ABSTRACT
In the present study, the gelatin from fresh croaker fish (Johnius sp) skin was obtained by rapid extraction and its properties were assessed. The gelling and melting temperature of croaker skin gelatin was 17.4 and 23.8°C as revealed by dynamic visco-elastic analysis using Controlled Stress Rheometer. Croaker skin gelatin (CSG) had the bloom value of 193.4 g. Scanning electron microscopic analysis of freeze dried gelatin showed the presence of voids surrounded by a loose network imitating a sponge or coral like structure. Texture profile parameters such as hardness, springiness, cohesiveness, gumminess, and chewiness of croaker fish skin gelatin gel were relatively lower than the porcine gelatin gel. The amino acid composition of gelatin revealed a higher proportion of glycine, alanine, and hydroxyl proline (30.25, 23.4, and 11.82 g/100 g protein, respectively) content. The electrophoretic analysis showed the presence of two peptide chains with the approximate molecular weight of 200 and 116 kDa corresponds to β and α peptide chain of collagen. The Fourier Transform Infrared (FT-IR) spectra of gelatin showed peaks correspond to the structures like α helix, β sheet, and random coil in the gelatin preparations.
Introduction
Gelatin is a water-soluble protein obtained from partial denaturation of collagen. It has a wide range of applications in photographic, cosmetic, biomedical, pharmaceutical, and neutraceutical industries.[Citation1–Citation3] In the food industry, gelatin has been widely used as an ingredient to improve the elasticity, consistency, and stability of foods due to its gelling property and surface active behavior.[Citation4] In recent years, the application of gelatin in functional food formulation and novel product development is gaining importance. The thermal and rheological properties of gelatin determine its uses in various food systems.[Citation5] Molecular weight distribution of peptides and amino acid composition are the key intrinsic factors that govern the properties of gelatin.[Citation6] The knowledge on the rheological and structural properties of gelatin is essential to widen its application in different food formulations.
The skin and bone of bovine and porcine are the major sources of raw material for gelatin production. The gelatin from bovine and porcine may not be acceptable to all due to religious and social considerations. Hence, the industry is constantly looking for alternate raw material for gelatin production which will be acceptable to all. Fish processing waste has been considered as an ideal raw material for gelatin production.[Citation7,Citation8]
The fish processing wastes comprise of skin, bones, swim bladder, and head. Fish skin accounts for more than 30% (w/w) of total fish processing waste and contains high amount of collagen.[Citation9] The quality of gelatin from fish skin depends on habitat temperature of fish and method of extraction.[Citation6] The main advantages of using fish processing waste for gelatin production are the availability of raw material and utilization of processing waste. The utilization of fish processing waste will minimize the environmental pollution. However, the quality of gelatin obtained from bovine and porcine sources are superior compared to gelatin obtained from fish processing waste.[Citation2,Citation10]
Croaker fish belonging to family Sciaenidae comprises of different species. Croakers are widely distributed both in temperate and tropical waters and average annual landings globally during the year 2011–2014 was 850,000 tons.[Citation11] Major quantity of the croakers landed are utilized for surimi (separated meat water washed, partially dehydrated, mixed with cryo-protectants, frozen, and frozen stored) production which generates substantial processing waste. Many researchers have focused on the production of gelatin from fish waste such as Bigeye snapper and brownstripe red snapper[Citation12]; sin croaker (Johniusdussumeri)[Citation13]; Bigeye snapper[Citation14]; Priacanthus tayenus and Priacanthus macracanthus[Citation7]; Splendid squid[Citation15]; freshwater carps[Citation16]; Clown featherback.[Citation17]
The method of extraction is critical in determining the final quality of gelatin. There are various methods available in literature for the extraction of gelatin from different sources of raw material. The basic principle underlying extraction of gelatin is to remove non-collagenous protein and subject to further extraction. The non-collagenous proteins are removed by treating with alkali solution or neutral salt solution or by enzymatic treatment.[Citation10,Citation18,Citation19] Acid treatment is the most appropriate for less cross-linked collagens commonly found in porcine and fish skins, whereas, alkaline treatment is suitable for the more complex collagens found in bovine hides.[Citation7] The acid/alkali treatment and further extraction of gelatin is a laborious process and can take more than 24 h to obtain gelatin. There were a few attempts to optimize the extraction of gelatin from fish processing waste using rapid method of extraction where the process time has been reduced by less than 2 h.[Citation10,Citation19] The pre-treatment conditions such as acid and alkali treatment will enable the manufacturer to use lower temperature for gelatin extraction.[Citation6] However, the use of acid/alkali in gelatin preparation is not environmental friendly. Hence, there is a need to use an alternate reagent for acid/alkali pre-treatments. The application of temperature for extraction is generally associated with shorter duration if neutral pH was chosen.[Citation3] The process optimization for gelatin production primarily aims at maximizing the yield with desirable physical properties. The gelatin industry will gain, if the process time for extraction is reduced considerably without compromising on desirable properties. With this rationale, the objectives of the present investigation were: a) to prepare the gelatin from fresh croaker skin using sodium chloride (NaCl) as pre-treatment agent; b) optimization of temperature, time, and ratio of fish skin to water; c) assessing physico-chemical and structural properties of extracted gelatin.
