ABSTRACT
The objective of this study was to determine the effects of charge-carrying amino acids (Lys, Arg, Asp, and Glu) on the textural and rheological properties of potato starch. Results indicated that the hardness, springiness, and gel strength of potato starch gels decreased with acidic amino acids but increased with basic amino acids. Basic and acidic amino acids demonstrated opposite effects on the texture of starch gels. Both acidic and basic amino acids decreased the G′ and G′′ values of potato starch in dynamic rheological measurements. The starch with acidic amino acids had greater decreases in G′ and G′′ compared with the starch with basic ones. Potato starch with amino acids showed more shear-thinning behavior than starch alone by steady shear measurements. There is the potential to produce starch-based foods with the desired characteristics by adding appropriate amino acids.
Introduction
Starch can greatly contribute to the textural properties of many foods and is widely used in food and industrial applications as a thickener, colloidal stabilizer, gelling agent, bulking agent, and water retention agent.[Citation1] Potato starch has a low gelatinization temperature and a high water-binding capacity,[Citation2–Citation4] which is partly due to its large granular size, mineral content, amylose/amylopectin ratio, and high content of starch phosphate esters.[Citation5] Moreover, phosphate monoesters in potato starch are negatively charged groups, and thus, the ionic repulsion generated by these groups can weaken the association among the molecules and increase the granule water-binding capacity, as well as swelling power.[Citation6] Therefore, the presence of charge-carrying amino acids might influence such repulsion between the starch granules, resulting in some changes in the physicochemical properties of potato starch.
Aspartic acid (Asp) and glutamic acid (Glu) with negative charges present acidity in solution, while lysine (Lys) and arginine (Arg) with positive charges show alkalinity. Asp and Glu might attract a lot of hydrogen ions in the water, while Lys and Arg might separate out a large number of hydroxyl ions. Starch could act as a weak acid-based ion exchanger, and thus, its cations tend to protect and stabilize the granule structure, while its anions are a gelatinizing agent and rupture hydrogen bonds.[Citation7,Citation8] Charge-carrying amino acids (Lys, Arg, Asp, and Glu) significantly decreased the swelling power, solubility, light transmittance, L* value and gel strength of potato starch but increased the syneresis during freeze–thaw treatment, while neutral amino acids (Phe and Met) did not cause any modification in potato starch gels.[Citation9] Moreover, charge-carrying amino acids have a remarkable influence on the pH environment of a starch suspension.[Citation10] The addition of positively or negatively charged amino acids had a strong effect on the pasting properties of rice starch compared to neutral ones.[Citation11] Native starch is often modified with various chemical reagents or through acid, alkali, enzymatic or hydrothermal treatments for more applications in foods and non-foods. Alkali treatment, such as sodium hydroxide, is commonly used in the production of many traditional food products, such as tortillas, waxy rice dumplings and yellow alkaline noodles, to improve their qualitative characteristics.[Citation12] The alkalizing agent plays an important role in the preparation of many traditional starch-based food products, such as the use of lime in the production of tortillas.[Citation13] The physicochemical properties of various botanical origin starches could be varied when they were treated with alkaline solution.[Citation14] Acid treatment also has an influence on the gelatinization behavior of starch. For example, starches used in gum candies are generally modified with hydrochloric acid, citric acid and lactic acid.[Citation15]
The rheological properties of food are closely related to food processing, storage and taste, and they are regarded as one of the most important aspects in terms of the quality of starch and starch-based foods.[Citation16] Until now, there was little information about the effect of charge-carrying amino acids on the rheological properties of potato starch. Therefore, the objective of this study was to investigate the effects of four charge-carrying amino acids (Lys, Arg, Asp, and Glu) on the textural and rheological properties of potato starch gel.
Materials and methods
Materials
Potato starch (Xueguan Starch Company, Ningxia, China), which contained moisture (13.49%), protein (0.11%), fat (0.21%), ash (0.13%), and carbohydrate (86.06%), as determined on a dry basis, was used after drying in the oven at 80°C for 24 h until the moisture content reached 6%. Amino acids included two positive charge-carrying amino acids (Lys and Arg) and two negative charge-carrying amino acids (Asp and Glu) (Biosharp, Seoul, Korea).
Preparation of potato starch gel
Amino acids (0, 0.05, 0.10, and 0.20 mol/kg on a starch weight basis) were weighed, placed into beakers with distilled water (100 mL) and then mixed with 6 g of potato starch, respectively. Potato starch gels (6%, w/v) with different levels of charge-carrying amino acids were prepared by heating the starch suspension at 95°C for 20 min with stirring, and then, they were poured into the weighing bottle (40 mm in diameter and 25 mm in depth). The samples were sealed with plastic wrap to prevent moisture loss and then stored at 4°C for 12 h. The control was starch gel without amino acids.
