to immobilize water represents another important structural property of coagulated dairy products. The final texture of dairy products as well as their susceptibility to syneresis are closely related to the microstructure, especially the structure of the protein matrix.[Citation7,Citation8]
CM plays a major role in imparting structure to dairy products. During CM coagulation, the CM network initially formed in the aggregation step undergoes structural changes, leading to the shrinkage of the gel by rearrangement of the interparticle interactions in the following step of the process.[Citation9] The degree of compactness of gels depends on this rearrangement of the interparticle interactions. When gel formation is fast, these arrangements are partial and, in consequence, the gels formed become less compact. If the gelation process is slowly performed, the gel network can be restructured by breaking of some interactions and the forming of new ones, producing a tighter network with pores progressively smaller.[Citation10]
The gel hardness or firmness and water-holding capacity (WHC) are correlated with protein network connectivity, while the serum release during deformation and WHC are correlated with gel permeability.[Citation11] Pugnaloni et al. showed that a decrease in pore size implies an enhanced interconnectivity of the network, which increases the gel rigidity in sodium caseinate gels.[Citation12] For whey protein gels, as pore sizes increase, gel permeability increases and the WHC of the gel decreases.[Citation13] In cheese making, gel firmness at cutting should be optimized so as to reduce the loss of fat and protein from the curd particles into the whey. If the coagulum is too soft, extensive shattering will occur with high losses of fat and protein in the whey. If the coagulum is too firm, the coagulum may be difficult to cut using the usual equipment.[Citation14]
Polysaccharides used in dairy products are mostly galactomannans (GM) composed by a linear backbone of (1–4) linked β-d-mannose residues substituted by single (1–6)-α-d galactose residues. One of the most popular GM is guar gum (GG) extracted from Cyamopsis tetragonoloba, which grows in Pakistan, India, and the USA, and whose mannose to galactose ratio is 2:1.[Citation15,Citation16]
There are previous reports on the physicochemical interactions between milk protein (MP) and GG mixtures. Bourriot et al.[Citation17] and Tuinier et al.[Citation18] have found that systems composed by MPs and polysaccharides, such as GG, are usually incompatible due to a depletion–flocculation phenomenon. Mixing GG with MP upon a given concentration leads to phase separation. Therefore, formulations containing non-interacting polysaccharides, such as MP and GG, need to be carefully controlled since this segregative phase interaction affects the resulting products.[Citation19] In the last few years, there has been an increasing interest in the study of the non-interacting polysaccharides effect on MP interactions due to the facts that these metastable systems may show potential to develop novel microstructures and textures in dairy products.[Citation19]
The microstructures resulting from the interplay between phase separation due to depletion mechanisms and acid-induced protein gelation were investigated by Rohart et al.[Citation20,Citation21] for mixtures of GG and MP. They have reported that a broad range of defined microstructures can be created by arresting the phase-separating structure by acid gelation.
Previous studies of model renneted skim milk/biopolymer mixtures showed that the addition of GG influenced the viscoelastic properties of rennet-induced skim milk gels. Elasticity, aggregation, and gelation time decreased with increasing amounts of added GG. In addition, the syneresis of rennet-induced skim milk gels increased when GG was added. These changes in the rheological properties and syneresis may be attributed to changes in the microstructure of the rennet-induced skim milk gel in the presence of GG.[Citation22] However, the effect of different concentrations of GG on MP gelation has not yet been reported for the enzymatic gelation of MP–GG mixed system.
Taking all this into account, it is of paramount importance to know the effect of an additive on the microstructural and textural characteristics of protein–polysaccharide mixed gel systems in order to develop dairy products with new specific properties since these attributes would impact on their sensory perception and functionality. Therefore, the aim of this work was to study the effect of the addition of different GG concentrations on rennet-induced MPG microstructure and how these structural changes affect their textural characteristics.
Materials and methods
Materials
Reconstituted MP suspension was prepared from commercial skim dried milk (Nestlé SA, Switzerland) reconstituted to 20% w/v in 5 mM CaCl2 (Merck, Germany). Sodium azide (Mallinckrodt Ch.W, USA) was added in all the cases as a preservative at a range of 0.01–0.02% w/v. Suspensions were held for 24 h at 4°C before use. MP concentration was determined by the Kuaye’s method, which is based on the ability of strong alkaline solutions to shift the spectrum of the amino acid tyrosine to higher wavelength values in the UV region.[Citation23] Commercial liquid rennet was donated by C.O.T.A.R. S.A. (Santa Fe, Argentina). GG was purchased from Sigma-Aldrich (St. Louis, USA), and 0.5% w/v solutions were prepared by dissolving the commercial product in distilled water at room temperature.