Materials and methods
Raw material
The fresh croaker fish (Johnius sp) in iced condition was procured from local landing centre, Mangalore, West coast of India, and brought to the laboratory. The average length and weight of fishes used for the study were 15 cm and 200 g, respectively. The fishes were washed in chilled water, beheaded, gutted, and filleted manually. The skin was separated from the fillets using fish meat bone separator (SG model, Tokyo Seikan Kaisha Limited, Tokyo, Japan). The separated skins were cleaned by scraping with a knife manually to remove the meat. The cleaned skins were washed in chilled potable water, air blast frozen, and stored at −20°C. Frozen stored skins were used for gelatin extraction within 15 days of storage. All the chemicals used were of analytical or reagent grade.
Extraction of gelatin
The gelatin extraction was carried out by the method as described by Kolodziejska et al.[Citation19] with slight modifications. The frozen skins were thawed and cut into small pieces of 1 cm3 and pre-treated with 0.75 M NaCl solution. The ratio of fish skin to NaCl solution was 1:6 (w/v) and duration of extraction was 10 min at 4°C. The fish skin were washed with chilled tap water and drained. The NaCl treatment and water washing was repeated twice. The temperature, time of extraction, and the ratio of skin to water were optimized for gelatin extraction. The details of method of extraction are presented in . The washed skins were gently stirred with appropriate volume of water and kept in water bath at a desired temperature and duration. After heat extraction, the extracts were cooled and subjected to centrifugation at 10,000 x g at 25°C for 15 min, using refrigerated centrifuge (SorvallLegand XTR centrifuge, Thermo Fisher Scientific, New Hampshere, USA) to separate the insoluble material. The supernatant was filtered using Whatman filter paper No.1 (Whatman Plc, Maidstone, Kent, UK). The filtrate was freeze dried to obtain gelatin. Freeze-drying was carried out in a freeze-dryer (Edward Freeze Dryer; Super Modulyo, Crawley, West Sussex, UK) attached to a vacuum pump (E2M-12; Edwards High Vacuum International, Manor Royal, Crawley, West Sussex RH10 2LW, UK) having the ultimate vacuum capacity of 0.003 mbar and maximum water vapour pumping rate of 0.18 kg/h. The thickness of the solution layer in the drying trays was less than 10 mm. The freeze-drying was carried out for 24 h at a temperature of 45°C with a vacuum of 0.3 mbar. The freeze-dried gelatin was stored in air-tight containers under desiccated conditions till further analysis was carried out.
Table 1. Effect of extraction condition on the yield of gelatin and some physico-chemical properties of gelatin obtained under optimum condition.
Gelatin yield
The yield of gelatin was calculated as a ratio of freeze-dried gelatin to wet weight of skin used for extraction and expressed in percentage.
pH and iso-electric point
The pH of 1% (w/v) gelatin solution was measured using pH meter (SystronicsModel: 361, 146, Ahmedabad, India). To determine the iso-electric point, the zeta potential analysis was carried out at different pH. In brief, 300 mg of gelatin was weighed and dispersed in 15 ml of deionized water and the pH was adjusted to 3.0, 5.0, 7.0, 9.0, and 11.0 using 1 M NaOH or 1 M HCl before making up to 30 ml. Aliquot of solutions were transferred to a disposable capillary zeta cell (Malvern Instruments, 179 Malvern, UK) to determine the Zeta potential in a Zetasizer Nano ZS (Malvern) based on laser 180 Doppler micro-electrophoresis. Measurements were conducted in triplicates at room temperature (25 ± 2°C).
Gelling and melting temperatures of gelatin
The gelling and melting temperature of gelatin solution was measured using Controlled Stress Rheometer (AR-1500ex, TA Instruments, Crawley, West Sussex, UK) under oscillatory mode. The concentration of gelatin used for measurement was 6.67% (w/v). The gelatin solution was heated to 60°C for 30 min and cooled to ambient temperature (25°C). The solution was then filtered using Whatman No. 4 filter paper (Whatman Plc, Maidstone, Kent, UK). The gelling and melting temperatures of gelatin were measured by assessing visco-elastic behavior in the temperature range of 0–30°C and 30–0°C, with heating/cooling rates of 2°C/min. A known volume of 1.5 ml was transferred on to Peltier plate and equilibrated at the desired temperature for 10 min before measurement. The measuring geometry used was 4 cm parallel plate and the gap set between measuring geometry and Peltier plate was 1000 µm. The frequency of oscillation was 1 Hz, and 1% strain was applied which was within linear visco-elastic region. The elastic modulus (G’), viscous modulus (G’’) and tan δ (G”/G’) values were recorded as a function of temperature. The gelling and melting temperatures of the gelatin were calculated from the plot of temperature vs G’ and G”. The cross-over point of G’ and G” was considered as the gelling or melting temperatures of the gelatin, respectively as described by Chandra and Shamasundar.[Citation20]
Bloom strength
The bloom strength of gelatin gel was determined using Texture Analyzer (Stable Micro Systems Ltd., Surrey, UK) according to the method described in official procedure of the Gelatin Manufacturers of Europe GME.[Citation21] A known quantity (7.50 g) of gelatin was weighed into the bloom jar (SCOTTGLAS Mainz. product No. 12402) and 105 ml deionized water was added. The jar was placed in water bath at 60°C for 20 min with occasional stirring, allowed to cool for 15 min at room temperature, and then placed in chilled condition (2–4°C) for 17 h. For determining the bloom strength, the bloom jar with gelatin gel was placed at the centre of the platform of the Texture Analyzer so that the plunger (1.27 cm flat-faced cylindrical probe) contacts the sample as nearly at its midpoint as possible. After attaining a 4 g trigger force, the probe proceeded to penetrate into the gel to a depth of 4 mm. At this depth, the maximum force reading was obtained and translated as bloom value (g) of the gel.
