ABSTRACT
The effects of two cross-linking agents (genipin and polyphenol) on the physical properties of gelatin hydrogels were studied to determine the more effective natural cross-linking agent. The highest gel strength obtained with polyphenols was 136.9 N/mm2 at concentrations up to 360 μg polyphenols/g gelatin, whereas the highest strength of samples cross-linked with genipin was 98.4 N/mm2 with 300 mg genipin/g dry gelatin. Scanning electron microscopy showed that gelatin modified by polyphenols at 360 μg/g gelatin had the most compact surface. Cross-linked gelatin-polyphenol exhibited a markedly enhanced denaturation temperature and greater thermal stability than gelatin–genipin.
Introduction
Gelatin is mainly obtained by the physical and chemical degradation or thermal denaturation of collagen. It has been widely used as a biomaterial for hard and soft capsules, microspheres, tissue adhesives, and carriers for drug-delivery systems. Gelatin is also an important gelling biopolymer widely used in foods to provide elasticity, viscosity, and stability. Compared with collagen, gelatin has low antigenicity under physiological conditions, is relatively inexpensive, and is easier to produce different concentrations in solution; moreover, its physicochemical properties can be easily modulated. [Citation1] However, the poor mechanical properties and thermal stability of gelatin limit its possible applications as a fundamental component during food processing. [Citation2] Because it contains a large number of functional groups (such as amino groups), gelatin is often modified for practical uses by chemical and physical methods, such as UV- and γ-irradiation, multivalent metal ions, and aldehyde cross-linkers. [Citation3] However, UV-irradiation only modifies the surface of gelatin, and the toxicity of aldehyde compounds limits their use in biomaterial production and application. [Citation4] As shown in a previous study, genipin is a naturally occurring cross-linker that can cross-link gelatin and collagen. [Citation5] However, genipin has some disadvantages including high cost, difficulties with its extraction, and few available sources. [Citation6] In particular, when collagen biomaterials are combined with genipin, a dark blue pigment spontaneously forms during the reaction, which limits the use of genipin. [Citation7] Therefore, it is necessary to find a novel cross-linking agent that is ubiquitous and has excellent biocompatibility. Genipin is a non-toxic cross-linker and is widely used in a variety of applications; therefore, this study aims to compare another cross-linker, polyphenol, with genipin to determine the more effective cross-linker.
Plant polyphenols are a diverse and important group of compounds, many of which occur in a range of plant-derived foods such as coffee beans, plant leaves, and fruit walls. [Citation8] Polyphenols contain sufficient hydroxyl groups and other groups, such as carboxyl groups, which can effectively cross-link with peptides under certain conditions. [Citation9] Due to their antioxidant activity and high compatibility with proteins, the interest in polyphenol use in pharmacological applications and functional foods has increased. The biological properties (free-radical scavenging capacity, pharmacological activity, and high affinity to proteins) of plant polyphenols have been largely confirmed. [Citation10] The polyphenols from Fructus Chebulae contain pyrogallol or hydrolysable tannins, which have phenol carboxylic acids with a high nutritional value. These compounds have been shown to be useful in biological and pharmacological applications. Since the use of gelatin in food packaging and biomaterials is increasing, understanding the role of polyphenols from Fructus Chebulae in gel stability is important for developing new cross-linkers and the production of new functional materials.
Compared to genipin, polyphenols have a broader selection of sources and are easier to extract from plants. However, very few reports have examined whether the polyphenols extracted from Fructus Chebulae have the ability to stabilize gelatin. In the present study, the polyphenols from Fructus Chebulae were used as cross-linkers, and their ability to modify gelatin hydrogels were compared with that of genipin. This study focused on understanding the gel behavior and physicochemical properties of gelatins that were modified by these two cross-linking agents, genipin and polyphenols obtained from Fructus Chebulae. The concentrations of genipin used in these experiments were in agreement with previously reported studies. [Citation1]
The objective of this research was to compare gelatin–polyphenol interactions with gelatin–genipin interactions to determine whether polyphenols could be an effective cross-linking agent. The structure and properties of the hydrogels were characterized based on gel strength, swelling properties, thermal stability, degree of cross-linking, Fourier transform infrared (FT-IR) spectroscopy, and scanning electron microscopy (SEM).
