ABSTRACT
Impact of roasting and boiling on the phenolic constituents, anti-radicals, xanthine oxidase (XO), and angiotensin 1-converting enzyme (ACE) inhibitory potentials of Brachystegia eurycoma seeds (BES) was evaluated. Raw BES contained caffeic acid, ellagic acid, rutin, and quercetin as the predominant phenolics, and strongly scavenged DPPH* and ABTS*+, and inhibited XO and ACE. Both roasting and boiling resulted in decrease in the levels of phenolics and bioactivities of the seeds, but roasted seeds retained higher levels of the phenolics and the bioactivities than the boiled seeds. Roasting could be recommended as a valuable processing method for the retention of the phenolics and bioactivities of BES.
Introduction
Brachystegia eurycoma Harms, belonging to the Caesalpinoideae family, is an underutilized legume that grows mostly in the tropical rain forest of West Africa. It is regarded as an economic tree crop due to its wide range of uses, including in food and ethnomedicine[Citation1] and as a source of timber.[Citation2] In West Africa, especially in southeastern part of Nigeria, many rural dwellers use the edible seeds flour as a natural thickening agent in traditional soups.[Citation1,Citation3] The seeds flour improves the consistency and flavour of traditional soups. Previous studies on the nutrients composition of the seed indicated that it is a rich source of nutrients supplement.[Citation3,Citation4]
In the rural areas of West Africa where B. eurycoma seeds and other underutilized legume seeds are used to thicken traditional soups, it has been observed that there are fewer incidences of nutritionally related diseases such as obesity, cardiovascular diseases, diabetes, and protein malnutrition.[Citation5] Ndukwu[Citation6] further noted that consumption of the B. eurycoma seeds helps in softening bulky stools and could protect against colorectal cancer. A recent in vitro study reported that the extract of B. eurycoma seeds flour inhibited some enzymes (α-amylase, α-glucosidase, and aldose reductase) that are associated with type 2 diabetes.[Citation7] In a related study, the seeds flour was reported to be rich in flavonoids and phenolic acids and to display antidiabetic activity in type 2 diabetic rats.[Citation1]
The various health benefits of legume seeds and other plant foods have been attributed to their bioactive compounds such as flavonoids and phenolic acids.[Citation1,Citation8] Hence, phenolic-rich extracts of some plant foods have been reported to be useful for the management of gout and hypertension through the inhibition of xanthine oxidase (XO) and angiotensin 1-converting enzyme (ACE), respectively, and scavenging of free radicals.[Citation9,Citation10] XO (EC 1.1.3.22) catalyzes the last two reactions in the catabolism of purine nucleotides, in which hypoxanthine is first converted to xanthine, and finally to uric acid; with a concomitant reduction of molecular oxygen to superoxide, a reactive oxygen species (ROS).[Citation11] Excessive activity of XO leads to over-production of uric acid, and consequently, hyperuricaemia. Subsequently, the deposition of monosodium urate monohydrate crystals in tissues of the body, particularly the joints, eventually results in gout.[Citation12] When in excess, the ROS and other uric acid-derived radicals generated from XO-catalyzed reactions can activate the renin/angiotensin pathway,[Citation13] thereby leading to the development of cardiovascular diseases such as hypertension.[Citation14]
In the renin/angiotensin pathway, ACE catalyzes the cleavage of angiotensin I to angiotensin II. The Angiotensin II produced is a vasoconstrictor that stimulates the secretion of aldosterone, and inactivates bradykinin, a vasodilator and hypotensive peptide, thereby raising the blood pressure.[Citation15] Hence, overactivity of ACE can cause the development of hypertension.[Citation16] Studies in different human populations have revealed a positive correlation between hypertension and the incidence and prevalence of gout.[Citation17]
One of the important clinical approaches for the treatment of gout and hypertension is the inhibition of XO and ACE, respectively.[Citation9,Citation10] Chemically synthesized inhibitors of XO (e.g. allopurinol) and ACE (e.g. captopril) available for the treatment of each of these two diseases,[Citation18,Citation19] however, have certain adverse effects. For example, XO inhibitors are associated with the risk of developing hypersensitivity syndrome, which is characterized by side effects such as hepatic dysfunction, renal impairment, rashes, and fever.[Citation20] Similarly, ACE inhibitors have been associated with such adverse effects as cough, skin rashes, proteinuria, and hypotension.[Citation21] To address the adverse effects of the synthetic inhibitors and the high cost of buying them, research efforts have been intensified recently in finding natural inhibitors of these enzymes.
Traditionally, B. eurycoma seeds are processed in various ways, including boiling in water and roasting. After any of these thermal treatments, they are shelled and ground into flour, which is then used to thicken and flavour traditional soups.[Citation22] However, processing methods are known to affect the levels of phenolic compounds in various dietary sources and their health-promoting bioactivities.[Citation23,Citation24] Hence, this study was designed to evaluate the effect of two processing methods (roasting and boiling) on the phenolic constituents, anti-radicals and enzymes (XO and ACE) inhibitory potentials of B. eurycoma seeds.
