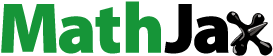
ABSTRACT
This study was conducted to investigate the potential of cryogenic freezing with liquid nitrogen in the shelf-life extension of northern snakehead (Channa argus) and clarify the effects of temperature fluctuations after freezing on the quality attributes and tissue microstructure during frozen storage. The fish fillets were frozen by three methods including freezing using an ultra-low-temperature freezer (−80°C) to the core temperature of −60°C (T1) or −18°C (T2), or liquid nitrogen (T3) followed by storage at −20°C for five months. Cryogenic freezing with liquid nitrogen postponed the decrease in pH and protein extractability. Temperature fluctuations after freezing might promote the accretion of ice crystals and resulted in the loss of tissue integrity and disorganization of myofibrils. The microstructural changes contributed greatly to the increased thawing loss and decreased resilience, as indicated by the enlarged extracellular spacing and the flakiness of myofibrils. Cryogenic freezing with liquid nitrogen showed no superiority in maintaining the microstructure of northern snakehead fillets, which was supposedly attributed to the cracking in tissue during freezing and the accretion of ice crystals during frozen storage.
Introduction
Northern snakehead (Channa argus) is one of the most important economic fish species cultured in China. In 2012, the total production of snakehead reached 480,594 tonnes.[Citation1] This fish has a tremendous market value due to the high quality of flesh, low fat content, and few intramuscular spines as an important protein source, which is also well known for its recuperative and medicinal qualities.[Citation2,Citation3] Generally, northern snakehead, which has high vitality, is sold whole and alive, killed, and cooked in a variety of ways.[Citation4] However, as an invasive species, its introduction can pose a great threat to the native fish populations. Therefore, it is urgent to find out alternative methods to meet the demands of global trade due to its high nutritive and medicinal value. It is well known that freezing plays a critical role in prolonging the shelf life and ensuring the safety of meat and meat products, especially the easily perishable fish. Unfortunately, no related information has been available on northern snakehead fish. Therefore, it is necessary to verify the suitability of frozen storage in the preservation of northern snakehead meat.
As the composition and structure of fish meat are complicated, changes in the quality of frozen meat are related to many factors, such as changes in the pH, protein properties, and microstructure of muscle tissue.[Citation5–Citation7] Tissue integrity is of great importance to the maintenance of muscle quality, especially for the water-holding capacity and textural properties.[Citation8] The formation and accretion of ice crystals during freezing and frozen storage may disrupt organelles and cells and then some proteinases and pro-oxidants are released, resulting in the deterioration of muscle quality.[Citation9] These changes can be influenced by various factors, including freezing rate, storage temperature, storage time, and so on.[Citation10–Citation12] Among those, freezing rate is thought to be critical to the size and distribution of ice crystals, which have great effects on the quality of frozen products.[Citation13] Generally, high freezing rate is preferred, which is assumed to reduce the size of ice crystals formed and maintain good quality of frozen products.[Citation14] In recent years, cryogenic freezing with liquid nitrogen has attracted great attention because it is non-toxic, inert, ensures high freezing rate at very low temperature, and maintains good properties of frozen products.[Citation15] It is supposed that cryogenic freezing with liquid nitrogen might be a potentially promising technique in seafood preservation, which can facilitate the formation of small ice crystals and reduce the quality deterioration on freezing with a high heat transfer coefficient.[Citation16]
Based on the above, the potential of cryogenic freezing with liquid nitrogen in the preservation of northern snakehead meat was investigated compared with fast freezing at −80°C. Moreover, different final freezing temperatures were used to clarify the effects of temperature fluctuations after freezing on the quality attributes and microstructure of northern snakehead meat. The pH, extractability of salt-soluble proteins, activities of α-glucosidase (AG) and β-N-acetyl-glucosaminidase (NAG), water-holding capacity, and textural properties of northern snakehead fillets were evaluated. The microstructural changes were observed by a light microscope (LM) and a scanning electron microscope (SEM) during frozen storage.