Instrumental texture profile analysis (TPA)
The texture profile analysis (TPA) was carried out by using the TA XT Plus Texture Analyzer (TA-XT2i, Stable Micro Systems, Surrey, UK) with a cylindrical probe (P/0.5). The deformation level was 40% of the original height of the sample at a speed of 1 mm/s with a 5 g force transducer.[Citation9] The gels were compressed twice. The hardness, springiness, cohesiveness, and gumminess were obtained from the TPA curves.[Citation17]
Gel strength
The penetration test of the potato starch gels was carried out using the texture analyzer (TA-XT2i, Stable Micro Systems, Surrey, UK) with a cylindrical probe (P/0.5). Starch gels were equilibrated at room temperature for 2 h prior to the test. The deformation level was 75% of the compression ratio of the original height at a speed of 1 mm/s and with a 5 g trigger force.[Citation18] The breaking force (g) and distance to rupture (mm) were obtained.
Rheological measurements
Frequency sweep
Frequency sweep measurements were carried out in a controlled stress rheometer (AR2000ex Rheometer, TA Instruments Ltd., New Castle, DE, USA) using the strain controlled mode and parallel plates. The paste samples (6%, w/v) were the same as above equilibrated to a 25°C temperature, after which the measurements for the viscoelastic behavior were acquired. The samples were loaded onto the plate (40 mm diameter, 1.0 mm gap), and a frequency sweep between 0.1 and 10 Hz was recorded at a strain amplitude of 1%, which was within the linear viscoelastic region. The mechanical spectra were obtained according to the dynamic modulus values G′ and G″ as a function of frequency.
Temperature sweep
Rheological measurements were carried out using an AR2000ex rheometer (TA Instruments Ltd., New Castle, DE, USA). Samples were placed in a parallel plate system (40 mm in diameter). The gap size was set at 1 mm for the potato starch suspension. The strain of 1% and the frequency of 5 rad/s were within the linear viscoelastic region and were used for all experiments. Potato starch suspensions of a 6% (w/v) concentration with different levels of charge-carrying amino acids, respectively, were mixed at room temperature and loaded directly onto the plate. The outer edge of the starch suspension was covered with a thin layer of silicone oil to minimize evaporation loss. The starch samples were measured during heating from 20 to 100°C at a rate of 5 °C/min and subsequently cooled from 100 to 20°C at the same rate. The storage modulus (G′) and loss modulus (G′′) of the samples were obtained.
Steady shear viscosity measurements
Samples of potato starch suspensions (6%, w/v) with amino acids were heated at 95°C for 20 min. Then, the paste was immediately transferred to the AR2000ex Rheometer (TA Instruments Ltd., New Castle, DE, USA) equipped with a parallel plate system (40 mm in diameter) at 25°C for the measurement of its steady shear rheological properties. The gap size was set at 1 mm. The exposed sample edge was covered with a thin layer of silicone oil to prevent evaporation during measurement. The flow curves were obtained by registering the shear stress at shear rates from 0.1 to 300 s−1 (forward) in 15 min and in 15 min from 300 to 0.1 s−1 (backward) at 25°C. Experimental data were described by the following power law equation[Citation19]:
where τ is the shear stress (Pa), γ is the shear (s−1), K is the consistency coefficient (Pa·sn), n is the flow behavior index, and R2 is the coefficient of determination (dimensionless). The software GraphPad Prism (GraphPad Prism 5 for windows, GraphPad Software, Inc., San Diego, CA, USA) was used to calculate those values (k, n and R2).
Statistical analysis
All experiments were replicated three times. The statistical analyses were performed using a SPSS package (SPSS 17.0 for Windows, SPSS Inc., Chicago, USA). Differences among the mean values of various treatments were compared using Duncan’s multiple range tests (p < 0.05).
