ABSTRACT
In order to broaden the use of chicken fat (CF), a new shortening was developed using stearin fractions of CF as a raw material. The CF was mixed with different base oils, and the compatibility, plasticity, and polymorphism of the mixture were analyzed. The influence of emulsifiers on the water-absorbing and air-absorbing abilities, and rheology of the CF-based shortening was studied. The mixtures of CF with palm oil at a mass ratio of 7:3 and CF with beef tallow at a mass ratio of 5:5 exhibited good compatibility, plasticity for baking, and crystal structure. By analysis of water-absorbing and air-absorbing abilities, the optimal amount of emulsifiers in both mixtures was determined as 1%, and the ratios of distilled monostearate, SPAN 60, and lecithin were determined to be 0.45:0.45:0.1 and 0.45:0.1:0.45 in the mixtures of CF with palm oil and CF with beef tallow, respectively. Different emulsifiers had significant effects on the rheological properties of CF-based shortenings. In addition, mixed emulsifiers had greater effects on the water-absorbing and air-absorbing abilities of the shortening than single emulsifier.
Abbreviations: BT: Beef tallow; CF: Chicken fat; DMS: Distilled monostearate; DSC: Differential scanning calorimeter; HPO: Hydrogenated palm oil; PO: Palm oil; POO: Palm oil olein; POS: Palm oil-based shortenings; PS: Palm stearin; SFC: Solid fat content; SMP: Slip melting point; XRD: X-ray diffraction.
Introduction
Besides the United States, China is the world’s second largest broiler producer. The rapid development of broiler industry has produced a lot of by-products from chicken processing, such as fat, bone, blood, and feathers. Centralized production of chicken also made the comprehensive utilization of by-products feasible.[Citation1]
At present, research on the processing of chicken fat (CF) in food industry is mainly focused on effects of different preparation methods on fat quality,[Citation1,Citation2] and research on the use of CF is focused on the preparation of chicken flavor seasoning powder.[Citation3,Citation4] However, to our knowledge, there is little research on utilization of CF as compared with common fats, such as margarin, shortening, and so on. CF must be modified to achieve diversification of its usage. Main methods of oils and fats modification include fractionation, transesterification, hydrogenation, and mixing.[Citation5] These modifications may lay the foundation for the development of baking shortening with CF.
Functional properties of shortening for baking, such as plasticity, water-absorbing, and air-absorbing abilities, are dependent on three elements: crystal structure of β′ form, solid fat content (SFC), and plasticity range. Solid fat in shortening is the most important factor as it may affect crystal formation and crystal type.[Citation6] The polymorphism of oils and fats mainly includes α form, β′ form and β form. When oils and fats with a certain SFC solidify, the crystal form is mainly determined by triglycerides with the highest melting point. When shortening stabilizes at β′ form, it presents a needle like morphology, has good air-absorbing ability and spreading capacity, and is suitable for making breads, biscuits, and other baked foods. When shortening stabilizes at β form, it presents a needle like morphology and has larger grains, reduced gas holding, and air-absorbing capacity. In addition, in order to make the shortening suitable for baking, different base oils were frequently used for mixing and modifying; and the compatibility of base oils directly influences the processing, quality, and shelf life of shortening and other special oil products. Problems such as sandiness, recrystallization, and reduced air-absorbing capacity occurred when mixing oils with poor compatibility.[Citation7] There are many research reports on application of emulsifiers in shortening and margarine. Emulsifiers could significantly delay transformation from β′ form to β form.[Citation8,Citation9]
The objective of this study was thus to use CF as the material for the development of CF-based shortening and to determine its functional properties, including compatibility, plasticity, crystal form, water-absorbing and air-absorbing abilities, and rheological properties. Furthermore, the study was aimed to optimize the base oils and emulsifier formulations for potential use in baking. This study may provide a way for the comprehensive utilization of CF and provide a way to evaluate a method for shortening product that can be used for baking.