Rennet-induced MPGs preparation
The MP and GG solutions were mixed in such a way so as to obtain samples with a final concentration of 3.5% w/v and 0–0.2% w/v, respectively. Rennet-induced MPG was prepared by adding rennet (2.7 RU) to the MP/GG mixtures at a ratio of 5μL . mL−1 at a controlled temperature of 35°C.
Microstructure
The microstructure of MPG was observed by using confocal scanning laser microscopy (CSLM). Rhodamine B (Sigma-Aldrich, St. Louis, USA) was added to samples of MP with and without GG to a final concentration of 0.002 mg . mL−1. After the rennet addition, each sample (100 μm) was immediately placed in a compartment of LAB-TEK II cells, where the gelation reaction takes place at 35°C. Z-stacks of xy-scans were recorded with a z plane total displacement of 15 μm (interval of 0.1 μm). Each sample stack was captured by using a confocal microscopy (Nikon Eclipse TE-2000-E, Japan), with an objective magnification of 60× (oil immersion lens) and a numerical aperture of 1.4. The digital images were acquired with a pixel resolution of 1024 × 1024 and stored in a TIFF format in order to be analysed.
Image texture parameters and pore size determinations
Digital image analysis techniques are helpful tools to study the microstructure features of MPG. In order to study the effect of the GG on the MPG microstructure, two digital analyses of the CLSM images were performed in this work: textural image parameter and pore size diameter.
The concept of texture from a computational point of view differs from the one in the food industry. In the latter case, food texture is usually referred to as the manner in which the human mouth behaves, while image texture is generally characterized by the spatial arrangement of the brightness values of the pixels in a region of the image.[Citation24] An image J plugin, called “Grey Level Co-occurrence Matrix Texture” (version 0.4), was used in order to obtain image texture parameters. This is one of the most commonly used statistical texture analysis methods in which texture features are calculated from the co-occurrence matrix.[Citation24] In this work, two textural parameters were estimated: entropy (E) and homogeneity (H). The value of H is related to the spatial distribution of the grey levels, and it is maximal for an image in which there are few grey-level transitions and decreases from there. In contrast, E is a measurement of the variability of the histogram of grey and is maximal for an image that contains all the shades of grey with equal probabilities.[Citation25] Gel pore size distribution from the CSLM stack images was determined using a plugin of the image J (version 1.485) program, called “Bone J” (version 1.3.12).[Citation26,Citation27] This is a free open-source tool to estimate a volume-based local thickness by fitting maximal spheres to every point in the structure. Local thickness at a given point in the structure is defined as the diameter of the largest sphere which includes that point and which can be completely fitted inside the structure. Since the method is not model-based, assumptions on the structural type are no necessary. Thus it is possible to assess the size distribution of the pores by determining the thickness parameter.[Citation28]
Instrumental texture analysis
Texture measurements were performed by using a motorized test frame Mecmesin Multitest 2.5-d (Mecmesin, Spain) equipped with a dynamometer of a 25 N load cell. Gel samples were prepared in 20 mL cylindrical containers (diameter: 35 mm; height: 30 mm). Five independent repetitions were made for each sample at room temperature. The cylindrical plunger (diameter: 20 mm; height: 20 mm) penetrated the gel mesh during a total displacement of 10 mm. Two parameters were obtained from the force–displacement curves: (a) breaking force (N) and (b) firmness (N⋅mm−1), defined as the force at the first significant break in the curve and the initial slope of the penetration curve within the first 2 mm, respectively.[Citation29]
WHC determination
The measurement was performed according to the methodology proposed by Pang et al.[Citation29] for the determination of WHC of acid MPGs, with some modifications. MPGs with or without GG were formed in 15 mL centrifuge tubes, according to the protocol described in 'Rennet-induced MPGs preparation' section. Samples were centrifuged at 200 g for 10 min at room temperature after 1 h storage at 35°C. The serum expelled (SE) in each tube was quantified. WHC was defined as WHC (%) = 100*[(MPG weight – SE weight)/MPG weight].
Statistical analysis
All determinations were performed at least in duplicate. ANOVA was performed in all the experimental determinations. Differences of p ˂ 0.05 were considered to be significant.