Results and discussion
TPA properties
shows the hardness, springiness, cohesiveness and gumminess of potato starch gels with different charged amino acids. When acidic amino acids (Asp and Glu) were added at 0.05 mol/kg, the hardness values of potato starch gels were significantly (p < 0.05) increased compared with the control. However, with the greater addition of acidic amino acids, the hardness values of potato starch gels were remarkably decreased. The hardness of starch gels was increased by the addition of basic amino acids (Lys and Arg). As the concentrations of basic amino acids were increased, there was a greater increase in the hardness of potato starch gels. As the concentration of Arg increased from 0.1 to 0.2 mol/kg, the increase in hardness was significant (p < 0.05). In the potato starch, there are phosphate ester groups with negative charges, which tend to attract cations and repel anions.[Citation13] When basic amino acids (Lys and Arg) (pH ˃ 7) were added, the starch hydroxyl groups would have a greater propensity to combine with positively charged amino acids for electrostatic interactions, which resulted in the rigid network structure of the starch gel.[Citation13] Under the addition of acidic amino acids (Asp and Glu) (pH < 7), potato starch carried negatively charged phosphate ester groups and led to the mutual repulsion of negative charges in the starch molecules and amino acids, which weakened the potato starch gel. Moreover, acid treatment could retard the swelling of starch granules and weaken the gel network because the acid preferentially attacks the amorphous regions of starch granules, in which mainly amylose in gathered.[Citation20]
Figure 1. Effects of the four charge-carrying amino acids on the hardness, springiness, cohesiveness, and gumminess of potato starch gel. A: Hardness, B: Springiness, C: Cohesiveness, and D: Gumminess. Different letters indicated the mean values were significantly different (p < 0.05).
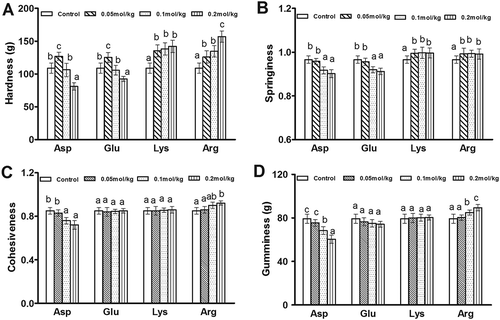
The springiness characterizes the extent the gel structure had broken down by the initial compression.[Citation21] The gel demonstrates a low springiness value when the gel structure is broken into many small pieces during the first TPA compression, and a high springiness value results from the gel being broken into few large pieces.[Citation17] Asp and Glu at low concentrations (0.05 mol/kg) had no significant effect (p > 0.05) on the springiness values of potato starch gels, but they decreased the springiness values of potato starch gels at higher concentrations (0.1 and 0.2 mol/kg) compared with the control. Lys and Arg could significantly increase the springiness of potato starch gels (p < 0.05). This might be due to that the negatively charged potato starch chains and the positively charged amino acids (Lys and Arg) attract each other, making the starch gel structure stronger and increasing the springiness. Meanwhile, the repulsion of acidic amino acids (Asp and Glu) and potato starch chains had the opposite effect, leading to a decrease in the springiness. Organic acids, such as citric acid and malic acid, were able to interact with the hydroxyl groups of the native cassava starch and act in the acid hydrolysis of starch molecules, reducing the connection of the starch chains.[Citation23] The addition of lactic and acetic acids into native and cross-linked wheat starches resulted in a softer gel texture with less adhesiveness, elasticity, cohesiveness, and gumminess.[Citation24] On the other hand, the addition of Na2CO3 or NaOH could significantly increase the stability of the starch granules and form a firm stable gel structure through electrostatic interactions between Na+ ions and starch hydroxyl groups.[Citation13]
Asp decreased the cohesiveness and gumminess values of potato starch gel, while Arg increased those values of potato starch gel, especially at 0.2 mol/kg. Glu and Lys had no significant effects on both values of potato starch gels. Asp and Arg, as the representatives of acidic and basic amino acids, could strongly affect the cohesiveness and gumminess characteristics of potato starch gels, consistent with the effects of the acid and alkali on the TPA behavior of starch gels.[Citation13,Citation20] Compared to Asp and Arg, the influences of Glu and Lys might be small because their solution pH values were closer to neutral, resulting in no change in both the cohesiveness and gumminess. Therefore, the addition of basic amino acids, especially more positively charged Arg, increased the hardness, springiness, and gumminess of potato starch gel, thus resulting in a more rigid elastic gel. The addition of acidic amino acids, especially at higher concentrations, led to a soft unstable starch gel.