Materials and methods
Sample preparation
Northern snakehead fish with a mean weight of 0.6–0.7 kg were purchased from a local aquatic market in Hangzhou, China. Living fish were killed by a blow to the head, beheaded, gutted, and transported to the laboratory in polystyrene boxes on ice within 20 min. Each fish was washed with ice water to remove any remaining blood, then filleted into 2-cm-thick slices manually perpendicular to the vertebral column, and randomly separated into three groups before freezing. The first group was frozen at −80°C by an ultra-low-temperature freezer (MDF-382E (N), Sanyo Electric Co., Ltd., Japan) to the core temperature of −60°C (T1). The second group was frozen under the same conditions to the core temperature of −18°C (T2). The third group was frozen by immersion in liquid nitrogen (T3). The core temperatures of the samples were monitored by a wireless temperature sensor (Data Trace RF, Mesa Laboratories, Inc., Germany). Then the samples were wrapped by a food wrap film made from polyethylene and stored at −20°C for up to five months. Three fish were taken randomly from one group at each sampling time and thawed at 4°C for 10 h before analysis. Fresh specimens were considered as 0 day of storage for comparison with the frozen ones. For every sampled fish, the dorsal part from the same position was used for the same parameter measurement to eliminate the effect of different positions. The fish slices from near the head to the tail were used for the water-holding capacity measurement, microscopic observation, textural analysis, and biochemical attributes assays. For the analysis of biochemical properties, the fish meat was minced after thawing before analyses.
Methods
pH
In this study, 10 g of minced fish after thawing was dispersed in 100 ml of cold distilled water and stirred for 30 min. The pH was then measured using a digital pH meter (PHS-3BW, Bante Instruments, Ltd., Shanghai, China).
Salt-soluble protein content
Salt-soluble proteins were extracted according to a slightly modified method described by Careche, Del Mazo, Torrejón, and Tejada.[Citation17] For 2.00 g of minced meat, 20 ml of cold phosphate buffer (I = 0.05, pH 7.5) was added and stirred, followed by centrifuging at 3000 rpm for 10 min at 4°C using a high-speed refrigerated centrifuge (3 k-15, sigma Co., Ltd., Germany). The precipitate was washed twice more with the same buffer and centrifuged to remove the water-soluble proteins. To the collected pellet, 20 ml of 0.6 M KCl was subsequently added and the mixture was homogenized at 12000 rpm for 1 min. The homogenate was stirred at 4°C for 4 h, followed by centrifuging at 8500 rpm for 30 min at 4°C. The supernatant was collected and made up to 50 ml with 0.6 M KCl. The protein content was determined by the Biuret method,[Citation18] and the salt-soluble protein content was expressed as the percentage of salt-soluble proteins extracted from fish meat.
Activities of AG and NAG
The centrifugal exudate was prepared according to a slightly modified method described by Duun and Rustad.[Citation19] Minced meat was centrifuged at 15000 rpm for 40 min at 4°C, and the exudate was collected and made up to 25 ml with distilled water before conducting an enzyme assay. The protein content in the exudate was determined by the Biuret method. The activities of AG and NAG were determined by the method described by Sriket, Benjakul, Visessanguan, and Kijroongrojana.[Citation20] For the AG activity assay, the reaction mixture contained 0.6 ml of 0.1 M sodium citrate buffer (pH 4.0), 0.4 ml of 1.0 M NaCl, and 2 ml of diluted muscle exudate. The reaction mixture was pre-incubated at 37°C for 10 min before 2 ml of 4.2 mM ρ-nitrophenyl-α-glucopyranoside was added. After 60 min, the reaction was terminated by 2 ml of 0.3 M KOH. The absorbance was measured at 405 nm. A negative control was carried out by adding 0.3 M KOH prior to the addition of ρ-nitrophenyl-α-glucopyranoside. To determine the activity of NAG, 0.3 ml of 0.1 M sodium citrate buffer (pH 4.5) and 0.2 ml of 0.6 M KCl were added to 0.2 ml of diluted muscle exudate. The reaction was initiated by 0.2 ml of ρ-nitrophenyl-N-acetyl-β-D-glucose amide, and then the mixture was incubated at 37°C for 10 min. The reaction was stopped by 1 ml of 0.3 M KOH. The amount of ρ-nitrophenol released was monitored at 405 nm and calculated using a molar extinction coefficient of 19500 M−1 cm−1. One enzyme unit was defined as the activity that released 1 nmol of ρ-nitrophenol per minute.
Thawing loss
Blocks of samples (1 × 1 × 0.5 cm3 in size) were cut with a precooling blade and thawed at room temperature. The specimens were weighed before (Wa) and after thawing (Wb). The thawing loss was calculated according to Eq. (1):
Pressing loss
The pressing loss was measured by the pressing method with filter paper according to Benjakul, Visessanguan, Thongkaew, and Tanaka,[Citation21] with some modifications. The thawed specimens were placed between filter papers, with three sheets at the bottom and on the top, respectively, and then held under pressure (10 kg) for 10 min. The samples were removed from the paper and weighed (Wc). The pressing loss was calculated by the weight difference before and after pressing according to Eq. (2):
Total loss
For the fresh samples, thawing loss was not determined. As such, the pressing loss was regarded as the total loss. The total loss of frozen northern snakehead fillets was obtained according to Eq. (3):
Instrumental textural properties
Textural properties of the northern snakehead fillets were determined by a Texture Analyzer (TA-XT2i, Stable Micro Systems Co., Ltd., UK). The thawed samples that were cut into small pieces (1 × 1 × 0.5 cm3 in size) were placed at room temperature (25°C) for 1 h to eliminate the effect of low temperature prior to textural analysis. Each sample was compressed twice perpendicular to the muscle fibre orientation with a flat-ended cylindrical plunger (P/5) at a constant test speed of 1 mm/s and the deformation was 60%. The textural attributes were obtained using textural profile analysis-macro software attached to the instrument.