Scanning electron microscopy (SEM)
Microstructure of gelatin was obtained using a scanning electron microscopy (JEOL JSM-5800 LV, Tokyo, Japan). Samples having a thickness of 2–3 mm were fixed with 2.5% (v/v) glutaraldehyde in 0.2 M phosphate buffer (pH 7.2) for 12 h. The samples were then rinsed with distilled water for 1 h and dehydrated in ethanol with a serial concentration of 50, 70, 80, 90, and 100% (v/v). Dried samples were mounted on a bronze stub and sputter-coated with gold (Sputter coater SPI-Module, West Chester, PA, USA). The specimens were observed at an acceleration voltage of 20 kV.
Instrumental texture profile analysis (TPA)
Gelatin gels of 6.67% (w/v) were prepared by dissolving the dry powder in distilled water at 50–60°C for 30 min with occasional stirring using a magnetic stirrer. The homogenous solution was allowed to cool for 10 min and was kept in a refrigerator (4–6°C) for 24 h. The gelatin gels were removed and immediately used for texture profile analysis (TPA). TPA was performed using a TA-TX2 texture analyzer (Stable Micro Systems Ltd., Surrey, UK), attached with a 50 kg load cell. The test conditions were according to the method described by Chandra and Shamasundar.[Citation20] Textural parameters, such as hardness, adhesiveness, springiness, cohesiveness, gumminess, chewiness, and resilience were calculated with the help of software provided along with the instrument.
Amino acid composition
Amino acid content of gelatin samples were analyzed using the Pico-Tag method, as described by Bildlingmeyer et al.[Citation22] This method involves derivatization of amino acids using phenylisothiocyanate (PITC) and determination of the phenylthiocarbamyl (PTC) amino acids using a Pico-Tag reverse phase high-performance liquid chromatography (RP-HPLC) (Water Model 7122 WISP Waters, Watford, Herts, USA) (Badaii & Howell).[Citation23]
Sodium dodecyl sulphate—polyacrylamide gel electrophoresis (SDS-PAGE)
The electrophoretic mobility of gelatin under reduced conditions was obtained by the method described by Laemmli.[Citation24] Electrophoresis was carried out using polyacrylamide gel slabs of 10 × 8 cm (length × width) in a vertical slab electrophoresis apparatus (Model mighty small II, SE 250/SE 260, Hoefer Pharmacia Biotech Inc., Halliston, USA). Gelatin samples (1 mg/ml) were dissolved in 5% SDS solution and incubated at 85°C for 60 min, followed by centrifugation at 8000 × g for 10 min at room temperature. The supernatant was mixed with the sample buffer (0.5 M Tris-HCl, pH 6.8 containing 4% SDS, 20% glycerol and 0.03 mM of coomassive brilliant blue-G) at 1:1 (v/v) ratio and boiled for 3 min. Samples (10µl) were loaded onto a polyacrylamide gel (7.5% running gel and 4% stacking gel). The molecular weight of the proteins was approximated using standard wide range-molecular-weight protein markers (Sigma, St. Louis, MO, USA).
Fourier transform infrared spectroscopy (FT-IR)
The FT-IR spectra of gelatin sample were analyzed using FT-IR spectroscopy (Bruker Optik GmBH, Germany). A known quantity of the gelatin sample (30–40 mg) was mixed with a pinch of potassium bromide and macerated using pestle and mortar to get a fine powder. The powder was placed in the 13 mm diameter die provided by the manufacturer and made into a pellet using a hydraulic press by applying a pressure of 5000–10000 psi. The pressed pellet was carefully removed from the die and placed in the FT-IR sample holder. The pressed pellet was nearly transparent. The sample was analyzed for the presence of different functional groups in the region of 4000–500 cm−1 wave numbers with 4 cm−1 resolution. The analysis was carried out after due background correction.
Statistical analyses
The experiments were carried out in triplicates independently. The mean and standard deviation values were calculated using the statistics programme SPSS (SPSS.16.0 for windows, SPSS Inc., Chicago, IL).
Results and discussion
Gelatin yield
The extraction conditions such as temperature, time, and ratio of skin to water along with gelatin yield is given in . Based on the yield, visual colour, and odour (data on colour and odour not presented), it was found that a temperature of 85°C for the duration of 60 min with skin: water ratio of 1:6 to be optimum for extraction of gelatin. In any commercial operation, yield obtained will be critical and at the same time the properties of gelatin should meet the expected requirements. The yield of gelatin from clown featherback skin has been found to increase with increase in temperature.[Citation17] But at the same time, the properties such as bloom value, viscosity and colour were found to diminish.[Citation4,Citation17] The yield of gelatin from acid and alkali pre-treated skins of bigeye snapper, tiger-toothed croaker, and sin croaker was 4, 7.56, and 14.3%, respectively.[Citation13,Citation14,Citation25] Mohtar et al.[Citation10] reported 17.6% of yield from hoki skins pre-treated with 0.75 M NaCl. The differences in yield are mainly due to fish species, method of pre-treatment, temperature, and extraction duration.[Citation15] The conversion of collagen into gelatin depends on the processing parameters, pre-treatment conditions, and the method of preservation of raw material practiced. With increase in temperature and time of extraction, destabilization of bonds between α-chains of the native collagen molecules occurs.[Citation4] This results in higher recovery of gelatin. In the present study, a temperature of 85°C for 60 min yielded 7.15%. Further, characterization of gelatin obtained at optimum conditions were carried out.