Gel strength
shows the gel strength parameters of potato starch gels with different charge-carrying amino acids, including the breaking force, deformation and gel strength. The addition of charge-carrying amino acids significantly changed the breaking force and gel strength values (p < 0.05) of potato starch gels, while there was not a significant change in the deformation (p > 0.05). The breaking force and gel strength of starch gels with the addition of Asp and Glu were significantly decreased (p < 0.05) with the addition of 0.2 mol/kg. However, they were significantly (p < 0.05) increased as alkaline amino acids were added, with the exception of gels with Lys at concentrations of 0.05 and 0.1 mol/kg. This might be due to that the degree of dissociation of Lys was weak with the addition of a small amount, which led to the pH changes of the starch and amino acid mixed system to not be strong at higher concentrations. The main structural parameters involved in starch gel were the deformability of swollen starch particles and the amylose concentration of the continuous network. A higher amylose content would cause more retrogradation of the starch during gel formation and consequently result in a denser and firmer gel structure.[Citation3] Acidic amino acids (Asp and Glu) could decrease the swelling capacity but increase amylose leaching of starch granules.[Citation25] They decreased the content of amylose in starch granules, thus resulting in a decrease in starch gel strength. On the other hand, alkaline amino acids could increase the swelling and decrease the amylose leaching of starch granules,[Citation25] thus resulting in an increase in gel strength for the potato starch gel. The gel of acid-modified oat starch became less rigid but more elastic than the corresponding native starch gel.[Citation26] The two positive charge-carrying amino acids (Lys and Arg) and two negative charge-carrying amino acids (Asp and Glu) showed opposite effects on the gel strength of potato starch gels.
Temperature sweep in dynamic rheology
The dynamic modulus is known to be good characterization tool in studying the rheological properties of some polymeric solutions.[Citation27] Starch pastes, consisting of swollen granules wrapped in a three-dimensional network of gathered amylose chains, can be viewed as a complex system.[Citation28] The rheological properties of the gelatinized starch systems are determined by the interactions between the dispersed and continuous phases. All samples exhibited a sharp increase of G′ with heating temperatures of approximately 60°C, followed by a decline from the maximum of G′ during heating (). The starch granules absorbed a lot of water when subjected to high temperature (>50°C) and formed a stable network structure during heating.[Citation9] Such swelling was responsible for the increase of G′. Further heating above the temperature of the maximum storage modulus provided energy to break down the crystalline structure remaining in the swollen starch granules and increased the movement of amylose, causing the decrease of G′.[Citation29]
Figure 3. Effects of the four charge-carrying amino acids on the storage modulus (G′) and loss modulus (G″) of potato starch during heating.
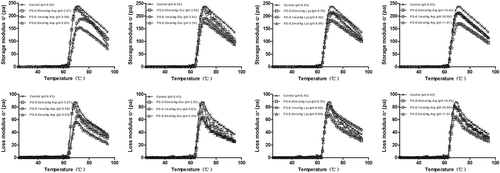
The addition of four charge-carrying amino acids (Lys, Arg, Asp, and Glu) decreased the maximum storage modulus (Gm′) and maximum loss modulus (Gm′′) compared with the control. For acidic amino acids (Asp and Glu), the maximum storage modulus and maximum loss modulus decreased with the addition of amino acids from 0.05 to 0.2 mol/kg. Both G′ and G′′ values showed similar behaviors to those of the control for potato starches with charge-carrying amino acids during heating. The gelatinization temperature, at which G′ was at its maximum, showed an evident increase when those amino acids were added into potato starch (). This might be related to the following two reasons. First, the Asp and Glu solutions were acidic (pH < 7), and potato starch could not resist acid hydrolysis. Therefore, its glycosidic bonds were easily hydrolyzed in the acidic environment.[Citation30] This resulted in the reduction of G′ and G′′ of potato starch with acidic amino acids. The degree of hydrolysis of the starch was increased with increasing concentrations of acidic amino acids, leading to decreases in the G′ and G′′ values of the starch system. The higher concentration of hydrochloric acid (1.0 mol/L) degraded both amylopectin and amylose to a greater extent compared with the other two lower concentrations (0.06 and 0.14 mol/L), resulting in decreases in the storage modulus (G′) and loss modulus (G′′) of the acid-thinned starch.[Citation31] Citric acid led to decreases in the G′ and G′′ for corn starch because of the hydrolysis of glucose chains.[Citation32] The two basic amino acids (Lys and Arg) could also affect the structure and physical behavior of starch. Most of the hydroxyl groups in the glucose units of the starch chain were ionized at high pH and in turn delayed starch agglomeration and retrogradation,[Citation33] thus leading to decreases in the G′ and G′′ values for starch pastes.
Second, amino acid groups also have an influence on the rheological properties of potato starch. Acidic amino acids (Asp and Glu) might result in a decrease of starch granule swelling,[Citation25] causing the decrease of G′. However, basic amino acids (Lys and Arg) could influence the ionization of the hydroxyl groups, resulting in the presence of negative charge with the repulsion of starch molecules, resulting in the ability of the potato starch granules to swell easily.[Citation34] Thus, basic amino acids facilitated the increase of water percolation within the granules, thus leading to increases of the G′ and G′′ values when the concentration of amino acids increased from 0.05 mol/kg to 0.2 mol/kg, but the G′ and G′′ values were still lower than the control. This probably resulted in electrostatic interactions between positively charged basic amino acids and negatively charged potato starch chains.[Citation9]
During cooling, the G′ showed a gradual increase, while G′′ showed a slight decline. There were lower G′ and G′′ values with the addition of charge-carrying amino acids compared to the control (). During cooling, there was a retrogradation of leached components and interaction among molecules remaining inside the granule, reinforcing the gel structure and leading to the increases in G′ and G′′. For both acidic amino acids (Asp and Glu) and basic amino acids (Lys and Arg), there is likely the hydrolysis of starch chains by acid and alkali,[Citation12] which deconstructed the network structure of the potato starch paste and tended to restrain the increases in G′ and G′′ compared to the control. Therefore, potato starch with both alkaline amino acids and acidic amino acids had lower G′ and G′′ values than the control during cooling.