LM observation
The samples were prepared according to Liu, Liang, Xia, Regenstein, and Zhou[Citation22] with slight modifications. After thawing, six blocks (approximately 3 × 3 × 6 mm3 in size) were cut from the back of each sample and then immersed in Carnoy’s solution (60% v/v absolute ethanol, 30% v/v chloroform, and 10% v/v glacial acetic acid) at 4°C for 24 h. The fixed samples were dehydrated using a graded series of ethanol solutions, followed by substitution with xylene. The specimens were embedded in paraffin, and the cross sections (8 µm in thickness) were achieved with a microtome (YD202A, Yidi Medical Appliance Co., Ltd., Zhejiang, China). Consecutively, the sections were reshaped in water, mounted on glass slides, dried for 24 h on a dryer (YD-AB, Yidi Medical Appliance Co., Ltd, Zhejiang, China), de-waxed, stained with hematoxylin and eosin solution, and then observed and photographed using an optical microscope (Model UB200i, UOP Photoelectric Technology Co., Ltd., Chongqing, China).
SEM observation
The samples (5 × 5 × 2 mm3) cut from the back were fixed in 2.5% glutaraldehyde (0.1 M phosphate buffer, pH 7.2) for 24 h at 4°C. All the specimens were washed twice with 0.1 M phosphate buffer for 15 min. The specimens were then post-fixed with 1% osmic acid solution for 1 h, and the washing process was repeated. After dehydration in a graded series of ethanol (from 50%, 70%, 90% to 100%, v/v) and critical point drying, the samples were coated with gold palladium and viewed using a SEM (XL30, Philips Co., Ltd., Nederland).
Statistical analysis
Data were subjected to a variance analysis (ANOVA) with OriginPro 8.5. Means comparison was performed using the Tukey method with a statistical significance of p < 0.05.
Results and discussion
Temperature changes of northern snakehead fillets
Changes in the core temperature of the samples were recorded until the temperatures maintained stable at −20°C (). The core temperature of the liquid nitrogen freezing samples reached −73°C after 20.5 min, whereas the core temperatures of those frozen at −80°C reached −18°C and −60°C after 24 min and 80 min, respectively. The time through the zone of maximum ice crystal formation was about 7 min and 2 min for T1 and T2 groups and T3 group, respectively. The freezing rates of these methods were very high, which were considered to lead to the formation of large amounts of small ice crystals. Small ice crystals, which cause less mechanical damage to frozen food, are preferred to the large ones.[Citation16] When the samples were transferred to −20°C, the temperatures of T1 and T3 groups increased initially because of the temperature differences between the samples and the storage ambient. The temperature fluctuation after freezing might affect the stability of ice crystals formed during freezing and consequently the quality changes of frozen meat.[Citation23]
Changes in pH and content of salt-soluble proteins of northern snakehead meat during frozen storage
Effects of freezing method on the pH of northern snakehead fillets during frozen storage are shown in ). The pH differed significantly with the increasing storage period (p < 0.05). The values decreased initially and then increased except for the T2 group. In this study, the processing time before freezing was less than 1 h, so the fish were considered to be in the state of pre-rigor. From the results, the pH of the T3 group, which was not different from that of the fresh meat, was significantly higher after 14 days of storage compared to the other methods. It was conceived that freezing with liquid nitrogen postponed the onset of rigor mortis. The initial reduction in pH is thought to be caused by the lactic acid formed by glycolytic reaction.[Citation24] Alkaline substances produced by protein degradation may increase pH due to endogenous enzymes released from the tissue with prolonged storage time.[Citation25]
Figure 2. Changes in pH and content of salt-soluble proteins of northern snakehead frozen by different methods during frozen storage.
T1 (frozen at −80°C to −60°C), T2 (frozen at −80°C to −18°C), and T3 (frozen by liquid nitrogen).Each value is expressed as mean±standard deviation (n = 3).