pH and iso-electric point
The pH of 1% solution prepared using croaker skin gelatin was 6.57 (). As the gelatin was extracted without acid or alkali, it is reasonable to expect the pH value to be above 6. Based on the process pre-treatments and pH of obtained gelatin they are categorized in to Type A and B. Type A gelatin is derived from acid treated raw material and Type B is derived from lime/alkali treated raw material. These processes affect the iso-electric point, pH, and other properties. Type A has pI between 8 and 9 and usually carry positive charge at neutral pH while Type B has a pI between 4.8 and 5.4 and carry negative charge at neutral pH.[Citation15] The type B gelatin usually reported to have the pH value of 5–7.5, whereas, Type A gelatin have the pH values in the range of 3.8–5.5.[Citation26] The croaker skin gelatin obtained in the present study, based on the pH value, can be categorized as Type B. This was confirmed by surface charge analysis using zeta potential measurement.
The iso-electric point was found to be closer to 5.85 () and carried negative charge at neutral pH 7. The zeta potential value (mV) was increasing on either side of 5.85 toward positive and negative charges, respectively. The results clearly indicate that the gelatin prepared from croaker skin in the present investigation belongs to Type B. The iso-electric point of gelatin samples prepared from splendid squid skin reported to be shifted from 5.94 to 5.49 and 4.86 when the extraction temperature increased from 60 to 70 and 80°C, respectively.[Citation15] This change in iso-electric point has been attributed to the deamidation of glutamic and aspartic acid contents at high temperatures. The charge distribution in gelatin sample is mainly determined by the positively (histidine, lysine, and arginine) and negatively charged amino acids (glutamic acid and aspartic acid). In the present study, the amino acid analysis showed a higher proportion of basic amino acids than acidic amino acids. Basic amino acids assume positive charge and acidic amino acids assume negative charge at neutral pH. In spite of this, at neutral pH, the overall surface charge of croaker skin gelatin was found to be negative. This indicates that the side chain of basic amino acids in the gelatin sample is in the bound form/interacted with other molecules. Hence, the side chains are not available freely to assume the charge.
Gelling and melting temperatures of gelatin
The dynamic visco-elastic behaviour (DVB) of gelatin samples was monitored in the temperature range 0–30°C and 30–0°C so as to obtain the gelling and melting point. The results of DVB are presented in . In the DVB measurement a stress in the form of sinusoidal wave was applied and resultant parameters such as storage modulus (G’) and loss modulus (G’’) and phase angle (tan δ) were obtained as a function of temperature. The gelling and melting temperatures were derived from the plot of temperature vs G’, G”, and tan δ. The temperature at which cross-over of G’ and G’’ occurs is close to sol-gel transition temperature. From ), gelling temperature of croaker skin gelatin was found to be 17.4°C. At the cross-over point, there is a sharp decrease in tan δ values suggesting the formation of gel.
Figure 2. Dynamic visco-elastic behavior (DVB) of craoker skin gelatin. A) Cooling curve in the temperature range of 30–0°C. B) Melting curve in the temperature range of 0–30°C.
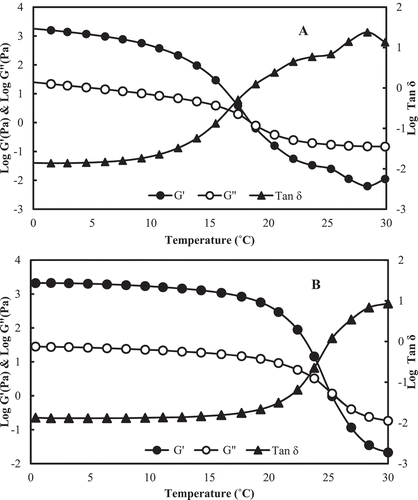
The melting temperature of gelatin was found to be 23.8°C ()) which was obtained by cross-over of G’ and G’’ curves. The tan δ values at the melting temperature increase sharply indicating the loss of gel structure and formation of viscous fluid. The gelling and melting temperatures of gelatin obtained from different sources vary with the nature of raw material, amino acid composition, and molecular weight distribution of peptides in the gelatin extract. The gelling and melting temperatures of gelatin obtained from the skin of different species were varied in nature. The gelling temperature of gelatin from bigeye snapper was found to be 10°C while that of gelatin from Indian major carps was in the range 6–15.7°C.[Citation1,Citation27]A higher gelling and melting temperature is normally obtained from gelatin of bovine and porcine sources.[Citation28] It has been indicated that the content of imino acids (hydoxyproline + proline) is critical in deciding the gelling and melting temperature.[Citation8] The imino acid content of bovine and porcine account for nearly 23% to the total amino acid content.[Citation29,Citation30] In the present study, the imino acid content of gelatin was 17.4% and thus leading to lower gelling and melting temperature.