Frequency sweep
The frequency sweep mode can demonstrate viscoelastic behaviors of starch pastes. shows the mechanical spectra of potato starch pastes with different charge-carrying amino acids. The rheological parameters of all of the samples, G′ and G″ showed significant variations for starch dispersions with different charge-carrying amino acids compared to the control. These results implied that the addition of charge-carrying amino acids could influence the viscoelastic properties of potato starch. Moreover, the G′ values were higher than the G′′ values throughout the accessible frequency range, while both G′ and G′′ were frequency dependent. There was no a crossover between both modulus values throughout the frequencies, and thus, potato starches with charge-carrying amino acids might be rheologically classified as having typical weak gel behavior.[Citation35]
Compared to the control, the addition of amino acids decreased the G′ value, showing increased weakening in the starch structure (). This was probably due to the formation of linking or hydrogen bonds among amino acids and hydroxyl groups of amylose in the starch–amino acid system, which reduced or prevented the interaction between amylose-amylose.[Citation36] When the acidic amino acids (Asp and Glu) were added to the starch, there were more hydrogen ions in the system and that decreased amylose-amylose associations, leading to the formation of a weaker starch paste network structure. On the other hand, the rheological behaviors of the starch system showed that it was strongly dependent on the concentration of leached amylose and also governed by the rigidity of the swollen granules. When basic amino acids (Lys and Arg) were added into potato starch, they brought more hydroxide ions into the starch granules, resulting in the increase of the molecular repulsion due to negative charges inside the granule.[Citation25] Thus, potato starch with basic amino acids became more unstable than the control, and the rigidity of the starch granules decreased, resulting in the decrease of G′. In the starch–amino acid system, either acidic or basic amino acids could reduce or prevent associations among the amylose by competing with amylose–amylose interactions that were crucial for short-time starch retrogradation. Hence, the molecular entanglement of the starch paste network structure was weakened with the addition of amino acids, resulting in the decrease of G′ during the frequency sweep.
However, the addition of charge-carrying amino acids had only a slight effect on the G″ values of potato starch (). The changes in G′ values were more evident with increasing contents of the charge-carrying amino acids. Compared with the control, the addition of amino acids decreased the G′ values. The decrease of the gap between the G′ and G″ values of starch/amino acid mixtures indicated that their storable energy reduced during the frequency sweep, which led to weaker elastic behavior.[Citation10]
Steady flow properties
demonstrates the consistency coefficient (K), flow behavior index (n) and coefficient of determination (R2) of the power law model for ascending and descending steady flow curves as well as the thixotropic area of potato starch with charge-carrying amino acids. The power-law model was fitted well for the flow curves of the rheological characteristics of the samples (R2 > 0.99; ). The addition of four charge-carrying amino acids increased the consistency coefficient values (K) of potato starch paste. The consistency coefficient, K, describes the consistency of the material, where a higher K value implies higher consistency.[Citation37] The starch molecular chains wrapped around each other, hindered the movement of starch molecules, and produced a viscosity resistance as starch gelatinization.[Citation38] Different types and concentrations of amino acids could influence the leaching out or aggregation of amylose, resulting in different consistency values. The addition of basic amino acids (Lys and Arg) could promote the swelling of the starch granules and amylose leaching out,[Citation25] which led to an increased consistency coefficient of starch paste. For acidic amino acids, they repelled the negatively charged potato starch chains, resulting in phase separation and increased concentrations in their respective micro-domains, which increased the overall consistency coefficients of the mixtures.[Citation39] However, with the addition of a high amino acid concentration (0.2 mol/kg), there were interactions between the amino acids and potato starch through hydroxyl and phosphate groups, reducing the possibility of amylose–amylose interactions.
Table 1. The rheological parameters of the power-law model for ascending and descending steady flow curves (shear rates: 0.1–300 s−1 and 300–0.1 s−1) and the thixotropic area of potato starch with charge-carrying amino acids.