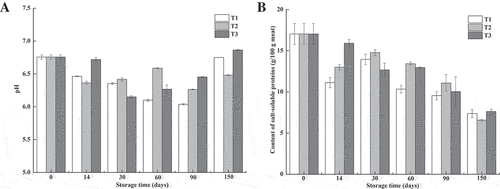
It is believed that the salt-soluble proteins are responsible for the functional properties of meat and meat products. A decrease in protein extractability of meat is indicative of quality deterioration. After 14 days of storage, the protein extractability of T3 group was not significantly different from that of the fresh ones. However, a significant decrease in the salt-soluble protein contents of T1 and T2 groups were observed (p < 0.05), which might be related to the lower pH in these samples.[Citation26] After 30 days of storage, the content of salt-soluble proteins decreased continuously with the extended storage period. No significant differences were found among the different freezing methods after 90 and 150 days of storage (p > 0.05). Compared to the fresh samples, there was a 52% decrease in the protein extractability after 150 days of frozen storage. Badii et al.[Citation10] reported that the protein solubility of cod and haddock muscles decreased with the increasing storage time despite the different storage temperatures. The decreased protein extractability might be caused by the aggregation and denaturation of proteins during freezing and frozen storage. After freezing, the formation of ice crystals causes the concentration of cellular solutes, which might accelerate protein denaturation. The water layers are thought to be of crucial importance for the structure of proteins.[Citation27] With the dehydration of proteins, some side-chain residues are exposed, and the aggregation and crossing-links of proteins might be formed easily by the formation of disulphide, hydrogen, and hydrophobic bonds, resulting in the decrease in protein extractability.[Citation28–Citation30] Moreover, the released proteinases from the organelles might accelerate the protein denaturation during frozen storage. Because myofibrillar proteins are of great importance in the nutritional and functional qualities of fish meat, denaturation of myofibrillar proteins, as indicated by the decrease in the extractability of salt-soluble proteins, might exert an adverse effect on the quality of northern snakehead fillets.
Changes in activities of AG and NAG of northern snakehead meat during frozen storage
The activities of AG and NAG have been reported as markers of cell or tissue integrity.[Citation31] As shown in , the activities of AG and NAG increased with the extended storage time, followed by a significant decrease in both the activities after 150 days of frozen storage (p < 0.05). With the increasing storage time, the accretion of ice crystals might cause mechanical damage to the myofibres, resulting in the release of enzymes. The decline in the enzymatic activity in the late storage period was presumed to be due to the denaturation of AG and NAG. These results were in accordance with those of Benjakul et al.,[Citation21] who found that the AG and NAG activities of croaker and lizardfish decreased after 20 and 16 weeks of frozen storage at −18°C, respectively. These enzymatic changes in this study indicated a significant loss of cell membrane integrity of northern snakehead during freezing and frozen storage. A significant increase in the activities of AG and NAG was observed in the T3 group after 60 days of storage (p < 0.05), whereas it was observed after 90 days in the T1 and T2 groups. Although a high freezing rate was obtained with the liquid nitrogen freezing method, the cracks after freezing and the accretion of ice crystals during frozen storage might be responsible for the deterioration of tissue integrity. It is reported that small ice crystals presumably melt faster than the larger ones and form larger crystals with a slight increase in temperature, even if it is still very low.[Citation28] In this study, large amounts of small ice crystals that formed after freezing in the T3 group might have melted, formed larger ice crystals, and caused mechanical damages to the meat when the samples were transferred to −20°C, which might be responsible for the loss of tissue integrity.
Changes in water-holding capacity of northern snakehead meat during frozen storage
One of the most important features of meat quality is its water-holding capacity, which is closely related to the juiciness, texture, and other quality properties of meat.[Citation32] Loss of water-holding capacity is not only unappealing to consumers but also causes an economic loss for enterprises. Changes in the water-holding capacity of northern snakehead fillets frozen by different methods during frozen storage are presented in in terms of thawing loss, pressing loss, and total loss. The thawing loss increased significantly with the samples of T1 and T2 groups after 90 days of frozen storage (p < 0.05), whereas the samples of T3 group kept having the highest values from 30 days of storage. These changes in thawing loss were closely related to the tissue integrity and structural changes.
Figure 4. Changes in the water-holding capacity of northern snakehead frozen by different methods during frozen storage.
T1 (frozen at −80°C to −60°C), T2 (frozen at −80°C to −18°C), and T3 (frozen by liquid nitrogen).Each value is expressed as mean±standard deviation (n = 6).