Materials and methods
Chemicals and reagents
All chemicals were of analytical grade. Methanol, acetonitrile, acetic acid, gallic acid, caffeic acid, and chlorogenic acid were purchased from Merck (Darmstadt, Germany). Quercetin, rutin, luteolin; DPPH, l-ascorbic acid, ABTS, Trolox, xanthine, allopurinol, rabbit lung ACE, captopril and hippuryl-histidyl-leucine were bought from Sigma Chemical Co. (St. Louis, MO, USA).
Samples collection and preparation
About 1.5 kg of dry B. eurycoma seeds sample was purchased at Bodija market in Ibadan, Oyo State, Nigeria. The seeds were authenticated at the Herbarium in Department of Botany, University of Ibadan, Nigeria. Subsequently, the seeds were sorted and later divided into three portions of about 500 g each. The first portion served as raw (untreated) B. eurycoma seeds. The second portion was roasted in a thermostatically regulated hot-air oven at 150 ± 2°C for 20 min; while the third portion was boiled in tap water at 1:10 (seeds:water) ratio in a thermostatically regulated water bath (100 ± 0.5°C) for 20 min. After the boiling time, the seeds were placed on filter paper at room temperature for 15 min to drain out the water retained on the seeds. Thereafter, the boiled seeds were oven-dried at 40°C for 48 h to attain a constant moisture content of about 10%. Each sample portion was manually shelled, and later milled into flour of 0.5 mm particle size. The flour samples were packed in an airtight container and stored at 4°C for further analysis.
Preparation of polyphenolics-rich extracts
Polyphenolics-rich extracts of the raw, roasted, and boiled B. eurycoma seeds flours were prepared according to the method described by Kuo et al.[Citation25] In brief, 100 g portion of each sample was extracted with 300 mL absolute methanol at 50°C for 3 h in three successive times. Each sample was filtered with Whatman (No. 2) filter paper, and the extract was collected, following each successive extraction. Thereafter, the extract was partitioned with 200 mL of hexane in a separatory funnel, and the aqueous phase was further extracted with 180 mL of ethyl acetate for three successive times. The resulting extract of each sample was dried in a rotary evaporator at 45°C under reduced pressure. The residue obtained after drying of each sample was used for the various analyses.
Quantification of phenolic acids and flavonoids by HPLC-DAD
Phenolic acids and flavonoids constituents of the extracts were quantified using a reverse phase high-performance liquid chromatography (HPLC-DAD) system (Shimadzu, Kyoto, Japan), equipped with a Shimadzu Prominence Auto-sampler (SIL-20A) and Shimadzu LC-20AT reciprocating pumps connected to a DGU 20A5 degasser with a CBM 20A integrator, SPD-M20A diode array detector, and LC solution 1.22 SP1 software. Each extract was injected by means of the auto-sampler at a concentration of 10 mg/mL. Chromatographic separations were carried out using Phenomenex C18 column (4.6 mm × 250 mm × 5 μm particle size). The mobile phase was HPLC grade water with 1% acetic acid (v/v) (solvent A) and HPLC grade acetonitrile (solvent B) at a flow rate of 0.6 mL/min and injection volume 40 μL. The gradient programme composition was 5% solvent B reaching 15% at 10 min; 30% solvent B at 25 min, 65% solvent B at 40 min, and 98% solvent B at 45 min, followed by 50 min at isocratic elution until 55 min. At 60 min the gradient reached the initial conditions again, following the method described by Adefegha et al.[Citation26] with slight modifications. The extracts and mobile phase were filtered through 0.45 μm membrane filter (Millipore) and then degassed by ultrasonic bath before use. Stock solutions of standards references were prepared in the acetonitrile: water (1:1, v/v) at a concentration range of 0.025–0.400 mg/mL. Quantifications were carried out by integration of the peaks using the external standard method at 254 nm for gallic acid and ellagic acid; 327 nm for caffeic acid; and 366 nm for quercetin, rutin, and luteolin. The chromatography peaks were confirmed by comparing their retention time with those of reference standards and by DAD spectra (200–600 nm). Calibration curve for gallic acid: Y = 16745x + 1329.8 (r = 0.9998); caffeic acid: Y = 12546x + 1165.3 (r = 0.9999); ellagic acid: Y = 11784x + 1094.5 (r = 0.9995); rutin: Y = 15083x + 1193.4 (r = 0.9997); luteolin: Y = 13749x + 1285.1 (r = 0.9999); and quercetin: Y = 11645x + 1425.9 (r = 0.9998). All chromatographic operations were carried out at ambient temperature and in triplicate.
Limit of detection (LOD) and limit of quantification (LOQ) were calculated based on the standard deviation (SD) of the responses and the slope using three independent analytical curves, as defined by Fernandes et al.[Citation27] LOD and LOQ were calculated as 3.3 and 10 σ/S, respectively, where σ is the SD of the response and S is the slope of the calibration curve.
DPPH* scavenging assay
The ability of each extract to scavenge DPPH* was determined according to the method reported by Cervato et al.[Citation28] A portion of 1.0 mL of various concentrations (15, 30, 45, and 60 μg/mL) of the extracts was mixed with 3.0 mL of DPPH* solution (60 µM) in test tube. The test mixture was kept in the dark for 30 min, and thereafter the absorbance was read at 517 nm in a UV/visible spectrophotometer. A reference test (with methanol in the place of the extract) and a positive control test (with ascorbic acid solution in the place of the extract) were included in the assay. The ability of each extract to scavenge DPPH* was subsequently calculated as % scavenging ability and expressed as half-maximal scavenging concentration (SC50). The SC50 was obtained from the non-linear regression equation of the % scavenging ability versus extract concentration curve.
where A517reference is the absorbance of the reference test, and A517sample is the absorbance of the test containing the extract.