Materials and methods
Materials
CF and beef tallow (BT) were purchased from a local supermarket. Palm oil (PO), palm oil olein (POO), palm stearin (PS), hydrogenated palm oil, palm oil-based shortening (POS, food grade), distilled monostearate [food grade, HLB = 3.8 (C16:0 65%, C18:0 35%)], lecithin [food grade, Hydrophilic lypophilic balance (HLB) = 7.5 (25% Phosphocholine (PC), 15% Phosphatidylinositol (PI), 20% Phosphoethanolamine (PE), 10% Phosphatidic acid (PA)], SPAN 60 (food grade, HLB = 4.7 (C16:0 50.92%, C18:0 41.50%, C18:1 1.96%)] were provided by Kerry Special Fats Ltd. (Shanghai, China). Supelco 37 component FAME mixture was purchased from Sigma-Aldrich (Shanghai, China). All other reagents and solvents were of analytical or chromatographic grade.
Preparation of CF shortening
Fresh CF was boiled and filtered to obtain raw CF for the experiment. The raw CF was heated to 80°C and kept for 30 min, and then immersed in 60°C water for 15 min. The treated sample was cooled to 24°C at a cooling speed of 2°C/min in a cold water bath. After holding for 16 h at 24°C, solid fat A and liquid oil B were obtained by separation with a refrigerated centrifuge (5000 r/min, 15 min, and 24°C). The liquid oil B was heated to 80°C and kept for 30 min, and then immersed in 60°C water for 15 min. The treated sample was cooled to 20°C at a cooling speed of 0.5°C/min in a cyclic cold water bath. After holding for 32 h at 20°C, solid fat C and liquid oil D were obtained by separation with a refrigerated centrifuge (5000 r/min, 15 min, and 20°C). Solid fat A and solid fat C were mixed and used as CF in the experiment.
The CF and other base oils were melted and mixed according to the ratios shown in , and then an emulsifier was added, mixed, melted, and ready for use. Preparation of CF shortening was as follows: the mixed oil was heated to 80°C and kept for 30 min after 1% emulsifier was added and mixed. Then the mixed oil was precooled 20 min at room temperature, and the mixed oil was stirred to crystallize at 20°C sharp quenching temperature and the mixed oil cooled to 18°C. The mixed oil was stirred and kneaded for 5 min at room temperature, and then was kept at 25°C to for 3 days. The samples were then stored at 20°C until analysis.
Table 1. Formula of CF-based shortenings.
Slip melting point measurements
The Slip melting point (SMP) was determined according to the method described in AOCS Cc 3-25. Briefly, the fat filled to 1 cm high in an open capillary tube was pre-solidified by touching over a piece of ice and then chilled at 10 ± 1°C for 16 h. Three capillaries were attached gently to a thermometer using a rubber band before being immersed into a beaker with cold distilled water. The water was stirred and heated and the temperature was recorded when the fat column began to rise in the tubes. Duplicate measurements were made.
Measurement of fatty acid composition
Fatty acid methyl esters (FAME) were prepared according to the AOCS Official Method Ce 2-66 and subsequently analyzed with a GC-14B gas chromatograph (GC) equipped with a flame ionization detector (Shimadzu, Tokyo, Japan) and a fused-silica capillary column (DB-5MS 60 m × 0.25 mm × 0.5 μm; Agilent Technologies). The temperature of the injection port and detector were all set at 250°C. The column was heated to 120°C and held for 3 min, then increased to 175°C at a rate of 8°C/min and held for another 28 min, and increased to 215°C at 3°C/min and held for 20 min. The fatty acid species was identified using the retention time of a FAME standard solution.
Measurement of SFC
The SFC was determined according to the AOCS Official Method Cd 16b-93. The SFC of the samples was determined on a NMI 20 pulsed nuclear magnetic resonance spectrometer (Niumag, Shanghai, China). The sample and standard were placed in the NMR tube and melted by heating to 80°C for 30 min and cooled to 60°C for 15 min, then kept at the desired temperature (10°C, 21.1°C, 26.7°C, 33.3°C, and 40°C) for 60 min respectively before measurement. Duplicate measurements were made.