Bloom strength
The bloom strength of croaker skin gelatin was 193.4 g (). The bloom strength of commercial gelatin varies from 100 to 300 g. The gelatin with a bloom value of 250–260 g bloom is the most desirable.[Citation31] The bloom strength of fish gelatin from the Nile perch was related to age of the fish.[Citation32] Similarly, the bloom strength of gelatin from the skin of seabass was found to be related with size of skin used for extraction.[Citation4] Gelatin from big size skin yields higher bloom value. The bloom values of gelatin extracted from the skins of splendid squid and clown feather back after acid and alkali pre-treatment at 80°C were 85 g and 140 g, respectively.[Citation15,Citation17] The difference in bloom strength of gelatin from different species is due to amounts of α and β components and amino acid composition. The imino acid, especially hydroxy proline, is involved in gel formation through hydrogen bonding by hydroxyl group. In the present study, the hydroxyproline content was found to be 11.8% () and higher proportion of β component (), which has led to reasonably high bloom strength. The bloom value (193.4 g) of croaker skin gelatin obtained in the present study indicates its suitability as a food ingredient in gelatin dessert, frozen foods, dairy products, meat products, wine, beer, and juices.[Citation15]
Table 2. Texture profile analysis of croaker skin waste gelatin gels.
Table 3. Amino acid composition of croaker skin gelatin.
Scanning electron microscopy (SEM)
Microstructure and surface area are the most important for its biomedical applications .[Citation2] The microstructure of croaker fish skin gelatin was sponge or coral-like in structure with looser network, larger voids in matrix and denser strands (). Gelatin had denser strand with small pores due to the different characteristics of gelation in terms of aggregation and alignment of protein molecules.[Citation12] The larger voids in the matrix may be due to extraction of gelatin at harsh conditions. It was found that the gelatin extracted at mild conditions had smaller voids compared to gelatin extracted under harsher conditions. The smaller voids indicate the uniform distribution of water in the fine and ordered matrix.[Citation4,Citation23]
Texture profile analysis (TPA)
The texture profile analysis (TPA) of gelatin gels are given in . The TPA includes application of controlled force to the product and recording its response with time. The TPA can give an indication of sensory properties. The textural properties of gels obtained by TPA correlate well with sensory evaluation results.[Citation33] The parameters that are obtained by TPA include hardness, adhesiveness, springiness, cohesiveness, gumminess, chewiness, and resilience. Each of these parameters gives important information on textural properties of gelatin. The hardness value of croaker skin gelatin, 15.51 N, is related to strength of the gel and is the peak force at first compression cycle. The hardness values obtained for gelatin from different fish skins varied from 20.16 to 0.41 N.[Citation16,Citation18,Citation34] This wide variation may be due to difference in composition of fish skin, extraction procedure, and method of analysis. Higher hardness values indicate the maximum force required to compress the food between molar teeth.[Citation35] The high hardness values obtained in present study indicates the method of gelatin extraction used is optimum.
The springiness value for croaker skin gelatin was 0.96 mm. Springiness is related to elasticity of sample. The samples with high springiness values require more energy for mastication in the mouth. The adhesiveness (g.sec) value obtained from the fish skin gelatin gels −20.3 g.sec was lower than the adhesiveness values reported for gelatin obtained from fresh water carps.[Citation16]
The cohesiveness (dimensionless) value of croaker fish skin gelain gel was 0.93. Muyonga et al.[Citation32] reported the cohesiveness value of 0.93 for Nile perch skin gelatin. The gumminess value of croaker skin gelatin gel was 14.43 N, which is higher than the gumminess values of fresh water carp skin gelatin gels.[Citation16] High gumminess value is due to high hardness value. The chewiness (N) value of croaker fish skin gelatin gel was found to be 19.6 N which is lower than value of porcine gelatin. The fish gelatin gel had resilience value of 0.90, which is comparable to the resilience value of mrigala fish skin gelatin reported by Chandra and Shamasundar.[Citation16]
Amino acid composition
The amino acid composition of gelatin extracted from croaker fish skin is presented in . The results showed a high proportion of glycine and alanine (30.25 and 23.49 g/100 g of protein) (). The imino acid content (hydroxyproline + proline) of croaker skin gelatin was found to be 17.94 g/100 g of protein. The amino acid residues such as cysteine and tryptophan were absent in gelatin samples. The content of hydrophobic amino acids in croaker fish skin gelatin was found to be 38.15 g/100 g of protein.
Alanine plays an important role in providing the visco-elastic properties of gelatin while imino acids are responsible for imparting the strength to the gel network and stabilization of triple helical structure in renatured gelatin.[Citation8,Citation9,Citation17] It has been reported that there is a high degree of correlation between imino acid content of gelatin and bloom value. The imino acid content of gelatin from the skins of bigeye snapper, sin croaker, shortfin scad, and seabass was found to be 14.4, 11, 10, and 23.3 g/100 g protein, respectively. Higher imino acid content in gelatin samples results in gelatin gels with higher gel strength/bloom strength.[Citation4,Citation13,Citation14] The changes in imino acid content might be due to differences in cross-links in the (raw material) skin used for gelatin preparation.[Citation4] The amount of imino acids, especially hydroxyl proline, depends on the environmental temperature in which the fish lives and it affects the thermal stability of the collagens.[Citation36]
SDS-PAGE pattern of croaker skin gelatin
The SDS-PAGE pattern of croaker skin gelatin is given in . The pattern showed three major bands in the molecular weight range of 200–120 kDa. The protein pattern of croaker skin gelatin was almost similar to that of porcine skin gelatin. The high molecular weight band (200 kDa) is β component while the bands at 120 kDa are α1 and α2 components. The croaker skin gelatin did not show any low molecular weight bands indicating the complete removal of meat proteins (non-collagenous) from the skin. The appearance of α and β chains are mainly due to degradation of native collagen during extraction. The approximate molecular weight of gelatin from croaker skin calculated from SDS-PAGE pattern was 320 kDa. The molecular weight of gelatin from the skin of bigeye snapper was found to be 290 kDa.[Citation14] In the present study, slightly high molecular weight was recorded probably due to absence of acid or alkali pre-treatment. The physical properties of gelatin depend on the relative content of α-chains, β components and higher molecular weight aggregates, as well as on the presence of lower molecular weight protein fragments.[Citation6] Kittiphattanabawon et al.[Citation17] reported that extraction of gelatin at high temperature induces degradation and forms low molecular weight peptides. At an extraction temperature of 85°C for duration of 6 h and 12 h, α and β chains were more degraded particularly with increasing extraction time. In the present study, extraction temperature of 85°C for 60 min has not degraded α-chains as indicated by absence of low molecular peptides.