Materials and methods
Materials
Gelatin (type B, bovine bone) was purchased from Qing Dao Biotechnology (Qing Dao, China). Genipin and the Folin–Ciocalteu regent were obtained from Sigma Chemical Co. NaHCO3, HCl, and 2,4,6-trinitrobenzenesulfonic acid (TNBS) were of analytical grade. Plant materials were obtained from local stores.
Preparation of polyphenols from fructus chebulae extracts
Plant extracts were prepared with water as decoctions where 1 g of minced, dried Fructus Chebulae was sequentially extracted with 100 mL boiling water and 100 mL of acetone/water (80:20 v/v) for 1 h each. The mixtures were centrifuged at 8000 rpm for 10 min and the resulting supernatants were concentrated by vacuum rotary evaporation at 50°C. The extract was lyophilized and stored at −20°C. Total phenol content (TPC) was determined according to the Folin–Ciocalteu method. [Citation11] TPC was expressed as µg of gallic acid equivalents (GAE) per g of material. The Fructus Chebulae extract used had 360 μg polyphenols/g of total plant extract. Only the approximate concentrations could be determined, because the plant extracts contained many other phenolic compounds that could interfere with the absorbance reading using this method.
Hydrogel sample preparation
For preparations of gelatin gels, lyophilized gelatin was dissolved in distilled water for 15 min and then heated to 50°C with stirring until completely dissolved. Then, the cross-linking agents were dissolved in distilled water and added to the gelatin solution at final concentrations of 240, 360, and 480 μg polyphenols/g dry gelatin and 200, 250, and 300 mg genipin/g dry gelatin. The obtained cross-linked gelatin composites were named CPG1, CPG2, and CPG3 and CGG1, CGG2, and CGG3, respectively. The solutions had a final pH between 5 and 6 were adjusted to the final concentration of 6% and allowed to cross-link for 30 min at 40°C. The resulting mixtures were cooled to room temperature, conditioned at 4°C for 24 h to form hydrogels, and then lyophilized using a vacuum dryer. The resulting freeze-dried samples were used in the following tests.
Measurement of gel strength
The gel strength of the cross-linked hydrogels was determined using the TMS-PRO food texture analyzer (Food Technology Co., Virginia, USA) with a 5-kN load cell. The method by Yan, Li, Zhao, & Yi, was slightly modified, and the measurement of gel strength was performed on hydrogels before freeze-drying. [Citation12] The cylindrical hydrogels for puncturing were placed in the center of the texture analyzer platform and a cylindrical probe with a plunger of 5 mm. The maximum force (in grams) and penetration distance were obtained as the plunger penetrated through the hydrogels. The break force was determined using the following equation:
where σ, F, and r are the break force, the maximum force, and the radius of the cylindrical plunger, respectively. Each sample was tested in triplicate.
Swelling kinetics
The swelling ratios were determined according to the method of Charulatha and Rajaram. [Citation13] Xerogels were weighed in an air-dried condition, and then rehydrated in distilled water at 4°C for 100 min. The samples were blotted with filter paper to remove surface water before weighing every 15 min. The swelling ratio is defined by Eq. 2:
where W0 and Wd are the hydrated weight and the dried weight of the xerogel, respectively.