Compatibility and plasticity
The compatibility of the two kinds of fat was evaluated with dual isothermal curve and ΔSFC. In the dual isothermal curve, the content of different base oils in the mixed fat was used as the horizontal coordinate, and the measured SFC was used as the vertical coordinate. ΔSFC is the difference between measured SFC and theoretical SFC of the mixed fat.
in which the theoretical SFC was calculated as follows:
where x and y represent respectively the volume fractions of components X and Y in the mixed fat; SFCX and SFCY represent respectively the measured SFC of components X and Y at a certain temperature. The deviation curve was drawn using temperature as horizontal coordinate and ΔSFC as vertical coordinate. The ideal ΔSFC value was zero, that is, the measured SFC value was equal to the theoretical SFC value. When ΔSFC was positive, the monotectic phenomenon occurred; on the contrary, the eutectic phenomenon occurred. When ΔSFC was closer to zero, the compatibility of the mixed fat was better.[Citation10]
The plasticity of fats could be typically reflected by SFC. Normally, a broad plasticity range was required for bakery shortening. The plasticity of shortening helped air incorporation and increased the volume during the creaming stage in cake batter and cookie dough formation. The typical ranges of SFC for commercial bakery shortening were 23–40% at 10°C, 16–30% at 21.1°C, 13–27% at 26.7°C, 9–21% at 33.3°C, and 3–11% at 40°C.[Citation11,Citation12]
Analysis of crystal polymorphism by X-ray diffraction
The polymorphic forms of fat crystals in the mixture were determined by a Dmax2200PC X-ray diffractor (Rigaku, Tokyo, Japan), using Cu–Ka radiation with a Ni filter (λ = 1.54056 Å, voltage 40 kV, current 30 mA, the divergence, anti-scatter and receiving slits were fixed at 1.0, 1.0, and 0.1 mm, respectively). The scanning range was 3–30°, and the scan rate was set at 2°/min. The samples were analyzed at ambient temperature, and duplicate analysis was carried out.
Analysis method of XRD[Citation13,Citation14]: Based on the short spacing characteristic peaks of the XRD pattern, the XRD was used to analyze the crystal structures of fats. The judgment criteria of shortening polymorph were characteristic peaks appearing near 4.15 Å for α form, near 4.20 and 3.80 Å for β ′ form, and near 4.60 Å for β form.
Melting-crystallizing curves of differential scanning calorimetry
The thermal properties of the samples were determined using a Q20 DSC (TA, USA). Samples were hermetically sealed in an aluminum pan. The samples were cooled to −40°C and held for 10 min, and then the melting profiles were obtained by heating to 80°C at 5°C/min. An empty pan served as a reference. DSC analysis method[Citation13,Citation14] was used to analyze crystal forms according to the characteristic endothermic peaks of sample’s melting curves. The analysis criteria of polymorphism in melting behavior were as follows: low characteristic peaks appearing at 0–10°C indicated α or β ′ form, significant spike characteristic peak at high-temperature range indicated β form, and irregular overlapping peaks indicated polymorphism between α and β form.
Measurements of water-absorbing and air-absorbing abilities
Measurement of water-absorbing ability: 20 g of base oils were weighed and 1% of emulsifier was added, then the mixture was heated and melted. At 25–30°C, 60 mL of distilled water was added slowly into the system, with stirring at the rate of 1000 r/min. After addition of water, the mixture was stirred at 2000 r/min for 2 min, and thin oil–water emulsion was formed. The mixture was placed at room temperature (20–25°C) for 12 h, and the volume of water leached from the system was measured. The amount of water absorbed by the system was calculated as the difference of the total amount of water added and the amount of water leached.[Citation9]
Measurement of air-absorbing ability: 20 g of base oils were weighed and 1% of emulsifier was added, then the mixture was heated and melted. The mixture was placed at 25–30°C for 4 h. Also, 30 g of syrup were added, and the mixture was stirred at 100 r/min for 1 min, then stirred at 500 r/min for 2 min, and finally stirred at 1000 r/min for 5 min. The mixture was made into fine and white paste by stirring, and its proportion was calculated. The amount of proportion decreased after stirring was used as the evaluation index of air-absorbing ability.[Citation9]
Twenty grams of shortenings were weighed respectively, and 1% of distilled monoglyceride (DMS), lecithin, and SPAN 60 were added, respectively, and their water-absorbing and air-absorbing abilities were measured, respectively. PO-based shortening was used as a control. With water-absorbing and air-absorbing abilities as indicators, a mixture regression design was performed to determine the optimal ratio. Because the mixing of ternary materials was studied and the content of each emulsifier could not be zero, this was a problem of mixture regression design with lower bound constraint. The content of each emulsifier was set not less than 10%, the ternary system was indicated with A–B–C, and their contents were expressed as XA, XB, and XC. So, XA ≥ 0.1, XB ≥ 0.1, XC ≥ 0.1, XA + XB + XC + 1. After conversion, XA = 0.7·ZA + 0.1, XB = 0.7·ZB + 0.1, XC = 0.7·ZC + 0.1. The formula of the emulsifiers in the ternary system is shown in .