ABTS*+ scavenging assay
The ability of each extract to scavenge ABTS*+ was according to the method described by Re et al.[Citation29] ABTS*+ reagent was prepared by mixing equal volume of 7 mM ABTS*+ and 2.45 mM K2S2O8 aqueous solutions in amber reagent bottle, and incubating same in the dark at room temperature for 16 h. Thereafter, the absorbance of the reagent was adjusted to 0.7 ± 0.02 with 95% ethanol at 734 nm. A portion of 2.0 mL of the ABTS*+ reagent was mixed with 0.2 mL of the extract, and the mixture was kept in the dark at room temperature for 15 min. Subsequently, the absorbance was read at 734 nm in a UV/visible spectrophotometer. The ABTS*+ scavenging ability of each extract was later calculated from a standard curve prepared using Trolox, and expressed as Trolox equivalent antioxidant capacity (TEAC).
Preparation of rats’ liver homogenate for XO inhibition assay
Four adult male Wistar strain albino rats weighing of 200 ± 10 g were used to prepare liver homogenate that served as the source of XO for the XO inhibition assay. The rats were acquired from the experimental animal breeding unit of the Department of Veterinary Medicine, University of Ibadan, Nigeria. The rats were given humane treatment by following the guidelines specified in the public health service policy on humane care and use of laboratory animals of the National Academy of Science, USA.[Citation30] The rats were initially allowed to acclimatize under ambient laboratory conditions for 7 days, during which they fed on commercial rodent feed and table water ad libitum. Subsequently, liver homogenate was prepared following the method previously described by Nakamura et al.[Citation31] Briefly, the rats were anaesthetized mildly with ether and then sacrificed. The liver tissue was quickly harvested, washed in ice-cold 0.15 M KCl, and then blotted dry with filter paper. One gram portion of it was homogenized in 9 mL ice-cold 50 mM Tris–HCl buffer (pH 7.4) containing 1 mM ethylene diamine tetraacetic acid. Thereafter, the homogenate was sonicated twice under ice-cold condition for 30 s and centrifuged at 10,000 × g for 20 min at 4°C. The supernatant was decanted and used immediately as the source of the XO for the XO inhibition assay.
XO inhibition assay
The method reported by Umamaheswari et al.[Citation32] was followed to determine the ability of each extract to inhibit XO, with slight modification. Briefly, 300 μL of 50 mM sodium phosphate buffer (pH 7.5), 100 μL of various concentrations (20, 40, 60, and 80 μg/mL) of each extract in dimethyl sulphoxide (DMSO), 100 μL of the freshly prepared liver tissue homogenate, and 100 μL of distilled water were mixed in test tube. The mixture was pre-incubated at 37°C for 15 min, and thereafter 200 μL of freshly prepared 0.15 mM of xanthine solution (substrate) was added. The mixture was incubated at 37°C for 30 min, following the reaction was terminated with the addition of 200 μL of 0.5 M HCl. Subsequently, the absorbance was read at 295 nm in a UV spectrophotometer against a reagent blank containing the phosphate buffer in the place of the liver tissue homogenate. Allopurinol was used as a positive control, while a reference test containing 100 μL of DMSO in the place of the extract was included in the assay in order to obtain the maximum uric acid formed under the experimental conditions. The percentage inhibition of XO by each extract was calculated, and the IC50 was obtained from the non-linear regression equation of the % inhibition versus extract concentration curve.
where A295reference is the absorbance of the reference test without the extract, and A295sample is the absorbance of test extract.
ACE inhibition assay
The method reported by Cushman and Cheung[Citation33] was followed to determine the ability of each extract to inhibit ACE. Briefly, 50 μL of various concentrations (15, 30, 45, and 60 μg/mL) of the extract was mixed with 50 μL of ACE solution (4 mU/mL), and the resulting mixture was incubated at 37°C for 15 min. Next, 150 μL of 8.33 mM of the substrate (hippuryl-histidyl-leucine) in 125mM Tris–HCl buffer (pH 8.3) was added to the reaction mixture, and this was incubated at 37°C for 30 min. Thereafter, the reaction was terminated with the addition of 250 μL of 1 M HCl to the mixture, and the hippuric acid (HA) produced was extracted with 1.5 mL of ethyl acetate. The ethyl acetate layer of the mixture was separated by centrifugation, and 1.0 mL portion of it was dispensed into a clean test tube in which it was evaporated to dryness in a hot-air oven. The HA residue in the test tube was dissolved in 1.0 mL of deionized water, and its absorbance was read in a UV spectrophotometer at 228 nm. A positive control test containing 64 nmol/L captopril and a reference test (both without the extract) were included in the assay. The percentage inhibition of ACE by each extract was calculated, and the IC50 was obtained from the non-linear regression equation of the % inhibition versus extract concentration curve.
where A228reference is the absorbance of the reference without the extract, and A228sample is the absorbance of test containing the extract.