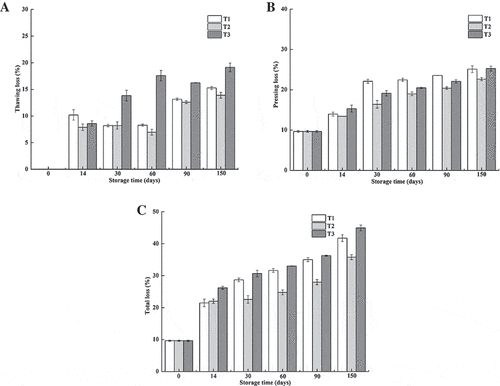
A continual increase in pressing loss was observed in all the samples with increasing storage time, with the samples of the T1 group being the highest from 30 days of storage. Freezing method had a significant effect on the water-holding capacity of frozen fish meat. Throughout the storage period, the minimum values of pressing loss and total loss were obtained in the T2 group. The thawing loss, pressing loss, and total loss were negatively correlated with the protein extractability with the correlation coefficients of −0.80, −0.84, and −0.87, respectively. Moreover, the loss of water-holding capacity of frozen northern snakehead meat was also closely related to the disruption of tissue integrity.
Changes in textural characteristics of northern snakehead meat during frozen storage
Changes in the textural characteristics of northern snakehead fillets during frozen storage are shown in . From the texture profile analysis, different parameters of fish texture were obtained. Hardness is defined as the maximum resistance during the first compression, whereas springiness is the height recovered after the first compression. Cohesiveness provides a relative and dimensionless measure of the muscle strength that remained after the first compression.[Citation33] In this study, the hardness showed no significant changes within 30 days of storage (p > 0.05), and then decreased gradually with extended storage time, especially after 150 days of storage. The decrease in hardness with a long storage period might be caused by the loss of tissue integrity and the enzymes released during frozen storage.[Citation34] The springiness changed little throughout the frozen storage. No significant difference was observed with the cohesiveness of the fillets (data not shown). As a result, chewiness, which is the product of hardness, springiness, and cohesiveness, decreased gradually with the extended storage time. The chewiness was affected by the freezing method significantly. It decreased significantly in the T1 and T3 groups after 60 days of storage (p < 0.05), whereas it showed less changes in the T2 group until 90 days of storage. Resilience is calculated by the area of the first upstroke divided by the area of the first downstroke and reflects the force and speed with which the fillets bounce back after the first compression.[Citation33] As a parameter of meat elasticity, the resilience of northern snakehead was mostly affected by freezing instead of storage time. Changes in the textural properties during frozen storage were postulated to be affected by many factors, such as the protein solubility, modifications of myofibrillar proteins due to the protease action, and decrease in water-holding capacity.[Citation35,Citation36] The textural deterioration of northern snakehead during frozen storage in this study was coincidental with the reduction in the extractability of salt-soluble proteins and water-holding capacity. The structural changes of the tissue and myofibrils also supposedly played an important role in the textural changes.
Effect of freezing method on the microstructure of northern snakehead fillets during frozen storage
To clarify the effects of freezing method and frozen storage on the microstructure of northern snakehead fillets, the LM and SEM profiles were observed. From the LM profiles, the myofibres were in a typical polygonal shape and were tightly attached to one another for the fresh samples. The cell boundaries were quite distinct, and no detachments between the cells were found ( and 0 d). After 30 days of storage, the cells of the T2 group still maintained a good shape, whereas small detachments were found in the T1 and T3 groups, and the cells showed deformities, especially in the T3 group ( and 30 d). With prolonged storage time, the myofibres were more seriously damaged and deformed in the T3 group. Larger extracellular spacing observed in the T3 group after 30 days and 60 days of storage might be responsible for the higher thawing loss than the other two groups. However, more severe intracellular damages were observed in the T1 group, as indicated by more cavities inside the cells. It might be related to the protein denaturation. During thawing, the ice crystals in the muscle melted and more white holes were left when the “recovery ability” of the cells decreased, which mainly depended on the protein properties.[Citation23] The high pressing loss in the T1 group might be caused by these structural damages. Disorganization was found after 90 days of storage including in the T2 group. No difference in muscular disintegration could be found among different freezing methods after 150 days of storage, which was so severe that the structure of the myofibres could not be recognized totally. This was in accordance with the results observed in the tilapia muscle after six months of storage at −20°C.[Citation37]
Figure 6. Changes in LM (A) and SEM (B) profiles of northern snakehead frozen by different methods during frozen storage.
T1 (frozen at −80°C to −60°C), T2 (frozen at −80°C to −18°C), and T3 (frozen by liquid nitrogen).Magnification of LM profiles is 100 × .