Table 2. Mixture regression design of distilled monostearate, SPAN 60, and lecithin.
Rheology analysis
Samples were melted at 50°C for 2 h and transferred to AR1500ex rheometer with a 20 mm plate. The changes of viscosity and shearing stress of the samples were measured with the change of shear rate.[Citation15] The program was set as follows: sample temperature was 30°C, pre-sheared at a rate of 5 s−1 for 30 min, increased to 50 s−1, and then decreased to 5 s−1. This process took 20 min, and the changes of viscosity and shearing stress were obtained with the change of shear rate. The rheological parameters including yield, apparent viscosity, and thixotropy were determined using the methods of the International Confectionery Association.[Citation16] The yield was determined at a shear rate of 5 s−1. The viscosity at a shear rate of 30 s−1 was set as apparent viscosity. The thixotropy was set as the difference of yield at a shear rate of 5 s−1 during processes of speeding up and slowing down of shear rate.
Statistical analysis
Results are expressed as mean ± standard deviation. Data were analyzed with one-way ANOVA, followed by Duncan’s multiple-range tests using SPSS Statistics 17.0 (SPSS, Inc., Chicago, IL, USA). Experiments were repeated three times. Significance was evaluated at ± 5% (P ≤ 0.05).
Results and discussion
Fatty acid composition and SMP
As shown in , the level of total saturated fatty acids in CF was lower than the amount of total saturated fatty acids in the five shortening base oils. In addition to POO, the amount of total unsaturated fatty acids in CF was higher than that in the other oils. The contents of oleic acid (C18:1) and palmitic acid (C16:0) were the highest in CF, followed by linoleic acid (C18:2) and stearic acid (C18:0). The value of SMP was higher when the content of saturated fatty acid in fats was higher. However, the contents of saturated fatty acids and unsaturated fatty acids in CF were close to that of palm olein, but the difference of their SMP points was approximately 20°C. This was because the SMP of fats was often determined by the saturated fatty acid triglyceride, which has the highest melting point in fats, and in the fractionation process of fats triglyceride generally crystallized as seed crystal for fats crystallization. Therefore, CF contained more triglyceride and POO contained less triglyceride, resulting in the difference between their melting points.
Table 3. Fatty acid compositions (%) and SMP (ºC) of raw oils and fats.
SFC, compatibility, and plasticity of base oils
Both CF-based shortenings of different formulations and commercial PO-based shortening had a high SFC and wide range of plasticity suitable for commercial shortenings. The level of solid fats content and wide plasticity range in CF-based shortening was suitable for making plastic fats of different functional requirements, making products that may meet different functional requirements.
It was very effective and quick to use ΔSFC-T to determine the compatibility of oils and fats. The theoretical SFC value of each CF-based shortening was calculated according to , and provides the measured SFC value of each CF shortening. For fully compatible binary mixture, its ΔSFC was less than ±1.5%. The six formulas provided by pre-experiment had good compatibility and conformed to the requirements of SFC and plasticity range of shortenings. As shown in , although slight monotectic and eutectic crystallization phenomena occurred, base oils of different formulas had good compatibility as a whole, which met the general requirements for commercial shortening.
Table 4. The SFC (%) of measured SFC and deviation value of the shortenings at different temperatures.
Figure 1. SFC-T profiles of chicken fat (CF), palm oil (PO), palm oil olein (POO), palm stearin (PS), and beef tallow (BT).