Statistical analysis
Results of replicate experiments were presented as mean ± SD. Analysis of variance was carried out on the result data at 95% confidence level using SPSS statistical software package, version 17.
Results and discussion
The HPLC profiles of the phenolic acids and flavonoids constituents of raw, roasted, and boiled B. eurycoma seeds extracts are shown in , , and , respectively. Raw seeds () contained gallic acid (retention time – tR = 9.85 min, peak 1), caffeic acid (tR = 22.53 min, peak 2), ellagic acid (tR = 31.45 min, peak 3), rutin (tR = 40.13 min, peak 4), quercetin (tR = 49.06 min, peak 5), and luteolin (tR = 60.11 min, peak 6). Roasted seeds contained gallic acid (peak 1), ellagic acid (peak 3), rutin (peak 4), and quercetin (peak 5); while boiled seeds contained gallic acid (peak 1), rutin (peak 4), and quercetin (peak 5).
Figure 1. Representative high-performance liquid chromatography profiles of raw (A), roasted (B), and boiled (C) B. eurycoma seeds. Gallic acid (peak 1), caffeic acid (peak 2), ellagic acid (peak 3), rutin (peak 4), quercetin (peak 5), and luteolin (peak 6).
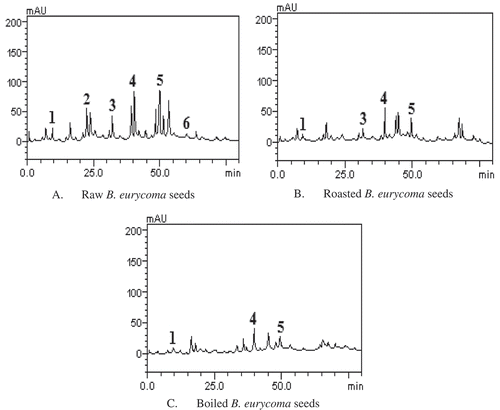
The levels of the various phenolic acids and flavonoids are presented in . In the raw seeds, phenolic acids were present in the order of caffeic > ellagic > gallic acids; while flavonoids were present in the order of quercetin > rutin > luteolin. In a previous study, the presence of these phenolic acids and flavonoids except luteolin in raw B. eurycoma seeds flour had been reported.[Citation1] However, the levels of the various phenolic acids (gallic acid: 1.04 ± 0.05; caffeic acid: 2.95 ± 0.01; ellagic acid: 2.18 ± 0.01 mg/g) and flavonoids (rutin: 4.57 ± 0.03; quercetin: 4.61 ± 0.01 mg/g) detected in the raw seeds in this study were generally lower than levels (gallic acid: 1.17 ± 0.03; caffeic acid: 18.13 ± 0.02; ellagic acid: 9.26 ± 0.01; rutin: 5.92 ± 0.01; quercetin: 6.04 ± 0.01 mg/g) earlier reported.[Citation1] These differences may be attributed to variations in biotic and abiotic factors, time of sample collection, as well as variation in sample extraction method used, which may all affect the levels of phytochemicals in the seeds.[Citation34,Citation35]
Table 1. Phenolic acids and flavonoids constituents of raw, roasted, and boiled B. eurycoma seeds.
Relative to the raw seeds, the two processing methods used (roasting and boiling) resulted in significant (p < 0.05) decrease in the levels of gallic acid, rutin, and quercetin. Ellagic acid reduced significantly (p < 0.05) in the roasted seeds, but it was not detected in the boiled seeds. The result further shows that both roasting and boiling methods led to total loss of caffeic acid and luteolin as these were not detected in both the roasted and boiled seeds. Although there is no previous literature on loss of phenolic compounds in B. eurycoma seeds due to heat treatments, our findings are consistent with those of similar studies on tree nuts and vegetables. Lemos et al.[Citation36] reported reductions in the levels of some phenolic acids, including gallic, caffeic, and ellagic acids in roasted baru nut, relative to the raw nuts. Similarly, Gębczyński and Lisiewska[Citation37] reported that boiling resulted in the decrease of the levels of phenolic acids and flavonoids in broccoli. The reduction in the levels or the absolute loss of some the phenolic acids and flavonoids observed in this study may be due to their thermal decomposition as polyphenols are known to get degraded easily when subjected to heat treatment.[Citation38] Phenolic acids such as caffeic acids are known to volatilize easily during heating for a long period.[Citation36] Flavonoids and ellagic acid are susceptible to heat, possibly due to their highly hydroxylated structure, which is vulnerable to redox reactions, thereby promoting their losses during heat treatment.[Citation36]
For the roasted seeds, Maillard reactions that possibly took place at the roasting temperature (150°C) might have contributed to the decrease of the levels of the phenolic acids and flavonoids.[Citation39] More of the phenolic acids and flavonoids were lost in boiled seeds than in the roasted seeds. This may be due to heat-induced oxidation and leaching of the water-soluble phenolics into water during boiling.[Citation39,Citation40] It is also possible that chemical rearrangements involving binding of the phenolic compounds with other organic substances during boiling resulted in the decrease of the levels of the phenolic acids and flavonoids.[Citation41] The report of Francisco et al.[Citation42] had earlier indicated that different types of phenolic compounds even within the same group are affected differently by the same cooking method. Thus, the differential reductions in the quantities of the individual phenolic acids and flavonoids after boiling may be a reflection of variations in their individual thermostability and solubility in hot water.[Citation43]
The free radical scavenging abilities of raw, roasted, and boiled B. eurycoma seeds, as tested using DPPH* and ABTS*+ scavenging assays, are presented in . The half-maximal scavenging concentration (SC50) of raw seed (25.10 ± 1.39 μg/mL) against DPPH* was significantly (p < 0.05) lower than those of roasted and boiled seeds (27.38 ± 1.48 and 48.06 ± 2.03 μg/mL, respectively). These results clearly indicate that both roasting and boiling methods decreased the free radical scavenging ability of the seeds since lower SC50 values represent stronger DPPH* scavenging ability. However, ascorbic acid (SC50: 7.36 ± 0.74 μg/mL), used as a reference antioxidant in this study, exhibited stronger DPPH* scavenging activity than the raw seeds. Similarly, the ABTS*+ scavenging ability of the seeds decreased significantly from 1.77 ± 0.20 mmol TEAC/g in the raw seeds to 1.54 ± 0.07 and 0.83 ± 0.02 mmol TEAC/g in the roasted and boiled seeds, respectively.