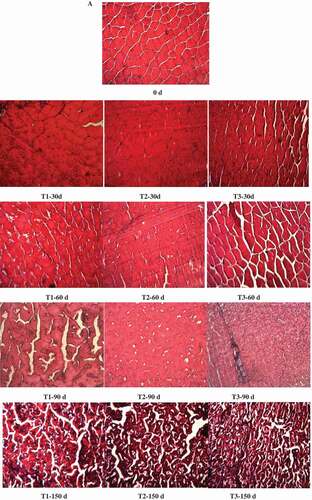
Theoretically, large amounts of nuclei will be formed, limiting the size of ice crystals in a system subjected to high freezing rates. However, ultra-rapid freezing (freezing in liquid nitrogen in this study) might cause mechanical cracking,[Citation38] which might accelerate the microstructural deterioration and quality loss of the northern snakehead fillets. Moreover, small ice crystals tend to melt and form large ice crystals when suffering from temperature fluctuations.[Citation28] Because of the larger temperature shift in the samples under T1 and T3 conditions, recrystallization and redistribution of ice crystals might take place more severely in the northern snakehead fillets when the samples were transferred to −20°C for storage. Therefore, not only the freezing method, but also the management of storage temperature is of crucial importance for the quality of fish meat.
More detailed information on the intracellular changes of northern snakehead was obtained from the SEM profiles ()). The myofibrils arranged neatly and straightforwards with clear boundaries in the fresh samples. After 30 days of storage, some ruptures were observed in the T1 group and disorder in the T3 group, while regular shape was retained in the T2 group. However, the flakiness of myofibrils was observed in all the samples after frozen storage, which might be caused by the dehydration of proteins. It was much more clearly observed after 60 days of storage, at which time the disorganized myofibrils were first found in the T2 group. After 90 days and 150 days of storage, the myofibrils were in disorder in all the samples with holes and gaps irrespective of the freezing method. The changes in the myofibrils observed could partly be responsible for changes in the textural properties and water-holding capacity, especially the resilience of the northern snakehead.
Conclusions
Effects of freezing method and frozen storage on the quality attributes and tissue microstructure of northern snakehead were investigated in this study. Cryogenic freezing with liquid nitrogen could postpone the decrease of the pH and salt-soluble protein content in meat, whereas it showed no superiority in maintaining the tissue integrity. The deterioration of tissue structure, which resulted in the decrease of water-holding capacity and textural properties, might be caused by the cracking during freezing and the accretion of ice crystals due to temperature fluctuation after freezing. It is indicated that avoiding temperature fluctuation is of crucial importance for meat quality.
Additional information
Funding
References
- Bureau of Fisheries of the Ministry of Agriculture. China Fishery Statistical Yearbook, China Agriculture Press: Beijing, 2013.
- Lu, F.; Liu, D. H.; Ye, X. Q.; Wei, Y. X.; Liu, F. Alginate–Calcium Coating Incorporating Nisin and EDTA Maintains the Quality of Fresh Northern Snakehead (Channa argus) Fillets Stored at 4°C. Journal of the Science of Food and Agriculture 2009, 89(5), 848–854. DOI: 10.1002/jsfa.v89:5.
- Lu, F.; Ding, Y. T.; Ye, X. Q.; Liu, D. H. Cinnamon and Nisin in Alginate–Calcium Coating Maintain Quality of Fresh Northern Snakehead Fish Fillets. LWT-Food Science and Technology 2010, 43(9), 1331–1335. DOI: 10.1016/j.lwt.2010.05.003.
- Marimuthu, K.; Thilaga, M.; Kathiresan, S.; Xavier, R.; Mas, R. H. M. H. Effect of Different Cooking Methods on Proximate and Mineral Composition of Striped Snakehead Fish (Channa striatus, Bloch). Journal of Food Science and Technology 2012, 49(3), 373–377. DOI: 10.1007/s13197-011-0418-9.
- Saeed, S.; Howell, N. K. Effect of Lipid Oxidation and Frozen Storage on Muscle Proteins of Atlantic Mackerel (Scomber scombrus). Journal of the Science of Food and Agriculture 2002, 82(5), 579–586. DOI: 10.1002/(ISSN)1097-0010.
- Leelapongwattana, K.; Benjakul, S.; Visessanguan, W.; Howell, N. K. Physicochemical and Biochemical Changes during Frozen Storage of Minced Flesh of Lizardfish (Saurida micropectoralis). Food Chemistry 2005, 90(1–2), 141–150. DOI: 10.1016/j.foodchem.2004.03.038.
- Muela, E.; Sañudo, C.; Campo, M. M.; Medel, I.; Beltran, J. A. Effect of Freezing Method and Frozen Storage Duration on Lamb Sensory Quality. Meat Science 2012, 90(1), 209–215. DOI: 10.1016/j.meatsci.2011.07.003.