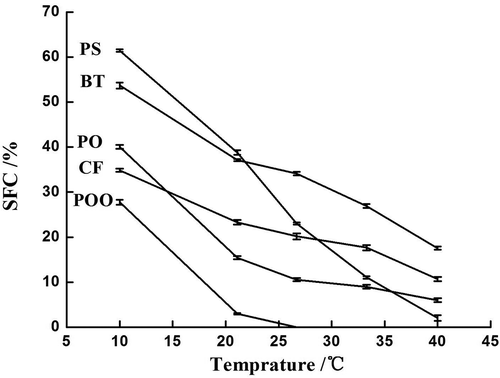
As shown in , the SFC is bigger in blends CF + POo1 and CF + POo2 then in CF + PO1 and CF + PO2 at same temperatures. The main reasons for this question are twofold: first, the mixing ratio of each oil is different. CF + POO1, CF, and POO mixed by a mass ratio of 8:2. CF + POO2, CF, and POO mixed by a mass ratio of 7:3. CF + PO1, CF, and PO mixed by a mass ratio of 7:3. CF + PO2, CF, and PO mixed by a mass ratio of 6:4. Secondly, CF + POO2 and CF + PO1 are compared with each other in the same proportion. CF + POO2 shows a certain degree of monotectic phenomenon at 10°C, 21.1°C, and 26.7°C, and a certain degree of eutectic phenomenon at 33.3°C and 40°C; CF + PO1 shows a certain degree of eutectic phenomenon at 10°C, 21.1°C, 26.7°C, 33.3°C, and 40°C.
Crystal forms and melting behavior of shortenings
At present, XRD and DSC are the best methods for studying polymorphism of fats at normal temperature and crystal form evolution of fats during heating process. As shown in , the CF-based shortenings and commercial PO-based shortening were mixtures of various crystal forms. As can be seen from the XRD patterns, CF + PO1 and CF + BT showed significant β′ crystal peaks, and characteristic peaks of other crystal forms were not obvious. CF + POO2, CF + PS, and CF + PO2 showed mainly β′ crystal peaks, but also showed some β crystal peaks. CF + POO1 and CF showed both β′ and β crystal peaks, and two kinds of peaks were both obvious.
Figure 2. XRD profiles (A) and DSC melting (B) of CF + POO1, CF + POO2, CF + PS, CF + PO1, CF + PO2, CF + BT, and POS sample.
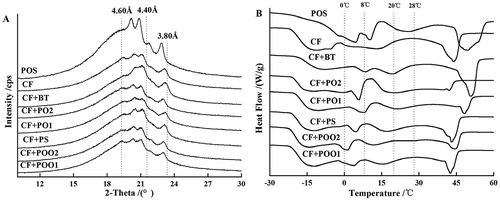
DSC melting curves showed the polymorphism of oils and fats during melting process. There were many times of crystal form transformation during melting process of solid fats. Each crystal form transformation or recrystallization may change the relatively unstable state to a more stable state. The characteristic peaks of DSC melting curves of various shortenings are shown in . A study in PO[Citation17] showed that the crystal corresponding to endothermic peaks at 0–8°C was β′ crystal of three times chain length, the crystals corresponding to endothermic peaks at 8–20°C were partly β′ crystal of three times chain length and partly β′ crystal of two-times chain length, the crystal corresponding to endothermic peaks at 20–28°C was β′ crystal of two times chain length, and the crystal corresponding to endothermic peaks at above 28°C was β crystal of two times chain length. Our study only discussed the crystal form of the shortenings without analyzing the chain length of the shortening. The XRD short spacing and DSC characteristic endothermic peaks of the same crystal forms were observed at the same time, which could be verification and supplement to sample’s crystal composition for each other. The crystal forms of various shortenings were different at room temperature, but they would transform into β crystal as the temperature increased, and finally the fats melted completely into liquids. In the practical utilization of shortening products in bakery foods, they only needed to exhibit their water-absorbing ability, air-absorbing capacity, and other functions at room temperature. So, as shown in , by comprehensive analysis of XRD and DSC patterns, CF + PO1 and CF + BT were found to be the best products in the seven CF-based shortenings.