Fourier transform infrared spectroscopy (FT-IR)
FT-IR spectra of croaker fish skin gelatin samples in the wave number ranging from 4000 to 500 cm−1 are presented in . The rotational and vibrational frequencies of the molecules in the infrared region of electromagnetic spectrum can be employed to study the molecular rotations and vibrations. These rotations and vibrations are sensitive to chemical changes and can be monitored with infrared spectroscopy.
The FT-IR spectra results indicated spectral bands at wave number 3423 and 2925 cm−1 and can be assigned to amide A and amide B components. The amide A arises mainly due to OH and NH stretching vibrations. The amide B arises mainly due to NH stretching vibrations. The amide A band in spectra is broader which likely to be temperature induced. According to Kemp,[Citation37] the amide A band tends to merge with CH2 stretch peak and carboxylic groups, exist in stable dimeric (intermolecular associations). This association is related to high temperature gelatin extract, which mainly comprises of low molecular weight peptides. However, in the present study, it is evident from SDS-PAGE pattern that gelatin samples have not been degraded into low molecular components and likely emergence of broader amide A band is perhaps due to stretching of CH2 molecule.
The FT-IR spectra can be made use of to get the information on secondary structure is being employed to study the protein in terms of functional groups and the secondary structures like α-helix and β-sheet. The results of present study clearly indicate that the band at 1642 cm−1 could be due to random coil structure. In FT-IR spectra, the band around 1654 cm−1 is assigned to α helix or disorder structure.[Citation38] The presence of β sheet in secondary structure is indicated at wave number 1638 cm−1.[Citation39] In the present study, it is likely that the presence of β secondary structure cannot be ruled out as there is a peak at wave number of 1642 cm−1 as amide I band. The amide I band arises mainly due to C = O stretching vibrations (80%) and to lesser extent because of C-N stretching vibration (20%) of peptide linkages. The amide II spectra are due to NH bending (60%) and CN stretching vibration (40%).[Citation40]
The FT-IR spectra of croaker skin gelatin has shown peak at different wave numbers and will be difficult to assign appropriate functional groups as the vibrational band appearing at different wave numbers are very complex and depends on nature of side chain involved in hydrogen bonding, which therefore of little use in protein conformational studies. The peak at wave number of 1242 cm−1 can be assigned to amide III. The FT-IR spectra of skin gelatin of sea bass and Nile perch showed amide III band in wave number of 1236 and 1235 cm−1.[Citation4,Citation41] The emergence of amide III band is indicative of presence of greater disordered structure in gelatin and could be associated with loss of triple helix.[Citation41] In the present study, extraction temperature used was 85°C and this would be responsible for the formation of amide III band. The same observation has been made in gelatin extracted from Nile perch at high temperature of 70°C.[Citation32] The results of FT-IR spectra clearly indicate that the optimum conditions (85°C for 60 min) for extraction of gelatin has not altered the secondary structure and is comparable to FT-IR spectra of gelatin obtained from different sources.
Conclusion
The optimum conditions for the extraction of gelatin from croaker fish skin by rapid method were standardized. The optimum temperature and time of extraction for gelatin was found to be 85°C and 60 min, respectively. The yield, bloom value, gelling and melting temperature of gelatin obtained by rapid method of extraction did not show much variation compared to that of conventional method of extraction. The secondary structure of extracted gelatin as revealed by FT-IR spectra showed loss of triple helix structure and more disordered in nature. The textural properties of croaker skin gelatin are slightly lower than commercial porcine gelatin and can be related to imino acid content. The extraction procedure detailed in the present study is less time consuming and does not involve use of acid or alkali. Further, the properties of gelatin are comparable to the gelatin obtained from conventional method of extraction.
Acknowledgment
University Grants Commission fellowship, Government of India, to the first author and financial support provided by European Union, Brussels under FP-7, SECUREFISH (Grant No.289282) for conducting the research work is gratefully acknowledged.
References
- Regenstein, J. M.; Zhou, P. Collagen and Gelatin from Marine By-Products. In Maximising the Value of Marine By-Products, 1st ed.; Shahidi, F. ed.; Woodhead Publishing Limited; Cambridge, England; 2007; pp. 279–303.
- Veeruraj, A.; Arumugam, M.; Balasubramanian, T. Isolation and Characterization of Thermostable Collagen from the Marine Eel-Fish (Evenchelysmacrura). Process Biochem. 2013, 48, 1592–1602. DOI: 10.1016/j.procbio.2013.07.011.