The flow behavior index (n) is dimensionless and reflects the closeness to Newtonian flow, sometimes called the power law index.[Citation40] When the n is 1, it corresponds to a Newtonian fluid, and a lower n value reflects a higher degree of pseudoplasticity of the paste. The flow behavior index (n) ranged from 0.332 to 0.468 (n < 1), indicating that all of the starch pastes tended toward a non-Newtonian fluid with pseudoplastic behavior (). Pseudoplastic behavior refers to a decrease in the viscosity as the shear rate increases (shear thinning). Under shear stress, the intertwined amylose molecular chains had a straight orientation and the entanglement between starch chains was reduced, resulting in a decrease in the apparent viscosity. This phenomenon was related to the shear thinning or pseudoplastic behavior.[Citation41] All pastes exhibited pseudoplastic behavior due to their lower values of n (). The n values decreased when charge-carrying amino acids were added. This implied that the paste showed higher pseudoplasticity than the control due to weaker entanglements. Gelatinized potato starch with charge-carrying amino acids exhibited less gel-like behavior than gelatinized potato starch, which was consistent with the results obtained from viscoelastic measurements ().
The paste of potato starch and charge-carrying amino acids exhibited clockwise hysteresis loops, suggesting the thixotropic behavior of the paste. Compared with the control, the hysteresis loop area of the potato starch paste was increased with the addition of the four amino acids, illustrating that the thixotropy of potato starch paste increased. With the increase of amino acids, the hysteresis loop area was gradually reduced, except for Arg, which might be due to its higher basic characteristic compared to other charge-carrying amino acids. The clockwise hysteresis loop was due to that the structure was broken down by the shear and the newly developed texture was less resistant to shear, resulting in the decrease of the apparent viscosity in the down curve.[Citation39] This implies that the potato starch paste structure was not stable and was easily damaged with the addition of amino acids. When the shear force increased, such structural damage was more obvious. A greater addition of charge-carrying amino acids turned the potato starch paste closer to a pseudoplastic fluid compared to the control.
Conclusions
The results showed that potato starch with charge-carrying amino acids possessed different textural and rheological properties. The hardness and gel strength values of potato starch gels were increased significantly with the addition of Arg and Lys but decreased by Asp and Glu. As the concentrations of the charged amino acids were increased, those changes were increased. During heating, the amino acids (Arg, Lys, Asp and Glu) decreased the G′ and G′′ values of potato starch. The addition of charge-carrying amino acids decreased the G′ values and G″ values of starch paste in the frequency sweep. Potato starch with charge-carrying amino acids exhibited more shear-thinning flow behavior than native starch, indicating that its structure was not stable and easily damaged. In general, charge-carrying amino acids had a variety of influences on the textural and rheological properties of the starch-based product. There is a potential to produce starch-based foods with desired characteristics by adding appropriate amino acids, such as potato starch noodles with a designated texture.
Additional information
Funding
References
- Singh, J.; Kaur, L.; McCarthy, O. J. Factors Influencing the Physico-Chemical, Morphological, Thermal and Rheological Properties of Some Chemically Modified Starches for Food applications—A Review. Food Hydrocoll. 2007, 21, 1–22. DOI:10.1016/j.foodhyd.2006.02.006.
- Cai,; Hong, Y.; Gu, Z.; Zhang, Y. The Effect of Electrostatic Interactions on Pasting Properties of Potato Starch/Xanthan Gum Combinations. Food Res. Int. 2011, 44, 3079–3086.
- Sandhu, K. S.; Kaur, M. Mukesh, Studies on Noodle Quality of Potato and Rice Starches and Their Blends in Relation to Their Physicochemical, Pasting and Gel Textural Properties. LWT Food Sci. Technol. 2010, 43, 1289–1293. DOI:10.1016/j.lwt.2010.03.003.
- Zaidul, I. S. M.; Absar, N.; Kim, S. J.; Suzuki, T. Karim, A.A.; Yamauchi, H.; Noda, T. DSC Study of Mixtures of Wheat Flour and Potato, Sweet Potato, Cassava, and Yam Starches. J. Food Eng. 2008, 86, 68–73. DOI: 10.1016/j.jfoodeng.2007.09.011.
- Kaur, A.; Singh, N.; Ezekiel, R.; Guraya, H. S. Physicochemical, Thermal and Pasting Properties of Starches Separated from Different Potato Cultivars Grown at Different Locations. Food Chem. 2007, 101, 643–651. DOI:10.1016/j.foodchem.2006.01.054.
- Zhou, H.; Wang, C.; Shi, L.; Chang, T.; Yang, H.; Cui, M. Effects of Salts on Physicochemical, Microstructural and Thermal Properties of Potato Starch. Food Chem. 2014, 156, 137–143. DOI: 10.1016/j.foodchem.2014.02.015.