Table 2. DPPH* SC50 and ABTS*+ scavenging abilities of raw, roasted, and boiled B. eurycoma seeds extract.
The DPPH* and ABTS*+ scavenging abilities (SC50: 25.10 ± 1.39 μg/mL and 1.77 ± 0.20 mmol TEAC/g, respectively) of the raw seeds observed in this study are much stronger when compared with the DPPH* and ABTS*+ scavenging abilities (IC50: 5.05 ± 0.64 mg/mL and 29.78 ± 0.07 µmol TE/g, respectively) previously reported for methanol extract of B. eurycoma seed flour.[Citation7] As stated earlier, variations in biotic and abiotic factors, time of sample collection, and method of sample extraction may have contributed to the difference in the free radical scavenging potential we observed, relative to the previous report.[Citation34,Citation35] The decrease in the DPPH* and ABTS*+ scavenging potentials of the seeds due to roasting and boiling observed in this study is consistent with the findings of other researchers. Rawson et al.[Citation39] reported a decrease in the DPPH* scavenging ability of fennel bulb due to boiling and roasting. Similarly, Girgin and El[Citation44] reported a decrease in DPPH* and ABTS*+ scavenging ability of cauliflower due to boiling, while Borges et al.[Citation45] also reported a decrease in the DPPH* scavenging potential of cashew nut due to roasting. Since the free radical scavenging abilities of the roasted and boiled seeds decreased in a similar trend as the levels of the individual phenolic acids and flavonoids, these phenolics might be the major contributor to the antioxidant activity of the seeds. Phenolic acids and flavonoids have unique structures that confer them with redox properties, enabling them to act as hydrogen donors, singlet oxygen quenchers, and reducing agents.[Citation46] Rawson et al.[Citation39] noted that loss in antioxidant capacity is due to the chemical oxidation of polyphenolic compounds during thermal processing treatments.
The abilities of the raw, roasted, and boiled seeds extracts to inhibit XO and ACE, expressed as their half-maximal inhibitory concentrations (IC50), are presented in . The IC50 of the seeds against XO increased from 45.17 ± 2.14 μg/mL in the raw seeds to 46.58 ± 2.37 and 60.74 ± 2.96 μg/mL in the roasted and boiled seeds, respectively. This trend indicates a decreasing order in the XO inhibitory potential of the seeds due to roasting and boiling. Allopurinol, which was used as a reference XO inhibitor in this study, inhibited XO more than the raw and thermally processed seeds. However, the IC50 of the raw seeds against XO is comparable with those of Olea europaea leaf (IC50: 42 μg/mL)[Citation47] and Tetrapleura tetraptera fruit (IC50: 45.71 ± 1.44 μg/mL),[Citation48] also against XO. By inhibiting XO, raw B. eurycoma seeds may be useful for retarding the production of uric acid, and consequently, preventing hyperuricaemia and gout since the overactivity of XO contributes to the development of these two conditions.[Citation12]
Table 3. IC50 of raw, roasted, and boiled B. eurycoma seeds extracts against xanthine oxidase (XO) and angiotensin 1-converting enzyme activities (ACE).
Similarly, the IC50 of the seeds against ACE increased from 29.08 ± 1.74 μg/mL in the raw seeds to 32.87 ± 1.81 and 48.93 ± 2.15 μg/mL in the roasted and boiled seeds, respectively, indicating a decreasing order in the ACE inhibitory potential of the seeds following roasting and boiling treatments. Captopril, the reference ACE inhibitor in this study, had the strongest ACE effect (IC50: 4.75 ± 0.15 μg/mL). Whereas the raw B. eurycoma seeds had a stronger ACE inhibitory potency than Ocimum gratissimum (IC50: 56.63 ± 3.12 μg/mL),[Citation49] it had a weaker ACE inhibitory effect than guava leaves (IC50: 21.06 ± 2.04 μg/mL).[Citation10] Raw B. eurycoma seeds extract may therefore be useful for preventing excessive production of angiotensin II (a vasoconstrictor) through the inhibition of ACE, which is an important clinical approach for treating and managing hypertension.[Citation9]
The XO and ACE inhibitory effects of the seeds extracts may be attributed to their constituent phenolic acids and flavonoids. Previous studies had shown that plants extracts rich in these two classes of polyphenolic compounds, including cocoa and guava leaves, inhibited XO and ACE.[Citation10,Citation50] Thus, the decrease in the XO and ACE inhibitory capacities of the roasted and boiled seeds is a reflection of the decrease in the levels or the outright loss of some the phenolic acids and flavonoids constituents following these two thermal processing methods.