Table 5. XRD and DSC pattern parameters of various shortenings.
Effects of emulsifiers on water-absorbing and air-absorbing abilities of shortenings
Effects of single emulsifier on water-absorbing and air-absorbing abilities of shortenings: Water-absorbing ability of shortenings reflected their ability to absorb and retain moisture in bakery foods. Good water-absorbing ability could effectively prevent food hardening and make the foods crisp. As shown in , although different emulsifiers had slightly different effects on the water-absorbing ability of these two base oils, the trend of effects of various emulsifiers on water-absorbing ability of two base oils was the same (DMS > SPAN 60 > lecithin), and the shortening added with DMS and PO-based shortening had similar water-absorbing abilities.
Air-absorbing ability of shortenings reflected their ability of parceling air when shortenings and batter were mixed with high-speed whipping. Good air-absorbing ability was especially important to products such as cakes so that for baked goods with fluffy and soft taste, a stronger air-absorbing ability was better.[Citation18] As shown in , the results of air-absorbing ability were similar to those of water-absorbing ability. The effects of different emulsifiers on air-absorbing ability of the two base oils were the same (DMS > SPAN 60 > lecithin). However, lecithin had a more significant influence on the air-absorbing ability of samples.
Effects of a mixture of emulsifiers on water-absorbing and air-absorbing abilities of shortenings: The single emulsifier had only limited improving effects and different emulsifiers may have synergetic effects, so a mixture of emulsifiers was commonly used in practical application.[Citation19–Citation21] According to the mixture regression design, the emulsifiers were mixed, and the added amount of the emulsifiers was 1%. As shown in , the water-absorbing abilities of emulsifiers with different ratios of two base oils were all approximately 20 mL/20 g. However, the air-absorbing abilities exhibited significant differences with changes of ratio and oil phase of emulsifiers. So when the water-absorbing and air-absorbing abilities of the shortenings were considered at the same time, Formula 4 was optimal for CF + PO1, and Formula 5 was optimal for CF + BT.
Table 6. Water-absorbing and air-absorbing abilities of different mixed emulsifiers.
Effects of emulsifiers on rheological parameters of shortenings
Yield values indicated the stress level needed to be overcome for flow of shortenings when exerted with a certain energy. Higher yield values meant the shortenings were tougher and stronger. As shown in , the control had the highest yield value, indicating that the system had very high viscosity and high energy was needed to make the shortening to flow. Addition of 1.0% lecithin or a mixture of emulsifiers significantly reduced the yield value of the system. SPAN 60 and DMS had weaker effects on the decrease of yield values of shortenings. This was because SPAN 60 and DMS in oil systems tended to be crystalline improvement agent and affected the crystal growth of base oils and fats, but had insignificant effects on improvement of interfacial tension between dispersed phase and oil phase.[Citation22]
Table 7. Rheological parameters of the shortenings added with different emulsifiers.
The apparent viscosity of shortenings was expressed as the viscosity value at a shear rate of 30 s−1. Apparent viscosity could reflect to some extent the microstructure of the system. A lower apparent viscosity indicated that the dispersed phase of the system existed in the form of small aggregates. As shown in , the apparent viscosity of the control was the highest, followed by samples added with 1.0% of DMS and 1.0% of SPAN 60, and then samples with 1.0% emulsifiers mixture, and the apparent viscosity of samples added with 1.0% of lecithin was the lowest, which was consistent with a previous report.[Citation23]
Thixotropy indicated a fluid’s flow capability changed by contact such as stirring, which could reflect the aggregation degree of particles in the system.[Citation24] As shown in , the results of thixotropy were consistent with those of yield value and apparent viscosity. The thixotropy of the control was far higher than other samples, and the particles in the system had a strong tendency to aggregate. Addition of lecithin significantly reduced the thixotropy of the system, which was because lecithin molecules had both hydrophilic phospholipid acyl group and hydrophobic fatty acyl group, and could significantly improve the surface tension of interface. The thixotropy of samples added with 1% SPAN 60 was higher than that of lecithin, but was significantly lower than that of DMS. The sample added with DMS had a strong trend of particles aggregation, and the effect of a mixture of emulsifiers on thixotropy was close to that of DMS.