Results and discussion
Gel microstructure
The microstructure of MP/GG mixed gels was visualized by CLSM. A representative image of each gel sample is shown in . The protein network, stained with rhodamine B, is seen as bright areas while dark areas represent the non-protein phase (a mix of serum and GG). A continuous branched protein network structure was observed in the MP gel without GG addition and in the presence of GG concentrations <0.075% w/v. On the other hand, large pores and a less interconnected protein network structure were observed in samples containing more than 0.075% w/v of GG. When GG concentration exceeded 0.15% w/v, the protein mesh was no longer continuous. Finally, at 0.2% w/v GG concentration, non-connecting rich protein droplets dispersed in an aqueous phase could be detected. This had been reported earlier by some authors who studied other kind of micro-phase-separated food gels.[Citation30,Citation31]
Figure 1. (A) Digital images of MP/GG mixed gels obtained by CLSM at different GG concentrations (0.0000–0.2000% w/v). The scale bars represent 20 μm. (B) z-stack image of MP gel without GG addition. (C) z-stack image of MP/GG mixed gel (0.2% w/v GG).
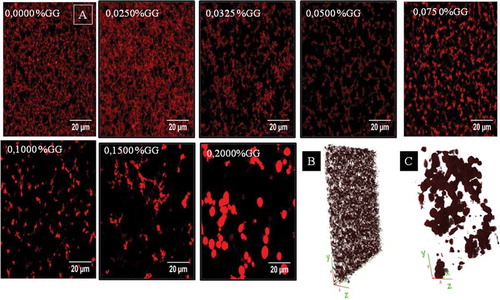
Rohart et al.[Citation20,Citation21] have previously reported about the combined effect of phase separation and gelation of milk–GG mixtures for acid-induced gelation of milk with a previous thermal treatment and 0.3% GG concentration. They controlled the kinetics of both processes by using various stages of temperature. However, no similar studies of the effect of the relative concentration of MP and GG on the enzymatic coagulation process have been found in the literature.
shows the variations of the textural parameters E and H among the rennet-induced MPG samples added with different GG concentrations. When GG concentration in the mixed gels exceeded 0.025% w/v, an increase in the H value and a decrease in the E value were obtained. These effects correspond to a transition of the gels from a homogeneous protein network distribution to a heterogeneous one. In the latter case, the particles were in well-defined sectors of the images. This heterogeneous distribution of particles is consistent with a micro-phase separation in which the protein and the polysaccharide are separated in local areas as GG concentration increases above 0.025% w/v. As GG concentration increases, micro-phase separation becomes more marked, resulting in a discontinuous protein phase immersed in a GG continuous phase at the highest polysaccharide concentration. The textural analysis of images enables us to estimate the added GG concentration at which a difference in the microstructure starts to occur. In this case, we could postulate that a GG addition higher than 0.025% w/v induces a change in the MPG microstructure, as a consequence of the depletion–flocculation mechanism reported earlier by Buriot et al.[Citation17] In such mechanism, when GG chains are added to the MP suspension, they are excluded from the space between the micelles. The incompatibility among the biopolymers causes the increase in the local biopolymer concentration in each phase and the formation of a depleted layer around the CM with an osmotic pressure gradient which makes the CM attract one another.[Citation19,Citation32] The energetic barrier which stabilizes the CM in the suspension through steric and electrostatic repulsions would be then crossed over, leading to the aggregation of the micelles. Therefore, during the enzymatic coagulation process of MP, a competition between protein–GG phase separation and MPG formation would take place. The first one would be favoured at high GG concentrations, whereas the second one would predominate in the absence of GG or at low concentrations of this polysaccharide.
Figure 2. Texture parameters obtained from the CLSM digital images as a function of the GG concentration (0–0.2% w/v).