- Benjakul, S.; Kittiphattanabawon, P.; Regenstein, J. M. Fish Gelatin. Food Biochemistry and Food Processing, 2nd ed.; John Wiley & Sons, Inc.: England 2012, pp. 388–405.
- Sinthusamran, S.; Benjakul, S.; Kishimura, H. Molecular Characteristics and Properties of Gelatin from Skin of Seabass with Different Sizes. Int. J. Biol. Macromol. 2015, 73, 146–153. DOI: 10.1016/j.ijbiomac.2014.11.024.
- Giménez, B.; Turnay, J.; Lizarbe, M. A.; Montero, P.; Gómez-Guillén, M. C. Use of Lactic Acid for Extraction of Fish Skin Gelatin. Food Hydrocoll. 2005, 19, 941–950. DOI: 10.1016/j.foodhyd.2004.09.011.
- Johnston-Banks, F. A.;. Gelatin. In Food Gels; Harris, P., Ed.; Elsevier Applied Sciences: New York, USA; 1990; pp. 233–289.
- Benjakul, S.; Oungbho, K.; Visessanguan, W.; Thiansilakul, Y.; Roytrakul, S. Characteristics of Gelatin from the Skins of Bigeye Snapper. Priacanthustayenus and Priacanthusmacracanthus. Food Chem. 2009, 116, 445–451. DOI: 10.1016/j.foodchem.2009.02.063.
- Haug, I. J.; Draget, K. I.; Phillips, G. O.; Williams, P. A. Gelatin. Handbook of Hydrocolloids; Woodhead Publishing Limited: Cambridge, England; 2009, pp. 142–163.
- Gomez-Guillen, M. C.; Turnay, J.; Fernandez-Diaz, M. D.; Ulmo, N.; Lizarbe, M. A.; Montero, P. Structural and Physical Properties of Gelatin Extracted from Different Marine Species: A Comparative Study. Food Hydrocoll. 2002, 16, 25–34. DOI: 10.1016/S0268-005X(01)00035-2.
- Mohtar, N. F.; Perera, C.; Quek, S. Y. Optimisation of Gelatine Extraction from Hoki (Macruronusnovaezelandiae) Skins and Measurement of Gel Strength and SDS–PAGE. Food Chem. 2010, 122, 307–313. DOI: 10.1016/j.foodchem.2010.02.027.
- FAO. The State of World Fisheries and Aquaculture; FAO Fisheries and Aquaculture Department: Rome, 2016.
- Jongjareonrak, A.; Benjakul, S.; Visessanguan, W.; Tanaka, M. Skin Gelatin from Bigeye Snapper and Brownstripe Red Snapper: Chemical Compositions and Effect of Microbial Transglutaminase on Gel Properties. Food Hydrocoll. 2006, 20, 1216–1222. DOI: 10.1016/j.foodhyd.2006.01.006.
- Cheow, C. S.; Norizah, M. S.; Kyaw, Z. Y.; Howell, N. K. Preparation and Characterisation of Gelatins from the Skins of Sin Croaker (Johniusdussumieri) Andshortfinscad (Decapterusmacrosoma). Food Chem. 2007, 101, 386–391. DOI: 10.1016/j.foodchem.2006.01.046.
- Binsi, P. K.; Shamasundar, B. A.; Dileep, A. O.; Badii, F.; Howell, N. K. Rheological and Functional Properties of Gelatin from the Skin of Bigeye Snapper (Priacanthushamrur) Fish: Influence of Gelatin on the Gel-Forming Ability of Fish Mince. Food Hydrocoll. 2009, 23, 132–145. DOI: 10.1016/j.foodhyd.2007.12.004.
- Nagarajan, M.; Benjakul, S.; Prodpran, T.; Songtipya, P.; Kishimura, H. Characteristics and Functional Properties of Gelatin from Splendid Squid (Loligoformosana) Skin as Affected by Extraction Temperatures. Food Hydrocoll. 2012, 29, 389–397. DOI: 10.1016/j.foodhyd.2012.04.001.
- Chandra, M. V.; Shamsundar, B. A. Rheological and Physico‐Chemical Properties of Gelatin Extracted from the Skin of a Few Species of Freshwater Carp. Int. J. Food Sci. Technol. 2014, 49, 1758–1764. DOI: 10.1111/ijfs.12511.
- Kittiphattanabawon, P.; Benjakul, S.; Sinthusamran, S.; Kishimura, H. Gelatin from Clown Featherback Skin: Extraction Conditions. LWT-Food Sci. Technol. 2016, 66, 186–192. DOI: 10.1016/j.lwt.2015.10.029.
- Jridi, M.; Nasri, R.; Salem, R. B. S. B.; Lassoued, I.; Barkia, A.; Nasri, M.; Souissi, N. Chemical and Biophysical Properties of Gelatins Extracted from the Skin of Octopus (Octopus Vulgaris). LWT-Food Sci. Technol. 2015, 60, 881–889. DOI: 10.1016/j.lwt.2014.10.057.
- Ko1odziejska, I.; Skierka, E.; Sadowska, M.; Ko1odziejski, W.; Niecikowska, C. Effect of Extracting Time and Temperature on Yield of Gelatin from Different Fish Offal. Food Chem. 2008, 107, 700–706. DOI: 10.1016/j.foodchem.2007.08.071.
- Chandra, M. V.; Shamasundar, B. A. Texture Profile Analysis and Functional Properties of Gelatin from the Skin of Three Species of Fresh Water Fish. Int. J. Food Prop. 2015, 18(3), 572–584. DOI: 10.1080/10942912.2013.845787.