Differential scanning calorimetry
Calorimetric measurements were performed using a Netzsch differential scanning calorimeter 200 PC (Netzsch, Bavaria, Germany) equipped with a liquid nitrogen cooler at ambient temperature. Approximately 10–12 mg of dried sample was hermetically sealed in an aluminum pan while an empty sealed pan was used as a reference. The thermogram was obtained by heating the chambers from 10 to 150°C at a scanning rate of 5 K/min with a N2 flow of 50 mL/min. [Citation14]
Thermogravimetric analysis
Thermal weight loss of the cross-linked samples was determined using a thermogravimetric analyzer (Netzsch TG 200PC, Germany) transitioning from 20 to 800°C at a heating rate of 10°C/min under nitrogen atmosphere to avoid thermo-oxidation reactions. Approximately, 5 mg of each sample was used for the analyses.
Determination of the degree of cross-linking
The degree of cross-linking was determined using a UV assay to detect uncrosslinked ε-amino groups before and after cross-linking using 2,4,6-trinitrobenzenesulfonic acid (TNBS) as previously described. [Citation15] The relationship between the absorbance and content of ε-amino was calculated from the following Eq. 3:
where 1.46 × 104 L/mol·cm is the molar absorptivity of THP-lys, b is the cell path length in cm, andis the sample weight in g.
FT-IR spectral analyses
The structures of the cross-linked samples were analyzed using a FT-IR (Nicolet 200SXV) spectrometer. The spectra obtained were used to determine the possible interactions between functional groups in the cross-linked gelatin molecules. [Citation16]
Scanning electron microscopy
The morphologies of air-dried hydrogels were observed using a scanning electron microscope (HITACHI S 4300, Japan) with an accelerating voltage of 15 kV as described previously. [Citation17]
Results and discussion
Gel strength
Gel strength is one of the vital functional properties of gels. The physical properties of gelatin mainly depend on the inter- and intra-molecular hydrogen bonds, the triple-helix content, and the molecular weight distribution of the gelatin. [Citation18] However, the low gel strength of natural gelatin limits its use for some specific applications in biomaterials. The changes in gel strength after cross-linking are shown in . The gel strengths of all treated samples were higher compared to the control condition. The most significant increase in the gel strength for polyphenol cross-linked gelatins was found for CPG2, which was 136.9 N/mm2, whereas the highest strength observed for samples cross-linked with genipin (CGG3) was 98.4 N/mm2. The increase in gel strength can be attributed to hydrogen bonds formed by polyphenols, but the mechanical properties of the gel will decrease when the concentration of the polyphenols exceeds a critical value. [Citation19] This may result from precipitation, as previous studies have proved that all of the gelatin or collagen components could be precipitated with polyphenols. This phenomenon could also be explained by a higher number of hydrogen bonds on one chain, which would prevent polyphenols from forming cross-links between chains. These results also demonstrated that the concentration of polyphenols needed in the experiment was much lower than that of genipin and the gel strength of the hydrogel cross-linked by polyphenol was higher. Such a result could be attributed to the higher extent of hydrogen bonding of the molecular structures of polyphenols than that of genipin.
Swelling kinetics
The swelling ratios of xerogels cross-linked by polyphenols and genipin are presented in . The swelling ratios of the control and cross-linked gelatin samples increased quickly for 60 min, and maximum volumes were measured at 90 min. After 90 min, all samples dissolved completely over time (not shown in data). The maximum swelling ratios (MSRs) of the control, CGG2, and CGG3 were 1810.24%, 1690.90%, and 1420.79%, respectively, whereas the MSRs of CPG2 and CPG3 were 849.98% and 669.88%, respectively—both were much lower than the MSRs observed for the genipin cross-linked or control groups. This phenomenon suggested that the swelling ratios of the samples decreased when the cross-linking agent was introduced and that the gelatin treated with polyphenols had a reduced ability to absorb water. Previous studies also showed that cross-linking might decrease the swelling ratio of a gel. Bigi et al. found that the swelling ratio was significantly reduced as the degree of cross-linking increased. [Citation1] They had used GTA (gelatin tea abstract) to modify gelatin, and found that cross-linking decreased the degree of swelling. In this study, polyphenols from Fructus Chebulaewere the preferred natural cross-linkers for gelatin, which was consistent with the conclusions from the gel strength experiments. The ability to entrap water can be controlled by changing the characteristics of the cross-linking agents.