- Benjakul, S.; Bauer, F. Biochemical and Physicochemical Changes in Catfish (Silurus glanis Linne) Muscle as Influenced by Different Freeze–Thaw Cycles. Food Chemistry 2001, 72(2), 207–217. DOI: 10.1016/S0308-8146(00)00222-3.
- Alizadeh, E.; Chapleau, N.; De Lamballerie, M.; Le-Bail, A. Effect of Different Freezing Processes on the Microstructure of Atlantic Salmon (Salmo salar) Fillets. Innovative Food Science & Emerging Technologies 2007, 8(4), 493–499. DOI: 10.1016/j.ifset.2006.12.003.
- Badii, F.; Howell, N. K. Changes in the Texture and Structure of Cod and Haddock Fillets during Frozen Storage. Food Hydrocolloids 2002, 16(4), 313–319. DOI: 10.1016/S0268-005X(01)00104-7.
- Sánchez-Alonso, I.; Moreno, P.; Careche, M. Low Field Nuclear Magnetic Resonance (LF-NMR) Relaxometry in Hake (Merluccius merluccius, L.) Muscle after Different Freezing and Storage Conditions. Food Chemistry 2014, 153(15), 250–257. DOI: 10.1016/j.foodchem.2013.12.060.
- Sánchez-Valencia, J.; Sánchez-Alonso, I.; Martinez, I.; Careche, M. Estimation of Frozen Storage Time or Temperature by Kinetic Modeling of the Kramer Shear Resistance and Water Holding Capacity (WHC) of Hake (Merluccius merluccius, L.) Muscle. Journal of Food Engineering 2014, 120, 37–43. DOI: 10.1016/j.jfoodeng.2013.07.012.
- Martino, M. N.; Otero, L.; Sanz, P. D.; Zaritzky, N. E. Size and Location of Ice Crystals in Pork Frozen by High-Pressure-Assisted Freezing as Compared to Classical Methods. Meat Science 1998, 50(3), A303–313. DOI: 10.1016/S0309-1740(98)00038-2.
- Rodezno, L. A. E.; Sundararajan, S.; Solval, K. M.; Chotiko, A.; Li, J.; Zhang, J.; Alfaro, L.; Bankston, J. D.; Sathivel, S. Cryogenic and Air Blast Freezing Techniques and Their Effect on the Quality of Catfish Fillets. LWT-Food Science and Technology 2013, 54(2), 377–382. DOI: 10.1016/j.lwt.2013.07.005.
- Gökoğlu, N.; Yerlikaya, P. Seafood Chilling, Refrigeration and Freezing-Science and Technology, John Wiley & Sons, Inc.: Chichester, UK, 2015, pp. 174-181.
- Sanz, P. D.; De Elvira, C.; Martino, M.; Zaritzky, N.; Otero, L.; Carrasco, J. A. Freezing Rate Simulation as an Aid to Reducing Crystallization Damage in Foods. Meat Science 1999, 52(3), 275–278. DOI: 10.1016/S0309-1740(99)00002-9.
- Careche, M.; Del Mazo, M. L.; Torrejón, P.; Tejada, M. Importance of Frozen Storage Temperature in the Type of Aggregation of Myofibrillar Proteins in Cod (Gadus morhua) Fillets. Journal of Agricultural and Food Chemistry 1998, 46(4), 1539–1546. DOI: 10.1021/jf970841y.
- Gornall, A. G.; Bardawill, J. C.; David, M. M. Determination of Serum Proteins by Means of the Biuret Reaction. Journal of Biological Chemistry 1949, 177(2), 751–766.
- Duun, A. S.; Rustad, T. Quality of Superchilled Vacuum Packed Atlantic Salmon (Salmo salar) Fillets Stored at −1.4 And −3.6°C. Food Chemistry 2008, 106(1), 122–131. DOI: 10.1016/j.foodchem.2007.05.051.
- Sriket, P.; Benjakul, S.; Visessanguan, W.; Kijroongrojana, K. Comparative Studies on the Effect of the Freeze–Thawing Process on the Physicochemical Properties and Microstructures of Black Tiger Shrimp (Penaeus monodon) and White Shrimp (Penaeus vannamei) Muscle. Food Chemistry 2007, 104(1), 113–121. DOI: 10.1016/j.foodchem.2006.11.004.
- Benjakul, S.; Visessanguan, W.; Thongkaew, C.; Tanaka, M. Comparative Study on Physicochemical Changes of Muscle Proteins from Some Tropical Fish during Frozen Storage. Food Research International 2003, 36(8), 787–795. DOI: 10.1016/S0963-9969(03)00073-5.