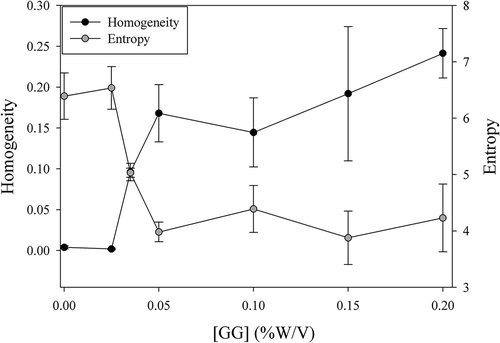
shows the box plots obtained from the pore size distributions of the gel samples analysed. As GG concentration increased, an increase in pore size was obtained for the MPG samples after the addition of GG concentrations >0.05. Larger pores emerge as a consequence of the depletion–flocculation effect that causes a less connected MP network. Several articles, including that by Spotti et al., reveal that the pores of gels formed by phase-separating systems (composed of a gelling protein and a polysaccharide) are larger than the pores of gels formed in the absence of the polysaccharide (Çakır et al., 2012; Çakır & Foegeding, 2011; Sanchez, Zuniga-Lopez, Schmitt, Despond, & Hardy, 2000; Spotti, Santiago, Rubiolo, & Carrara, 2012). On the other hand, in the presence of 0.15% and 0.2% w/v GG concentrations, it was not possible to estimate the gel pore size distribution because the microstructure changed from a protein continuous matrix to an isolated protein sector in a continuous non-protein phase (C). Therefore, the presence of GG at these concentrations would inhibit protein gel formation.
Texture analysis
shows the profile of the penetration curve in relation to the amount of GG in MPG. All force–displacement curves showed a sharp increase in the force over a short distance as the probe moved into the samples. The initial slope was different for each GG concentration analysed, except for the 0.15% and 0.2% w/v GG. shows the firmness and the force at break value for each gel sample. MPG firmness remained constant with the addition of GG in the range of 0.0–0.025% w/v, increased with further addition of GG until a concentration of 0.05%, whereas this parameter decreased at higher GG concentrations. A low firmness value indicates that the gels are easily deformed and tend to flow rather than break.[Citation33] These results are in agreement with those reported by Pang et al.[Citation34] for GG effect on textural properties of acid milk gels. In addition, another important characteristic that could be observed from penetrometry curves is the breaking point, which is a measure of the fracturability of the gel (force value of the plateau). A low breaking force at fracture indicates high fracturability. MPG showed a decrease in their fracturability value as GG concentration increased to 0.1% w/v. At higher GG concentrations, the absence of breaking point was observed.
Table 1. Penetration parameters (firmness and force at break) of rennet-induced milk protein gels (MP 20% w/v, GG 0–0.2000% w/v).
WHC determinations
shows the WHC results of rennet-induced MP/GG mixed gels. Additions of low concentration (˂0.05% w/v) of GG did not change the WHC values obtained. On the other hand, the addition of higher concentrations of GG led to lower WHC values. This is related to the microstructure found for the MPG and to the textural determinations since higher GG concentrations added to the MP systems led to a less interconnected protein network (large pore size) and less firmness values of the gels.
Figure 5. Water-holding capacity as a function of GG concentration for rennet-induced MP/GG mixed gels. The dotted line represents the result obtained for the gel sample without GG addition.
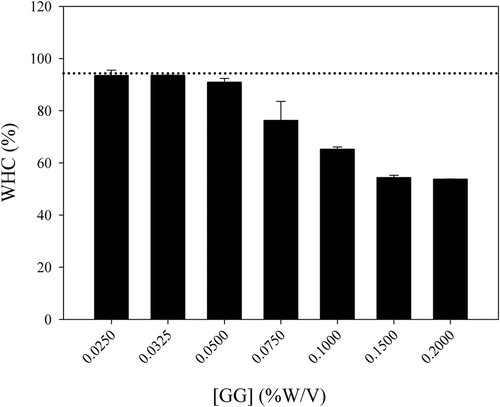
Foegeding et al.[Citation35] have reported that the use of MPs in combination with polysaccharides to alter structure by phase separation and to form cold-set gels with a series of microstructures (homogeneous, course stranded, bicontinuous, and protein continuous) allows the formation of gels with different textures, as well as design structures that either hold or release water.