Differential scanning calorimetry measurements
The peak values (Td) of gelatin with or without cross-linking agents (polyphenols and genipin) were computed from the differential scanning calorimetry (DSC) data (). The Td of different gelatin samples is shown in . The value of the endothermic peak is associated with the helix–coil transition of collagen, and the Td is generally related to the denaturation temperature. It is generally accepted that an improvement in Td is favorable for increasing the thermal stability of gelatin composites, which is important for the mechanical properties of gelatin-based materials. [Citation10] The endothermic process presented in the DSC thermogram suggests the rupture of hydrogen bonds and rearrangement of the oriented peptide chains. [Citation20] In addition, these studies on the thermal stability of gelatin modified by cross-linkers are of great interest, because of their applications in biomaterials where the hydrogels may experience sustained heat during their processing, preparation, or consumption. As shown in , the Td values of the cross-linked gelatins shifted toward higher temperatures compared to the value of uncrosslinked gelatin. However, the Td decreased slightly from CPG2 to CPG3, suggesting the high concentration of polyphenols did not significantly increase the thermal stability of the gelatin. The Td of CGG2 and CGG3 show small variations compared with untreated gelatin. This indicates that their interaction is not a simple physical effect. Moreover, the polyphenols may rely on the formation of hydrogen bonds between peptide chains, while genipin may form intra- and inter-molecular cross-links with cyclic structures in the gelatin chains. The different mechanisms used to form cross-links with gelatin could explain why the polyphenol cross-linked samples have a higher denaturation peak. A rise in Td reflects an increase in the number of cross-links per molecule. These data are in agreement with the previous swelling ratio tests, suggesting that the gelatin treated with polyphenols had a better cross-linking network than the genipin-modified gelatin or the untreated gelatin.
Table 1. The Td of different gelatin samples.
Thermogravimetric analysis
The thermal stability of xerogels in the presence and absence of cross-linking agents was assessed by thermogravimetric analysis (TGA). and b show the TGA and DTG of the samples, respectively. The three thermograms had similar variations with the main differences occurring between 20 and 500°C. Initial thermal degradation occurred between 20 and 150°C with a maximum rate at around 65°C, which was related to the loss of adsorbed and bonded water commonly observed with air-dried gelatin. The second stage of weight loss appeared between 200 and 500°C and was associated with degradation and modification of gelatin chains. The higher temperature stage, which exceeded 500°C, was related to the real breakage of more thermally stable structures. Most of the mass loss occurred in the second stage as showed in . A similar result has been reported in gelatin modified with procyanidin. [Citation10] The temperature at 50% of the total mass, the residue at 500°C, the maximum rate of weight loss, and the corresponding temperature (Tm) are also shown in . The cross-linked samples show better thermal stability than pure gelatin. The temperatures corresponding to the maximum rate of weight loss (Tm) increased in the following order: control (315.7°C) < CGG3 (317.2°C) < CPG2 (318.8°C), implying the thermal stability of the samples was improved by inter- and intra-molecular hydrogen bonds. The data showed that the interactions between gelatin and cross-linkers improved the thermal stability. In addition, the gelatins treated with polyphenols were more able to form thermally stable structures.
Table 2. The temperature of 50% mass, the residue at 500ºC, the maximum rate of weight loss, the temperature corresponding to maximum rate of weight loss (Tm) and weight loss of the two defined processes of the xerogels with two cross-linking agents.