Conclusion
Raw B. eurycoma seeds contained major phenolic acids and flavonoids, including caffeic acid, ellagic acid, rutin, and quercetin. The seeds extract strongly scavenged free radicals (DPPH* and ABTS*+) and inhibited XO and ACE. Roasting and boiling led to decrease in the levels or outright loss of some the phenolic acids and flavonoids; and in the free radical scavenging, and XO and ACE inhibitory effects of the seeds. However, roasted seeds retained higher levels of the phenolic acids and flavonoids, and displayed stronger free radical scavenging, and XO and ACE inhibitory effects than the boiled seeds. Hence, raw B. eurycoma seeds may be useful in retarding the production of uric acid and angiotensin II, through XO and ACE inhibition, respectively, which are important strategies for managing gout and hypertension, respectively. Roasting of the seeds is recommended as a more valuable processing method than boiling, for the retention of the phenolic constituents and bioactivities, for optimum health benefits.
References
- Irondi, A. E.; Oboh, G.; Akindahunsi, A. A.; Boligon, A. A.; Athayde, M. L. Phenolics Composition and Antidiabetic Property of Brachystegia Eurycoma Seed Flour in High-Fat Diet, Low-Dose Streptozotocin-Induced Type 2 Diabetes in Rats. Asian Pac. J. Trop. Dis. 2015, 5 (Suppl 1), S159–S165.
- Groenendijk, P.; Bongers, F.; Zuidema, P. A. Using Tree-Ring Data to Improve Timber-Yield Projections for African Wet Tropical Forest Tree Species. For. Ecol. Manage. 2017, 400, 396–407.
- Uhegbu, F. O.; Onwuchekwa, C. C.; Iweala, E. E. J.; Kanu, I. Effect Ofprocessing Methods on the Nutritive and Antinutritive Properties of Seeds of Brachystegia Eurycoma and Detarium Microcarpum from Nigeria. Pak. J. Nutr. 2009, 8 (4), 316–320.
- Ikegwu, O. J.; Okechukwu, P. E.; Ekumankana, E. O. Physico-Chemical and Pasting Characteristics of Flour and Starch from Achi Brachystegia Eurycoma Seed. J. Food Technol. 2010, 8 (2), 58–66.
- Igwenyi, I. O.; Azoro, B. N. Proximate and Phytochemical Compositions of Four Indigenous Seeds Used as Soup Thickeners in Ebonyi State Nigeria. J. Environ. Science, Toxicol. Food Technol. 2014, 8 (6), 35–40.
- Ndukwu, M. C. Determination of Selected Physical Properties of Brachystegia Eurycoma Seeds. Res. Agric. Eng. 2009, 55 (4), 165–169.
- Irondi, A. E.; Oboh, G.; Akindahunsi, A. A. Methanol Extracts of Brachystegia Eurycoma and Detarium Microcarpum Seeds Flours Inhibit Some Key Enzymes Linked to the Pathology and Complications of Type 2 Diabetes in Vitro. Food Sci. Hum. Wellness 2015, 4, 162–168.
- Oomah, B. D.; Corbé, A.; Balasubramanian, P. Antioxidant and Anti-Inflammatory Activities of Bean (Phaseolus Vulgaris L.) Hulls. J. Agric. Food Chem. 2010, 58 (14), 8225–8230.
- Villiger, A.; Sala, F.; Suter, A.; Butterweck, V. In Vitro Inhibitory Potential of Cynara Scolymus, Silybum Marianum, Taraxacum Officinale, and Peumus Boldus on Key Enzymes Relevant to Metabolic Syndrome. Phytomedicine 2015, 22, 138–144.
- Irondi, E. A.; Agboola, S. O.; Oboh, G.; Boligon, A. A.; Athayde, M. L.; Shode, F. O. Guava Leaves Polyphenolics-Rich Extract Inhibits Vital Enzymes Implicated in Gout and Hypertension in Vitro. J. Intercult. Ethnopharmacol. 2016, 5 (2), 122–130.
- Berry, C. E.; Hare, J. M. Xanthine Oxidoreductase and Cardiovascular Disease: Molecular Mechanisms and Pathophysiological Implications. J. Physiol. 2004, 555, 589–606.
- Kramer, H. M.; Curhan, G. The Association between Gout and Nephrolithiasis: The National Health and Nutrition Examination Survey III, 1988–1994. Am. J. Kidney Dis. 2002, 40, 37–42.
- Mazzali, M.; Hughes, J.; Kim, Y. G.; Jefferson, J. A.; Kang, D. H.; Gordon, K. L.; Lan, H. Y.; Kivlighn, S.; Johnson, R. J. Elevated Uric Acid Increases Blood Pressure in the Rat by a Novel Crystalin-Dependent Mechanism. Hypertension 2001, 38, 1101–1106.