Conclusion
Although the use of polysaccharides such as GG during acid-induced gelation in GG/milk mixture has received attention in the last years, little is known about the use of this polysaccharide during the rennet-induced gelation of MP. This study was undertaken to gain a better understanding of the combined effects of phase separation and gelation on the structure of MPG. Confocal laser microscopy, image analyses, textural and WHC approaches were found to be useful in determining the effect of the presence of GG on MPG characteristics. The presence of GG during the rennet-induced gelation increased the complexity in MP systems. The addition of GG induced changes in the MPG microstructure which were reflected in changes in texture and WHC. At low GG concentration (≤0.05% w/v), the microstructure of MPG was characterized by a protein continuous network and a discontinuous non-protein (serum and GG) phase. The gels were firmer and the WHC obtained was not altered in such range of GG concentration, in comparison with the MPG in the absence of GG. When GG concentration exceeded 0.075% w/v, the effect of the incompatibility of the two biopolymers became more important. As a consequence, the protein mesh was less interconnected and discontinuous. In addition, the firmness and the WHC were lower for these gel samples. Finally, when GG concentration exceeded 0.15% w/v, the protein phase was no longer continuous. As a result, a very weak gel is obtained since the addition of GG above this limit concentration results in the inhibition of the gel formation in the experimental conditions assayed. This effect on the gel formation of GG is a consequence of the mechanism of depletion–flocculation occurring among both biopolymers that it is predominant over the gel formation kinetics.
Acknowledgements
Micaela Galante would like to thank Consejo Nacional de Investigaciones Científicas y Técnicas (CONICET) for the fellowships. The authors would like to thank to the English Area of Facultad de Ciencias Bioquímicas y Farmacéuticas (UNR) for the language correction of the manuscript and to those who provided financial support CONICET (PIP 11220130100076CO) and UNR (1BIO368).
References
- Van Vliet, T.; Lakemond, C. M.; Visschers, R. W. Rheology and Structure of Milk Protein Gels. Current Opinion in Colloid & Interface Science 2004, 9(5), 298–304.
- Sinaga, H.; Bansal, N.; Bhandari, B. Gelation Properties of Partially Renneted Milk. International Journal of Food Properties 2017, 20(8), 1700–1714.
- Williams, P.; Phillips, G.; McKenna, B. The Use of Hydrocolloids to Improve Food Texture. In Texture in Food.; McKenna, B. M.; Ed.; Woodhead Publishing: Cambridge, England; Volume 1: Semi-Solid Foods, 2003; 251–274.
- Javidi, F.; et al. The Influence of Basil Seed Gum, Guar Gum and Their Blend on the Rheological, Physical and Sensory Properties of Low Fat Ice Cream. Food Hydrocolloids 2016, 52, 625–633.
- Liu, L.; Li, C.; Liu, J. Rheological and Physical Characteristics of Non-Fat Set Yogurt Prepared with EPS-producing Streptococcus Thermophilus and an H+-ATPase-defective Mutant Lactobacillus Delbrueckii Subsp. Bulgaricus. International Journal of Food Properties 2017, 20(4), 745–753.
- Szczesniak, A. S. Texture Is a Sensory Property. Food Quality and Preference 2002, 13(4), 215–225.
- Domagała, J. Instrumental Texture, Syneresis and Microstructure of Yoghurts Prepared from Goat, Cow and Sheep Milk. International Journal of Food Properties 2009, 12(3), 605–615.
- Domagała, J. Instrumental Texture, Syneresis, and Microstructure of Yoghurts Prepared from Ultrafiltrated Goat Milk: Effect of Degree of Concentration. International Journal of Food Properties 2012, 15(3), 558–568.
- Risso, P.; et al. Effect of Size, Proteic Composition, and Heat Treatment on the Colloidal Stability of Proteolyzed Bovine Casein Micelles. Colloid & Polymer Science 2007, 285(7), 809–817.
- Ingrassia, R.; et al. Application of a Digital Image Procedure to Evaluate Microstructure of Caseinate and Soy Protein Acid Gels. LWT - Food Science and Technology 2013, 53(1), 120–127.
- Pascua, Y.; Koç, H.; Foegeding, E. A. Food Structure: Roles of Mechanical Properties and Oral Processing in Determining Sensory Texture of Soft Materials. Current Opinion in Colloid & Interface Science 2013, 18(4), 324–333.
- Pugnaloni, L. A.; Matia-Merino, L.; Dickinson, E. Microstructure of Acid-Induced Caseinate Gels Containing Sucrose: Quantification from Confocal Microscopy and Image Analysis. Colloids and Surfaces B: Biointerfaces 2005, 42(3–4), 211–217.
- Verheul, M.; Roefs, S. P. Structure of Whey Protein Gels, Studied by Permeability, Scanning Electron Microscopy and Rheology. Food Hydrocolloids 1998, 12, 17–24.
- Fox, P. F.; Cogan, T. M.; Guinee, T. P. Factors that Affect the Quality of Cheese. In Cheese; McSweeney, P. L. H.; Fox, P. F.; Cotter, P.; Everett, D. W.; Eds.; Academic Press: San Diego, 2017; 617–641.