- Oosten, B. J. Interactions between Starch and Electrolytes. Starch – Stärke. 1990, 42, 327–330. DOI:10.1002/(ISSN)1521-379X.
- Chiotelli, E.; Pilosio, G.; Le Meste, M. Effect of Sodium Chloride on the Gelatinization of Starch: A Multimeasurement Study. Biopolymers. 2002, 63, 41–58. DOI:10.1002/(ISSN)1097-0282.
- Cui, M.; Fang, L.; Zhou, H.; Yang, H. Effects of Amino Acids on the Physiochemical Properties of Potato Starch. Food Chem. 2014, 151, 162–167. DOI:10.1016/j.foodchem.2013.11.033.
- Lu, J.; Luo, Z.; Xiao, Z. Effect of Lysine and Glycine on Pasting and Rheological Properties of Maize Starch. Food Res. Int. 2012, 49, 612–617. DOI:10.1016/j.foodres.2012.06.038.
- Liang, X.; King, J. M. Pasting and Crystalline Property Differences of Commercial and Isolated Rice Starch with Added Amino Acids. J. Food Sci. 2003, 68, 832–838. doi:10.1111/jfds.2003.68.issue-3.
- Cai, J.; Yang, Y.; Man, J.; Huang, J. Wang, Z.; Zhang, C.; Wei, C. Structural and Functional Properties of Alkali-Treated High-Amylose Rice Starch. Food Chem. 2014, 145, 245–253. doi: 10.1016/j.foodchem.2013.08.059.
- Lai, L. N.; Karim, A. A.; Norziah, M. H.; Seow, C. C. Effects of Na2CO3 and NaOH on DSC Thermal Profiles of Selected Native Cereal Starches. Food Chem. 2002, 78, 355–362. doi:10.1016/S0308-8146(02)00097-3.
- Nor Nadiha, M. Z.; Fazilah, A.; Bhat, R.; Karim, A. A. Comparative Susceptibilities of Sago, Potato and Corn Starches to Alkali Treatment. Food Chem. 2010, 121, 1053–1059. doi:10.1016/j.foodchem.2010.01.048.
- Whistler, R. L.; Daniel, J. R. Starch; John Wiley & Sons, Inc, Hoboken, New Jersey, 2000.
- Kong, X.; Kasapis, S.; Bertoft, E.; Corke, H. Rheological Properties of Starches from Grain Amaranth and Their Relationship to Starch Structure. Starch – Stärke 2010, 62, 302–308. doi:10.1002/star.v62:6.
- Huang, M.; Kennedy, J.; Li, B.; Xu, X.; Xie, B. Characters of Rice Starch Gel Modified by Gellan, Carrageenan, and Glucomannan: A Texture Profile Analysis Study. Carbohydr Polym. 2007, 69, 411–418. doi:10.1016/j.carbpol.2006.12.025.
- Krystyjan, M.; Ciesielski, W.; Khachatryan, G.; Sikora, M.; Tomasik, P. Structure, Rheological, Textural and Thermal Properties of Potato Starch – Inulin Gels. LWT Food Sci. Technol. 2014, 60, 131–136. doi:10.1016/j.lwt.2014.07.056.
- Achayuthakan, P.; Suphantharika, M. Pasting and Rheological Properties of Waxy Corn Starch as Affected by Guar Gum and Xanthan Gum. Carbohydr Polym. 2008, 71, 9–17. doi:10.1016/j.carbpol.2007.05.006.
- Komiya, T.; Nara, S. Changes in Crystallinity and Gelatinization Phenomena of Potato Starch by Acid Treatment. Starch‐Stärke 1986, 38, 9–13. doi:10.1002/(ISSN)1521-379X.
- Sanderson, G. R.;. Food Gels; Harris, P., Ed.; Springer: Netherlands, 1990; pp. 201–232.
- Olivato, J. B.; Grossmann, M. V. E.; Bilck, A. P.; Yamashita, F. Effect of Organic Acids as Additives on the Performance of Thermoplastic Starch/Polyester Blown Films. Carbohydr Polym. 2012, 90, 159–164. doi:10.1016/j.carbpol.2012.05.009.
- Majzoobi, M.; Beparva, P. Effects of Acetic Acid and Lactic Acid on Physicochemical Characteristics of Native and Cross-Linked Wheat Starches. Food Chem. 2014, 147, 312–317. doi:10.1016/j.foodchem.2013.09.148.