- Weseler, A. R.; Bast, A. Oxidative Stress and Vascular Function: Implications for Pharmacologic Treatments. Curr. Hypertens. Rep. 2010, 12, 154–161.
- Eriksson, U.; Danilczyk, U.; Penninger, J. M. Just the Beginning: Novel Functions for Angiotensin Converting Enzymes. Curr. Biol. 2002, 12, R745–R752.
- Ferrario, C. M.; Strawn, W. B. Role of the Renin-Angiotensin-Aldosterone System and Proinflammatory Mediators in Cardiovascular Disease. Am. J. Cardiol. 2006, 98 (1), 121–128.
- Singh, J. A.; Reddy, S. G.; Kundukulam, J. Risk Factors for Gout and Prevention: A Systematic Review of the Literature. Curr. Opin. Rheumatol. 2011, 23, 192–202.
- Emmerson, B. T. The Management of Gout. New England J. Med. 1996, 334, 445–451.
- Thurman, J. M.; Schrier, R. W. Comparative Effects of Angiotensin Converting Enzyme Inhibitors and Angiotensin Receptor Blockers on Blood Pressure and the Kidney. Am. J. Med. 2003, 114, 588–598.
- Khoo, B. P.; Leow, L. H. A Review of Inpatients with Adverse Drug Reactions to Allopurinol. Singapore Med. J. 2000, 41 (4), 156–160.
- Vyssoulis, G. P.; Karpanou, E. A.; Papavassiliou, M. V. Side Effects of Antihypertensive Treatment with ACE Inhibitors. Am. J. Hypertens. 2001, 14, 114–115.
- Ikegwu, O. J.; Okechukwu, P. E.; Ekumankama, E. O.; Okorie, P. A.; Odo, M. O. Modelling the Effect of Toasting Time on the Functional Properties of Brachystegia Eurycoma Flour. Niger. Food J. 2013, 31 (1), 108–114.
- Dini, I.; Tenore, G. C.; Dini, A. Antioxidant Compound Contents and Antioxidant Activity before and after Cooking in Sweet and Bitter Chenopodium Quinoa Seeds. LWT Food Sci. Technol. 2010, 43, 447–451.
- Abbès, F.; Kchaou, W.; Blecker, C.; Ongena, M.; Lognay, G.; Attia, H.; Besbes, S. Effect of Processing Conditions on Phenolic Compounds and Antioxidant Properties of Date Syrup. Ind. Crops Prod. 2013, 44, 634–642.
- Kuo, C.; Kao, E.; Chan, K.; Lee, H.; Huang, T.; Wang, C. Hibiscus Sabdariffa L. Extracts Reduce Serum Uric Acid Levels in Oxonate-Induced Rats. J. Funct. Foods 2012, 4, 375–381.
- Adefegha, S. A.; Oboh, G.; Molehin, O. R.; Saliu, J. A.; Athayde, M. L.; Boligon, A. A. Chromatographic Fingerprint Analysis, Acetylcholinesterase Inhibitory Properties and Antioxidant Activities of Red Flower Rag Leaf (Crassocephalum Crepidioides) Extract. J. Food Biochem. 2016, 40, 109–119.
- Fernandes, C. E. F.; Kuhn, F.; Scapinello, J.; Lazarotto, M.; Bohn, A.; Boligon, A. A.; Athayde, M. L.; Zanatta, M. S.; Zanatta, L.; Dal Magro, J.; Oliveira, J. V. Phytochemical Profile, Antioxidant and Hypolipemiant Potential of Ilex Paraguariensis Fruit Extracts. Ind. Crops Prod. 2016, 81, 139–146.
- Cervato, G.; Carabelli, M.; Gervasio, S.; Cittera, A.; Cazzola, R.; Cestaro, B. Antioxidant Properties of Oregano (Origanum Vulgare) Leaf Extracts. J. Food Biochem. 2000, 24, 453–465.
- Re, R.; Pellegrini, N.; Proteggente, A.; Pannala, A.; Yang, M.; Rice-Evans, C. Antioxidant Activity Applying an Improved ABTS Radical Cation Decolorization Assay. Free Radic. Biol. Med. 1999, 26, 1231–1237.
- Public Health Service (PHS). Public Health Service Policy on Humane Care and Use of Laboratory Animals, (PL 99-158. Health Research Extension Act, 1985); US Department of Health and Human Services: Washington, DC, 1996.
- Nakamura, T.; Ohta, Y.; Ikeno, K.; Ohashi, K.; Ikeno, T. Protective Effect of Repeatedly Pre-Administered Brazilian Propolis Ethanol Extract against Stress-Induced Gastric Mucosal Lesions in Rats. Evid. Based Complement. Alternat. Med. 2014, 2014, 1–10.
- Umamaheswari, M.; Kumar, K. A.; Somasundaram, A.; Sivashanmugam, T.; Subhadradevi, V.; Ravi, T. K. Xanthine Oxidase Inhibitory Activity of Some Indian Medical Plants. J. Ethnopharmacol. 2007, 109, 547–551.
- Cushman, D. W.; Cheung, H. S. Spectrophotometric Assay and Properties of the Angiotensin I-Converting Enzyme of Rabbit Lung. Biochem. Pharmacol. 1971, 20, 1637–1648.