Analysis of the degree of cross-linking
The degree of cross-linking of different gelatin samples is provided in . The content of uncrosslinked amino groups in gelatin samples was determined by the reaction of TNBS with amino groups. The data demonstrated an inverse relationship between the number of cross-links and the concentration of the polyphenols when a critical concentration was exceeded. As a cross-linking agent, the polyphenols introduced the maximum amount of cross-linking, while genipin introduced a minimum number of cross-links. The results reported in show that CPG2 is sufficient to cross-link more than 80% of the ε-amino groups, while the degree of cross-linking decreases with increasing concentrations to about 77%. The maximum content of cross-linking induced by genipin was 65% for the concentrations used in our experiments. Previous results from carbodiimide cross-linked collagen indicate that carbodiimide hydrochloride (EDC) is more effective at increasing the number of cross-links. However, it is worth noting that polyphenols can link more free ε-amino groups, even at lower concentrations; free amino groups were still detected at the critical concentration possibly due to masking of residual amino groups during cross-linking when gelatin nanoparticles were formed through inter- and intramolecular electrostatic interactions.
Table 3. Degree of cross-linking, expressed as the percentage of free ε-amino groups lost after cross-linking.
Fourier transform infrared spectroscopy
FT-IR spectra were used to reveal the structures of gelatin in the presence of cross-linking agents, because the FT-IR spectra of gelatin molecules can be correlated directly to their backbone conformation. shows the spectra for untreated gelatin and the complex of gelatin with cross-linkers. As shown in , the band around 3300 cm−1 also shifted to lower frequencies in the treated gelatin to some degree, and it appeared to be broader when compared with untreated gelatin. The variations in the positions of the major amide bonds proved that hydrogen bonding occurred between gelatin and the cross-linking agents. Therefore, it was deduced that hydrogen bonds play important roles in the stabilization of gelatin cross-linked by polyphenols or genipin.
The ratios of amide III/1450 cm−1 are also considered a measure of the extent of the damage to collagen triple helixes. [Citation21] In our case, it is worth nothing that the ratios for the gelatins cross-linked by polyphenols and genipin are 0.85, the same as the ratio of uncrosslinked gelatin, indicating that the cross-linkers (polyphenols and genipin) do not destroy the conformation of the gelatin. As shown in previous studies, the hydrogen bonds corresponding to water were crucial for stabilizing the triple helix structure of collagen. [Citation22] Therefore, it was concluded that hydroxyl-rich cross-linkers could displace water and promote new hydrogen bonding to stabilize the ultrastructure of gelatin. In conclusion, genipin and polyphenols act as cross-linking agents without damaging the ultrastructure of the gelatin.
Scanning electron microscopy
shows the surfaces and cross-sections morphologies of xerogels with or without cross-linkers using SEM. Both the untreated and cross-linked hydrogels were characterized by a smooth, compact, and homogenous surface that was similar to hydrogels composed of ferulic and tannin acids. [Citation23] It is notable that the cross-section of the untreated hydrogel showed porous structures with different and random pore sizes. [Citation24] On the contrary, the images of the cross-sections of hydrogels cross-linked with polyphenols or genipin had an apparent decrease in free volume and were superior to native samples. Polyphenols and genipin cross-linking caused the smoothest cross-linking surface [Citation8], suggesting that residual amine groups in gelatin chains react with polyphenols and genipin to form a dense network structure that limits the movement of the free gelatin chains. [Citation1] These results are in agreement with data on gelatin that was cross-linked with ferulic acid, which also formed a dense network of structures owing to the application of a force. [Citation2] Thus, the mechanical properties and thermal stability of polyphenol cross-linked hydrogels were superior to that of genipin cross-linked hydrogels [Citation15], possibly because more combinations of gelatin chains are formed into a more compact structure. [Citation22] This phenomenon can also be found in other cross-linked biopolymer systems. [Citation21]
Figure 6. Micrographs of the surface and a cross-section of the hydrogels with or without cross-linkers: (a) surface of an untreated xerogel; (b) surface of a polyphenol cross-linked xerogel; (c) surface of a genipin cross-linked xerogel; (d) cross-section of an untreated xerogel; (e) cross-section of a polyphenol cross-linked xerogel; (f) cross-section of a genipin cross-linked xerogel.