- Liu, D. S.; Liang, L.; Xia, W. S.; Regenstein, J. M.; Zhou, P. Biochemical and Physical Changes of Grass Carp (Ctenopharyngodon idella) Fillets Stored at −3 and 0°C. Food Chemistry 2013, 140(1–2), 105–114. DOI: 10.1016/j.foodchem.2013.02.034.
- Fukuda, Y. Denaturation by Freezing of Fish Muscle Proteins. Bull. Natl. Res. Inst. Fish. Sci. 1996, 8, 77–79.
- Bahmani, Z. A.; Rezai, M.; Hosseini, S. V.; Regenstein, J. M.; Böhme, K.; Alishahi, A.; Yadollahi, F. Chilled Storage of Golden Gray Mullet (Liza aurata). LWT-Food Science and Technology 2011, 44(9), 1894–1900. DOI: 10.1016/j.lwt.2011.01.009.
- Subramanian, T. A.; Effect of Processing on Bacterial Population of Cuttle Fish and Crab and Determination of Bacterial Spoilage and Rancidity Development on Frozen Storage. Journal of Food Processing and Preservation 2007, 31(1), 13–31. DOI: 10.1111/j.1745-4549.2007.00101.x.
- Bito, M.; Effect of pH on Water-Holding Properties of Frozen Skipjack Meat. Bulletin Japan Society of Science and Fisheries 1978, 44(2), 163–168. DOI: 10.2331/suisan.44.163.
- Puolanne, E.; Halonen, M. Theoretical Aspects of Water-Holding in Meat. Meat Science 2010, 86(1), 151–165. DOI: 10.1016/j.meatsci.2010.04.038.
- Soliman, Y. K. S.; Theories of Protein Denaturation during Frozen Storage of Fish Flesh. Advanced Food Research 1980, 26, 275–307.
- Jiang, S. T.; Hwang, D. C.; Chen, C. S. Effect of Storage Temperature on the Formation of Disulfide and Denaturation on Milkfish Actomyosin (Chanos chanos). Journal of Food Science 1988, 53(5), 1333–1335. DOI: 10.1111/j.1365-2621.1988.tb09270.x.
- Badii, F.; Howell, N. K. A Comparison of Biochemical Changes in Cod (Gadus morhua) and Haddock (Melanogrammus aeglefinus) Fillets during Frozen Storage. Journal of the Science of Food and Agriculture 2002, 82(1), 87–97. DOI: 10.1002/(ISSN)1097-0010.
- Alberio, G.; Barbagallo, R. N.; Todaro, A.; Bono, G.; Spagna, G. Effect of Freezing/Thawing Process in Different Sizes of Blue Fish in the Mediterranean through Lysosomal Enzymatic Tests. Food Chemistry 2014, 148, 47–53. DOI: 10.1016/j.foodchem.2013.10.013.
- Hamm, R.; Biochemistry of Meat Hydration. Advanced Food Research 1960, 10, 355–463.
- Hyldig, G.; Nielsen, D. A Review of Sensory and Instrumental Methods Used to Evaluate the Texture of Fish Muscle. Journal of Texture Studies 2001, 32(3), 219–242. DOI: 10.1111/jts.2001.32.issue-3.
- Leygonie, C.; Britz, T. J.; Hoffman, L. C. Impact of Freezing and Thawing on the Quality of Meat: Review. Meat Science 2012, 91(2), 93–98. DOI: 10.1016/j.meatsci.2012.01.013.
- Natseba, A.; Lwalinda, I.; Kakura, E.; Muyanja, C. K.; Muyonga, J. H. Effect of Pre-Freezing Icing Duration on Quality Changes in Frozen Nile Perch (Lates niloticus). Food Research International 2005, 38(4), 469–474. DOI: 10.1016/j.foodres.2004.10.014.
- Cai, L. Y.; Wu, X. S.; Dong, Z. J.; Li, X. P.; Yi, S. M.; Li, J. R. Physicochemical Responses and Quality Changes of Red Sea Bream (Pagrosomus major) to Gum Arabic Coating Enriched with Ergothioneine Treatment during Refrigerated Storage. Food Chemistry 2014, 160, 82–89. DOI: 10.1016/j.foodchem.2014.03.093.
- Chen, Y.; Pan, B. S. Morphological Changes in Tilapia Muscle following Freezing by Airblast and Liquid Nitrogen Methods. International Journal of Food Science and Technology 1997, 32(2), 159–168. DOI: 10.1046/j.1365-2621.1997.00392.x.
- Kalichevsky, M. T.; Knorr, D.; Lillford, P. J. Potential Food Applications of High-Pressure Effects on Ice-Water Transitions. Trends in Food Science and Technology 1995, 6(8), 253–258. DOI: 10.1016/S0924-2244(00)89109-8.