- Mpofu, A.; Sapirstein, H. D.; Beta, T. Genotype and Environmental Variation in Phenolic Content, Phenolic Acid Composition, and Antioxidant Activity of Hard Spring Wheat. J. Agric. Food Chem. 2006, 54, 1265–1270.
- Braga, T. V.; Dores, R. G.; Ramos, C. S.; Evangelista, F. C. G.; Tinoco, L. M.; Varotti, F.; Carvalho, M.; Sabino, A. Antioxidant, Antibacterial and Antitumor Activity of Ethanolic Extract of the Psidium Guajava Leaves. Am. J. Plant Sci. 2014, 5, 3492–3500.
- Lemos, M. R. B.; Siqueira, E. M.; Arruda, S. F.; Zambiazi, R. C. The Effect of Roasting on the Phenolic Compounds and Antioxidant Potential of Baru Nuts [Dipteryx Alata Vog.]. Food Res. Int. 2012, 48, 592–597.
- Gębczyński, P.; Lisiewska, Z. Comparison of the Level of Selected Antioxidative Compounds in Frozen Broccoli Produced Using Traditional and Modified Methods. Innovative Food Sci. Emerg. Technol. 2006, 7, 239–245.
- Podsedek, A.; Sosnowska, D.; Redzynia, M.; Koziołkiewicz, M. Effect of Domestic Cooking on the Red Cabbage Hydrophilic Antioxidants. Int. J. Food Sci. Technol. 2008, 43, 1770−1777.
- Rawson, A.; Hossain, M. B.; Patras, A.; Tuohy, M.; Brunton, N. Effect of Boiling and Roasting on the Polyacetylene and Polyphenol Content of Fennel (Foeniculum Vulgare) Bulb. Food Res. Int. 2013, 50, 513–518.
- López-Martínez, L. X.; Leyva-López, N.; Gutiérrez-Grijalva, E. P.; Heredia, J. B. Effect of Cooking and Germination on Bioactive Compounds in Pulses and Their Health Benefits. J. Funct. Foods. 2017. DOI:10.1016/j.jff.2017.03.002.
- Volf, I.; Ignat, I.; Neamtu, M.; Popa, V. I. Thermal Stability, Antioxidant Activity, and Photo-Oxidation of Natural Polyphenols. Chem. Pap. 2014, 68 (1), 121–129.
- Francisco, M.; Velasco, P.; Moreno, D. A.; García-Viguera, C.; Cartea, M. E. Cooking Methods of Brassica Rapa Affect the Preservation of Glucosinolates, Phenolics and Vitamin C. Food Res. Int. 2010, 43, 1455–1463.
- Irondi, A. E.; Akintunde, J. K.; Agboola, S. O.; Boligon, A. A.; Athayde, M. L. Blanching Influences the Phenolics Composition, Antioxidant Activity, and Inhibitory Effect of Adansonia Digitata Leaves Extract on α-amylase, α-glucosidase, and Aldose Reductase. Food Sci. Nutr. 2017, 5 (2), 233–242.
- Girgin, N.; El, S. N. Effects of Cooking on in Vitro Sinigrin Bioaccessibility, Total Phenols, Antioxidant and Antimutagenic Activity of Cauliflower (Brassica Oleraceae L. Var. Botrytis). J. Food Compos. Anal. 2015, 37, 119–127.
- Borges, T. H.; Rodrigues, N.; De Souza, A. M.; Pereira, J. A. Effect of Different Extraction Conditions on the Antioxidant Potential of Baru Almonds (Dipteryx Alata Vog.): Comparison to Common Nuts from Brazil. J. Food Nutr. Res. 2014, 53, 180–188.
- Chang, S. T.; Wu, J. H.; Wang, S. Y.; Kang, P. L.; Yang, N. S.; Shyur, L. F. Antioxidant Activity of Extracts from Acacia Confuse Bark and Heartwood. J. Agric. Food Chem. 2001, 49, 3420–3424.
- Flemmig, J.; Kuchta, K.; Arnhold, J.; Rauwald, H. W. Olea Europaea Leaf (Ph. Eur.) Extract as Well as Several of Its Isolated Phenolics Inhibit the Gout-Related Enzyme Xanthine Oxidase. Phytomedicine 2011, 18, 561–566.
- Irondi, E. A.; Oboh, G.; Agboola, S. O.; Boligon, A. A.; Athayde, M. L. Phenolics Extract of Tetrapleura Tetraptera Fruit Inhibits Xanthine Oxidase and Fe2+-Induced Lipid Peroxidation in the Kidney, Liver, and Lungs Tissues of Rats in Vitro. Food Sci. Hum. Wellness 2015, 5 (1), 17–23.
- Shaw, H.-M.; Wu, J.-L.; Wang, M.-S. Antihypertensive Effects of Ocimum Gratissimum Extract: Angiotensin-Converting Enzyme Inhibitor in Vitro and in Vivo Investigation. J. Funct. Foods 2017, 35, 68–73.
- Irondi, A. E.; Olanrewaju, S.; Oboh, G.; Olasupo, F.; Boligon, A. A. Inhibitory Potential of Cocoa Leaves Polyphenolics-Rich Extract on Xanthine Oxidase and Angiotensin 1-Converting Enzyme. J. Biol. Active Prod. Nat. 2017, 7 (1), 39–51.