- Lapasin, R.; Pricl, S. Rheology of Polysaccharide Systems, in Rheology of Industrial Polysaccharides: Theory and Applications; Springer US: Boston, MA, 1995; 250–494.
- Yu, K.; et al. Guar Gum. In Analytical Profiles of Drug Substances and Excipients; Harry, G. B. Editor; Academic Press: New Jersey, 1996; 243–276.
- Bourriot, S.; Garnier, C.; Doublier, J.-L. Phase Separation, Rheology and Microstructure of Micellar Casein–Guar Gum Mixtures. Food Hydrocolloids 1999, 13(1), 43–49.
- Tuinier, R.; Ten Grotenhuis, E.; De Kruif, C. The Effect of Depolymerised Guar Gum on the Stability of Skim Milk. Food Hydrocolloids 2000, 14(1), 1–7.
- Corredig, M.; Sharafbafi, N.; Kristo, E. Polysaccharide–Protein Interactions in Dairy Matrices, Control and Design of Structures. Food Hydrocolloids 2011, 25(8), 1833–1841.
- Rohart, A.; Moulin, G.; Michon, C. Interplay between Phase Separation and Gel Formation in Stirred Acid Milk/Guar Gum Gels: Effect of Acidification Rate. Biopolymers 2014, 101(9), 966–974.
- Rohart, A.; Michon, C. Effect of Thermomechanical Treatment on Microstructure of Guar Gum/Acid Milk Gels. Journal of Food Engineering 2016, 171, 14–21.
- Tan, Y.; et al. Effects of Biopolymer Addition on the Dynamic Rheology and Microstructure of Renneted Skim Milk Systems. Journal of Texture Studies 2007, 38(3), 404–422.
- Kuaye, A. Y. An Ultraviolet Spectrophotometric Method to Determine Milk Protein Content in Alkaline Medium. Food Chemistry 1994, 49(2), 207–211.
- Zheng, C.; Sun, D.-W.; Zheng, L. Recent Applications of Image Texture for Evaluation of Food Qualities—A Review. Trends in Food Science & Technology 2006, 17(3), 113–128.
- Haralick, R. M.; Shanmugam, K.; Dinstein, I. H. Textural Features for Image Classification. Systems, Man and Cybernetics, IEEE Transactions On 1973, 3(6), 610–621.
- Doube, M.; et al. BoneJ: Free and Extensible Bone Image Analysis in ImageJ. Bone 2010, 47(6), 1076–1079.
- Hildebrand, T.; Rüegsegger, P. A New Method for the Model‐Independent Assessment of Thickness in Three‐Dimensional Images. Journal of Microscopy 1997, 185(1), 67–75.
- Abd-Rabou, N.; et al. Properties of Edam Cheese Fortified by Dietary Zinc Salts. Journal of American Science 2010, 6(10), 441–446.
- Pang, Z.; et al. Effect of Addition of Gelatin on the Rheological and Microstructural Properties of Acid Milk Protein Gels. Food Hydrocolloids 2015, 43, 340–351.
- De Jong, S.; Van De Velde, F. Charge Density of Polysaccharide Controls Microstructure and Large Deformation Properties of Mixed Gels. Food Hydrocolloids 2007, 21(7), 1172–1187.
- Hidalgo, M. E.; et al. Acid-Induced Aggregation and Gelation of Sodium Caseinate-Guar Gum Mixtures. Food Biophysics, 2015; 10(2),181–194.
- Monteiro, S. R.; et al. The Influence of Galactomannans with Different Amount of Galactose Side Chains on the Gelation of Soy Proteins at Neutral pH. Food Hydrocolloids 2013, 33(2), 349–360.
- Pang, Z.; et al. Rheology, Texture and Microstructure of Gelatin Gels with and without Milk Proteins. Food Hydrocolloids 2014, 35, 484–493.
- Pang, Z.; Deeth, H.; Bansal, N. Effect of Polysaccharides with Different Ionic Charge on the Rheological, Microstructural and Textural Properties of Acid Milk Gels. Food Research International 2015, 72, 62–73.
- Foegeding, A. E.; Çakır, E.; Koç, H. Using Dairy Ingredients to Alter Texture of Foods: Implications Based on Oral Processing Considerations. International Dairy Journal 2010, 20(9), 562–570.