- Chen, W.; Zhou, H.; Yang, H.; Cui, M. Effects of Charge-Carrying Amino Acids on the Gelatinization and Retrogradation Properties of Potato Starch. Food Chem. 2015, 167, 180–184. doi:10.1016/j.foodchem.2014.06.089.
- Virtanen, T.; Autio, K.; Suortti, T.; Poutanen, K. Heat-Induced Changes in Native and Acid-Modified Oat Starch Pastes. J. Cereal Sci. 1993, 17, 137–145. doi:10.1006/jcrs.1993.1014.
- Kavanagh, G. M.; Ross-Murphy, S. B. Rheological Characterisation of Polymer Gels. Prog. Polym. Sci. 1998, 23, 533–562. doi:10.1016/S0079-6700(97)00047-6.
- Heyman, B.; Depypere, F.; Van Der Meeren, P.; Dewettinck, K. Processing of Waxy Starch/Xanthan Gum Mixtures within the Gelatinization Temperature Range. Carbohydr Polym. 2013, 96, 560–567. doi:10.1016/j.carbpol.2012.10.076.
- Chiotelli, E.; Le Meste, M. Effect of Small and Large Wheat Starch Granules on Thermomechanical Behavior of Starch. Cereal Chem. J. 2002, 79, 286–293. doi:10.1094/CCHEM.2002.79.2.286.
- Wang, L.; Wang, Y.-J. Structures and Physicochemical Properties of Acid‐Thinned Corn, Potato and Rice Starches. Starch‐Stärke 2001, 53, 570–576. doi:10.1002/1521-379X(200111)53:11<570::AID-STAR570>3.0.CO;2-S.
- Wang, Y.-J.; Truong, V.-D.; Wang, L. Structures and Rheological Properties of Corn Starch as Affected by Acid Hydrolysis. Carbohydr Polym. 2003, 52, 327–333. doi:10.1016/S0144-8617(02)00323-5.
- Hirashima, M.; Takahashi, R.; Nishinari, K. Effects of Adding Acids before and after Gelatinization on the Viscoelasticity of Cornstarch Pastes. Food Hydrocoll. 2005, 19, 909–914. doi:10.1016/j.foodhyd.2004.12.004.
- Hedayati, S.; Shahidi, F.; Koocheki, A.; Farahnaky, A.; Majzoobi, M. Physical Properties of Pregelatinized and Granular Cold Water Swelling Maize Starches at Different pH Values. Int. J. Biol. Macromol. 2016, 91, 730–735. doi:10.1016/j.ijbiomac.2016.06.020.
- Karim, A. A.; Nadiha, M. Z.; Chen, F. K.; Phuah, Y. P. Chui, Y. M.; Fazilah, A. Pasting and Retrogradation Properties of Alkali-Treated Sago (Metroxylon Sagu) Starch. Food Hydrocoll. 2008, 22, 1044–1053. doi: 10.1016/j.foodhyd.2007.05.011.
- Clark, A.; Ross-Murphy, S. Biopolymers; Springer:Berlin, Heidelberg, 1987; pp. 57–192.
- Xu, Y. X.; Kim, K. M.; Hanna, M. A.; Nag, D. Chitosan–Starch Composite Film: Preparation and Characterization. Ind Crops Prod. 2005, 21, 185–192. doi:10.1016/j.indcrop.2004.03.002.
- Alamri, M. S.; Mohamed, A. A.; Hussain, S. Effects of Alkaline-Soluble Okra Gum on Rheological and Thermal Properties of Systems with Wheat or Corn Starch. Food Hydrocoll. 2013, 30, 541–551. doi:10.1016/j.foodhyd.2012.07.003.
- Hirashima, M.; Takahashi, R.; Nishinari, K. Effects of Citric Acid on the Viscoelasticity of Cornstarch Pastes. J. Agric. Food Chem. 2004, 52, 2929–2933. doi:10.1021/jf0347867.
- Wang, B.; Wang, L.-J.; Li, D.; Özkan, N.;, et al. Rheological Properties of Waxy Maize Starch and Xanthan Gum Mixtures in the Presence of Sucrose. Carbohydr Polym. 2009, 77, 472–481. doi: 10.1016/j.carbpol.2009.01.017.
- Pongsawatmanit, R.; Temsiripong, T.; Ikeda, S.; Nishinari, K. Influence of Tamarind Seed Xyloglucan on Rheological Properties and Thermal Stability of Tapioca Starch. J. Food Eng. 2006, 77, 41–50. doi:10.1016/j.jfoodeng.2005.06.017.
- Song, K. W.; Kim, Y. S.; Chang, G. S. Rheology of Concentrated Xanthan Gum Solutions: Steady Shear Flow Behavior. Fibers. Polym. 2006, 7, 129–138. doi:10.1007/BF02908257.