- Gelatin Manufacturers of Europe (GME). Standardised Methods for the Testing of Edible Gelatin; Gelatin Monograph, Bruxelles; 2000. version July.
- Bidlingmeyer, B. A.; Cohen, S. A.; Tarvin, T. L. Rapid Analysis of Amino Acids Using Pre-Column Derivatization. J. Chromatogr. B Biomed. Sci. Appl. 1984, 336, 93–104. DOI: 10.1016/S0378-4347(00)85133-6.
- Badii, F.; Howell, N. K. Fish Gelatin: Structure, Gelling Properties and Interaction with Egg Albumen Proteins. Food Hydrocoll. 2006, 20, 630–640. DOI: 10.1016/j.foodhyd.2005.06.006.
- Laemmli, U. K.;. Cleavage of Structural Proteins during the Assembly of the Head of Bacteriophage T4. Nature. 1970, 227, 680–685. DOI: 10.1038/227680a0.
- Koli, J. M.; Basu, S.; Nayak, B. B.; Patange, S. B.; Pagarkar, A. U.; Gudipati, V. Functional Characteristics of Gelatin Extracted from Skin and Bone of Tiger-Toothed Croaker (Otolithesruber) and Pink Perch (Nemipterusjaponicus). Food Bioprod. Process. 2012, 90, 555–562. DOI: 10.1016/j.fbp.2011.08.001.
- Baziwane, D.; He, Q. Gelatin: The Paramount Food Additive. Food Rev. Int. 2003, 19, 423–435. DOI: 10.1081/FRI-120025483.
- Madhamuthanalli, C. V.; Bangalore, S. A. Rheological and Physico‐Chemical Properties of Gelatin Extracted from the Skin of a Few Species of Freshwater Carp. Int. J. Food Sci. Technol. 2014, 49, 1758–1764. DOI: 10.1111/ijfs.12511.
- Ahmed, J.;. Rheological Properties of Gelatin and Advances in Measurement. In Advances in Food Rheology; Ahmed, J., Ed.; Elsevier: New York, USA; 2017; pp. 377–404.
- Arvanitoyanni, I. S.;. Formation and Properties of Gelatin Films and Coatings. In Protein-Based Films and Coatings; Gennadios, A.; Ed.; CRC Press: New York, USA; 2002.
- Eastoe, J. E.; Leach, A. A. Chemical Constitution of Gelatin [From Mammals, Chicken Tendon, Calf Skin, Pig Skin]; Ward, A. G.; Courts, A.; Eds, The science and technology of gelatin, Academic Press: London, England; 1977, pp. 73–107.
- Holzer, D.; Gelatin Production. US patent, 5, 484, 888, 1996.
- Muyonga, J. H.; Cole, C. G. B.; Duodu, K. G. Extraction and Physico-Chemical Characterisation of Nile Perch (Latesniloticus) Skin and Bone Gelatin. Food Hydrocoll. 2004, 18, 581–592. DOI: 10.1016/j.foodhyd.2003.08.009.
- Lau, M. H.; Tang, J.; Paulson, A. T. Texture Profile and Turbidity of Gellan/Gelatin Mixed Gels. Food Res. Int. 2000, 33, 665–671. DOI: 10.1016/S0963-9969(00)00111-3.
- Ktari, N.; Jridi, M.; Nasri, R.; Lassoued, I.; Ayed, H. B.; Barkia, A.; Nasri, M. Characteristics and Functional Properties of Gelatin from Zebra Blenny (Salariabasilisca) Skin. LWT-Food Sci. Technol. 2014, 58, 602–608. DOI: 10.1016/j.lwt.2014.03.036.
- Szczesniak, A. S.;. Texture Is a Sensory Property. Food Qual. Prefer. 2002, 13, 215–225. DOI: 10.1016/S0950-3293(01)00039-8.
- Balian, G.; Bowes, J. H. The Structure and Properties of Collegen. In From Science and Technology of Gelatin; Ward, A. G., Courts, A. Ed.; Academic press Inc: New York, USA, 1977; pp. 1–27.
- Kemp, W.;. Organic Spectroscopy, 2nd ed.; Macmillian Education Ltd: Hampshire, England; 1987.
- Barth, A.;. Infrared Spectroscopy of Proteins. Biochim. Biophys. Acta. 2007, 1767, 1073–1101. DOI: 10.1016/j.bbabio.2007.06.004.
- Elavarasan, K.; Shamasundar, B. A.; Badii, F.; Howell, N. Angiotensin I-Converting Enzyme (ACE) Inhibitory Activity and Structural Properties of Oven-And Freeze-Dried Protein Hydrolysate from Fresh Water Fish (Cirrhinusmrigala). Food Chem. 2016, 206, 210–216. DOI: 10.1016/j.foodchem.2016.03.047.
- Kong, J.; Yu, S. Fourier Transform Infrared Spectroscopic Analysis of Protein Secondary Structures. Acta Biochim. Biophys. Sin. 2007, 39, 549–559.
- Muyonga, J. H.; Cole, C. G. B.; Duodu, K. G. Fourier Transform Infrared (FTIR) Spectroscopic Study of Acid Soluble Collagen and Gelatin from Skins and Bones of Young and Adult Nile Perch (Latesniloticus). Food Chem. 2004, 86, 325–332. DOI: 10.1016/j.foodchem.2003.09.038.