In summary, types of emulsifiers had significant effects on the rheological properties of CF shortening. Lecithin significantly improved the rheological properties of the system. SPAN 60 had small effects on the viscosity and yield value of the system, but significantly reduced the thixotropy. The system with 1.0% DMS had a strong tendency of particle aggregation. The mixture of emulsifiers did not significantly affect the rheological properties of shortenings, but had significant effects on water-absorbing and air-absorbing abilities of shortenings.
Conclusion
The study developed a CF-based shortening. On the basis of this study on the melting points and the composition and content of fatty acids of stearin fractions of CF and four kinds of shortening base oils, the compatibility of CF with four kinds of base oils was analyzed and seven kinds of shortening formulation with suitable plasticity range for special bakery shortening were confirmed. CF and palm oil (PO) at a mass ratio of 7:3 (formulation 4) and CF and beef tallow (BT) at a mass ratio of 5:5 (formulation 6) showed different β’ crystal state which was more larger than that of the β crystal. And they were two best formulations in the seven kinds of formulations. Emulsifiers had important effects on the state and crystal stability of shortenings. DMS, lecithin, and SPAN 60 were chosen as emulsifiers for CF-based shortenings. The effects of emulsifiers on the water-absorbing and air-absorbing abilities and rheological properties of shortenings were studied. Types of emulsifiers had significant effects on the rheological properties of CF-based shortenings, and a mixture of emulsifiers had significant effects on the water-absorbing and air-absorbing abilities of shortenings.
Additional information
Funding
References
- Lasekan, A.; Bakar, F. A.; Hashim, D. Potential of Chicken By-Products as Sources of Useful Biological Resources. Waste Manag. 2013, 33, 552–565.
- Arnaud, E.; Trystram, G.; Relkin, P.; Collignan, A. Thermal Characterization of Chicken Fat Dry Fractionation Process. J. Food Eng. 2006, 72, 390–397.
- Liu, J.; Liu, M.; He, C.; Song, H.; Chen, F. Effect of Thermal Treatment on the Flavor Generation from Maillard Reaction of Xylose and Chicken Peptide. LWT Food Sci. Technol. 2015, 64, 316–325.
- Song, S.; Yuan, L.; Zhang, X.; Hayat, K.; Chen, H.; Liu, F.; Xiao, Z.; Niu, Y. Rapid Measuring and Modelling Flavour Quality Changes of Oxidised Chicken Fat by Electronic Nose Profiles through the Partial Least Squares Regression Analysis. Food Chem 2013, 141, 4278–4288.
- Kontkanen, H.; Rokka, S.; Kemppinen, A.; Miettinen, H.; Hellström, J.; Kruus, K.; Marnila, P.; Alatossava, T.; Korhonen, H. Enzymatic and Physical Modification of Milk Fat: A Review. Int. Dairy J. 2011, 21, 3–13.
- Oliveira, G. M. D.; Ribeiro, A. P. B.; Santos, A. O. D.; Cardoso, L. P.; Kieckbusch, T. G. Hard Fats as Additives in Palm Oil and Its Relationships to Crystallization Process and Polymorphism. LWT Food Sci. Technol. 2015, 63, 1163–1170.
- Zhou, S. L.; Zhang, F. Q.; Jin, Q. Z.; Liu, Y. F.; Shan, L.; Zhang, T.; Zhou, X. Q.; Wang, X. T. Characterization of Palm Kernel Oil, Palm Stearin, and Palm Olein Blends in Isosolid Diagrams. Eur. J. Lipid Sci. Technol. 2010, 112 (9), 1041–1047.
- Lai, Y. L.; Chin, N. L.; Lim, C. H.; Yusof, Y. A.; Talib, R. A. Saturated Distilled Monoglycerides Variants in Gel-Form Cake Emulsifiers. Agric. Agric. Sci. Procedia 2014, 2, 191–198.
- Hernquist, L.; Anjou, K. Diglycerides as a Stabilizer of the ß’-Crystal Form in Margarines and Fats. Eur. J. Lipid Sci. Technol. 2010, 85, 64–66.