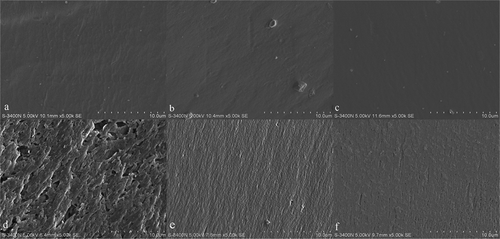
Conclusion
The results obtained regarding properties and structures of gelatins formed by addition of polyphenols or genipin can lead to the following conclusions. Polyphenol cross-linking at a concentration of 360 μg polyphenols/g of gelatin (CPG2) resulted in the highest thermal stability and strongest mechanical properties. Moreover, the use of polyphenols significantly decreased the swelling ratio of the freeze-dried hydrogels. The gelatin modified by genipin had little effect on these properties. The morphologies of the gelatins in the two conditions suggested that polyphenol-modified hydrogels had a more compact surface and a higher degree of cross-linking than genipin-cross-linked and control gels. The FT-IR spectra confirmed that polyphenols and genipin mainly induced hydrogen bond formation, which reinforced the interactions between gelatin chains and did not change the conformation of the gelatin. In addition, the cost of genipin required for the cross-linked samples was much higher than that of polyphenols, because the polyphenols obtained from Fructus Chebulae were more effective cross-linkers. In conclusion, the results above may provide a foundation for developing new and economical cross-linking agents for use in food processing and biomaterial applications.
Additional information
Funding
References
- Bigi, A.; Cojazzi, G.; Panzavolta, S.; Roveri, N.; Rubini, K. Stabilization of Gelatin Films by Crosslinking with Genipin. Biomaterials. 2002, 23, 4827–4832.
- Bigi, A.; Cojazzi, G.; Panzavolta, S.; Rubini, K.; Roveri, N. Mechanical and Thermal Properties of Gelatin Films at Different Degrees of Glutaraldehyde Crosslinking. Biomaterials. 2001, 22, 763–768.
- Sheu, M. T.; Huang, J. C.; Yeh, G. C.; Ho, H. O. Characterization of Collagen Gel Solutions and Collagen Matrices for Cell Culture. Biomaterials. 2001, 22, 1713–1719.
- Wu, K. J.; Wang, C. Y.; Lu, H. K. Effect of Glutaraldehyde on the Humoral Immunogenicity and Structure of Porcine Dermal Collagen Membranes. Arch. Oral Biol. 2004, 49, 305–311.
- Yao, C. H.; Liu, B. S.; Chang, C. J.; Hsu, S. H.; Chen, Y. S. Preparation of Networks of Gelatin and Genipin as Degradable Biomaterials. Mater. Chem. Phys. 2004, 83, 204–208.
- Mekhail, M.; Jahan, K.; Tabrizian, M. Genipin-Crosslinked Chitosan Gels Promote Fibroblast Adhesion and Proliferation. Carbohydr. Polym. 2014, 108, 91–98.
- Yang, X.; Guo, L.; Fan, Y.; Zhang, X. Preparation and Characterization of Macromolecule Cross-Linked Collagen Hydrogels for Chondrocyte Delivery. Int. J. Biol. Macromol. 2013, 61, 487–493.
- Balange, A.; Benjakul, S. Enhancement of Gel Strength of Bigeye Snapper (Priacanthus Tayenus) Surimi Using Oxidised Phenolic Compounds. Food Chem. 2009, 113, 61–70.
- Krishnamoorthy, G.; Madhan, B.; Sadulla, S.; Rao, J. R.; Madhulatha, W. Stabilization of Collagen by the Plant Polyphenolics. J. Appl. Polym. Sci. 2008, 108, 199–205.
- He, L.; Mu, C.; Shi, J.; Zhang, Q.; Shi, B.; Lin, W. Modification of Collagen with a Natural Cross-Linker, Procyanidin. Int. J. Biol. Macromol. 2011, 48, 354–359.
- Singleton, V.; Rossi, J. A. Colorimetry of Total Phenolics with Phosphomolybdic-Phosphotungstic Acid Reagents. Am. J. Roentgenology. 1965, 16, 144–158.
- Yan, M.; Li, B.; Zhao, X.; Yi, J. Physicochemical Properties of Gelatin Gels from Walleye Pollock (Theragra Chalcogramma) Skin Cross-Linked by Gallic Acid and Rutin. Food Hydrocoll. 2011, 25, 907–914.
- Charulatha, V.; Rajaram, A. Influence of Different Crosslinking Treatments on the Physical Properties of Collagen Membranes. Biomaterials. 2003, 24, 759–767.
- Hu, Y.; Liu, L.; Dan, W.; Dan, N.; Gu, Z.; Yu, X. Synergistic Effect of Carbodiimide and Dehydrothermal Crosslinking on Acellular Dermal Matrix. Int. J. Biol. Macromol. 2013, 55, 221–230.
- Bubnis, W. A.; Ofner, C. M., III. The Determination of ϵ-Amino Groups in Soluble and Poorly Soluble Proteinaceous Materials by a Spectrophotometric Method Using Trinitrobenzenesulfonic Acid. Anal. Biochem. 1992, 207, 129–133.
- Krishnamoorthy, G.; Sehgal, P. K.; Mandal, A. B.; Sadulla, S. Novel Collagen Scaffolds Prepared by Using Unnatural D-Amino Acids Assisted EDC/NHS Crosslinking. J. Biomater. Science-Polymer Edition. 2013, 24, 344–364.
- De Carvalho, R.; Grosso, C. Characterization of Gelatin Based Films Modified with Transglutaminase, Glyoxal and Formaldehyde. Food Hydrocoll. 2004, 18, 717–726.
- Muyonga, J.; Cole, C.; Duodu, K. Extraction and Physico-Chemical Characterisation of Nile Perch (Lates Niloticus) Skin and Bone Gelatin. Food Hydrocoll. 2004, 18, 581–592.
- Charlton, A. J.; Baxter, N. J.; Khan, M. L.; Moir, A. J.; Haslam, E.; Davies, A. P.; Williamson, M. P. Polyphenol/Peptide Binding and Precipitation. J. Agric. Food Chem. 2002, 50, 1593–1601.
- Usha, R.; Ramasami, T. The Effects of Urea and N-Propanol on Collagen Denaturation: Using DSC, Circular Dicroism and Viscosity. Thermochim. Acta. 2004, 409, 201–206.
- Albu, M.; Ghica, M.; Leca, M.; Popa, L.; Borlescu, C.; Cremenescu, E.; Trandafir, V. Doxycycline Delivery from Collagen Matrices Crosslinked with Tannic Acid. Molecular Crystals Liquid Crystals. 2010, 523, 97/[669]–105/[677].
- Cao, N.; Fu, Y.; He, J. Mechanical Properties of Gelatin Films Cross-Linked, Respectively, by Ferulic Acid and Tannin Acid. Food Hydrocoll. 2007, 21, 575–584.
- Cui, L.; Jia, J.; Guo, Y.; Liu, Y.; Zhu, P. Preparation and Characterization of IPN Hydrogels Composed of Chitosan and Gelatin Cross-Linked by Genipin. Carbohydr. Polym. 2014, 99, 31–38.
- Albu, M.; Ghica, M.; Leca, M.; Popa, L.; Borlescu, C.; Cremenescu, E.; Trandafir, V. Doxycycline Delivery from Collagen Matrices Crosslinked with Tannic Acid. Molecular Crystals Liquid Crystals. 2010, 523(1), 97/[669]-105/[677].