- Shen, Z.; Birkett, A.; Augustin, M. A.; Dungey, S.; Versteeg, C. Melting Behavior of Blends of Milk Fat with Hydrogenated Coconut and Cottonseed Oils. J. Am. Oil Chem. Soc. 2001, 78, 387–394.
- List, G. R.; Warner, K.; Pintauro, P.; Gil, M. Low-Trans Shortening and Spread Fats Produced by Electrochemical Hydrogenation. J. Am. Oil Chem. Soc. 2007, 84, 497–501.
- Criado, M.; Hernández-Martín, E.; López-Hernández, A.; Otero, C. Enzymatic Interesterification of Extra Virgin Olive Oil with a Fully Hydrogenated Fat: Characterization of the Reaction and Its Products. J. Am. Oil Chem. Soc. 2007, 84, 717–726.
- Meng, Z.; Liu, Y. F.; Jin, Q. Z.; Huang, J. H.; Song, Z. H.; Wang, F. Y.; Wang, X. Comparative Analysis of Lipid Composition and Thermal, Polymorphic, and Crystallization Behaviors of Granular Crystals Formed in Beef Tallow and Palm Oil. J. Agric. Food Chem. 2011, 59 (4), 1432.
- Wang, F.; Liu, Y.; Shan, L.; Jin, Q.; Meng, Z.; Wang, X. Effect of Fat Composition on Texture and Bloom of Lauric Compound Chocolate. Eur. J. Lipid Sci. Technol. 2010, 112, 1270–1276.
- Emmanuelohene, A.; Alistair, P.; Mark, F.; Joselio, V. Comparison of Rheological Models for Determining Dark Chocolate Viscosity. Int. J. Food Sci. Technol. 2010, 44, 162–167.
- Emmanuelohene, A.; Alistair, P.; Mark, F. Effects of Particle Size Distribution and Composition on Rheological Properties of Dark Chocolate. Eur. Food Res. Technol. 2008, 226, 1259–1268.
- Chong, C. L.; Kamarudin, Z.; Lesieur, P.; Marangoni, A.; Bourgaux, C.; Ollivon, M. Thermal and Structural Behaviour of Crude Palm Oil: Crystallisation at Very Slow Cooling Rate. Eur. J. Lipid Sci. Technol. 2010, 109, 410–421.
- Munk, M. B.; Marangoni, A. G.; Ludvigsen, H. K.; Norn, V.; Knudsen, J. C.; Risbo, J.; Ipsen, R.; Andersen, M. L. Stability of Whippable Oil-In-Water Emulsions: Effect of Monoglycerides on Crystallization of Palm Kernel Oil. Food Res. Int. 2013, 54 (2), 1738–1745.
- Radujko, I.; Jurić, J.; Pajin, B.; Omorjan, R.; Šereš, Z.; Simović, D. Š. The Influence of Combined Emulsifier 2 in 1 on Physical and Crystallization Characteristics of Edible Fats. Eur. Food Res. Technol. 2011, 232, 899–904.
- Schantz, B.; Rohm, H. Influence of Lecithin-PGPR Blends on the Rheological Properties of Chocolate. LWT Food Sci. Technol. 2005, 38, 41–45.
- Garti, N.; Schlichter, J.; Sarig, S. Effect of Food Emulsifiers on Polymorphic Transitions of Cocoa Butter. J. Am. Oil Chem. Soc. 1986, 63, 230–236.
- Smith, K. W.; Bhaggan, K.; Talbot, G.;, et al. Crystallization of Fats: Influence of Minor Components and Additives. J. Am. Oil Chem. Soc. 2011, 88, 1085–1101.
- Nebesny, E.; Zyzelewicz, D. Effect of Lecithin Concentration on Properties of Sucrose-Free Chocolate Masses Sweetened with Isomalt. Eur. Food Res. Technol. 2005, 220, 131–135.
- Do, T. A.; Hargreaves, J. M.; Wolf, B.; Hort, J.; Mitchell, J. R. Impact of Particle Size Distribution on Rheological and Textural Properties of Chocolate Models with Reduced Fat Content. J. Food Sci. 2007, 72, E541–E552.