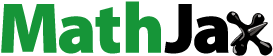
ABSTRACT
The aim of this study was to enhance aqueous solubility of xanthone with natural polymers using oil-in-water emulsion and complex coacervation as the microencapsulation techniques. There was no interaction between xanthone and the wall material; and xanthone crystallinity was decreased as observed via fourier transform infrared spectroscopy (FTIR), differential scanning calorimetry (DSC) and x-ray powder diffraction (XRD) after microencapsulation. Oil-in-water emulsion and coacervation increased xanthone solubility by 37-fold and 1.6-fold, respectively. However, O/W emulsion method required a high ratio of oil to xanthone. The solubilizing effect of oil and wall material, particle size reduction, and decreased crystallinity were responsible for enhancing the aqueous solubility of xanthone.
Introduction
Xanthones are polyphenolic heterocyclic compounds that can be naturally found in mangosteen rind or semi/fully synthesised.[Citation1] A substantial number of studies have demonstrated the compound’s antioxidant, antimicrobial, anti-inflammatory, anti-platelet, anti-malarial, and atherosclerotic activities.[Citation1,Citation2] Interest in the above-mentioned properties has led to its potential usage as a nutraceutical agent in food and pharmaceutical industries. However, poor aqueous solubility of xanthones limits its bioavailability and hence the full exploitation of its biological properties.[Citation3-Citation5] Solvent evaporation[Citation5] and solvent displacement[Citation3] that utilise synthetic polymers and organic solvents, such as methanol, have been attempted to improve the aqueous solubility of xanthone. These methods produce residual solvent which poses potential side effects. There is a need for an approach, which is simple, safe, solvent free, and effective in improving the aqueous solubility of xanthone. To date, only a few studies have been attempted such as spray drying of mangiferin with pectin or chitosan.[Citation6] Our previous studies show that xanthone can be spray dried with maltodextrin and gum Arabic[Citation4] or inulin[Citation7] in the presence of fish oil.
Hydrophobic compounds such as drugs incorporated into oil-in-water (O/W) emulsion have been shown to enhance aqueous solubility of poorly water-soluble compounds.[Citation8,Citation9] Although physical instability of emulsion is a disadvantage, this is not the case with dry emulsion. Dry emulsions are physically and microbiologically stable formulations and can be prepared by spray drying, rotary evaporation, and lyophilisation.[Citation8] Among these, spray drying is a widely used technique as it permits fast solvent evaporation, low cost, and continuous process.[Citation9] This technique successfully produced dry emulsion powder with increased aqueous solubility and in turn bioavailability of numerous hydrophobic compounds, including drugs, such as Lu 28–179[Citation10], 5-phenyl-1,2-dithiole-3-thione (5-PDTT)[Citation11], Carvedilol[Citation12], flaxseed oil[Citation13], d-limonene[Citation14], and curcumin.[Citation15] Complex coacervation is another common microencapsulation method, which involves spontaneous phase separation process forming an insoluble complex between two or more polymers as a result of electrostatic interactions.[Citation16] Microparticles produced by complex coacervation are heat resistant with controlled-release characteristics and have been shown to be a promising formulation for poorly water-soluble compounds.[Citation17,Citation18] Drying the coacervate microparticles either through freeze drying, oven drying and spray drying extends their shelf life and allows its usage in dehydrated products.[Citation16,Citation19,Citation20]
With increasing customers demand in natural products, polymers of natural source are favoured as wall material in this study. Inulin was selected as the wall material for the preparation of spray-dried O/W emulsion of xanthone. Inulin, a fructooligosaccharide (FOS), composed of fructose and oligomers units linked by β-(1,2) bonds with glucose at the end of fructose chains is naturally present in many food sources such as dandelion root and chicory root.[Citation21] Inulin is a substitute for sugar or fat with low caloric value. It has been used as an encapsulating agent for oregano oil[Citation22] and gallic acid.[Citation21] For O/W emulsion, castor oil, medium-chain triglycerides, liquid paraffin, and vegetable oils are commonly used in O/W emulsion. In the current study, canola oil contains low saturated fat and high in monounsaturated fat of omega-3 (alpha-linoleic acid) and omega-6 acids (linoleic acid) as well as the high amount of bioactive compounds such as antioxidants, polyphenols, tocopherols and phytosterols[Citation23], making it an attractive oil component for this study. For complex coacervation, xanthone was encapsulated by gelatin and gum Arabic, which are commonly used wall materials due to their biocompatibility and degradability. Gelatin is a water soluble protein obtained from partial hydrolysis of mammalian collagen.[Citation24] Gum Arabic is a complex heteroglycan consisting of a main chain of d-galactopyranose units joined by β-d-glycosidic bonds.[Citation24] Scientific literature has reported complex coacervation of β-glucuronidase[Citation20], drug such as indomethacin[Citation18], bioactive compounds such as lycopene[Citation19] and oil utilizing gelatin A and gum Arabic as the wall material. The aim of this study was to compare the aqueous solubility of xanthone using spray dried oil-in-water emulsion or oven dried complex coacervation techniques and to understand the underlying mechanism for improved aqueous solubility. Physicochemical properties of the microparticles were characterized.
Materials and methods
Materials
Frutafit® IQ is a commercial native inulin with ≥ 90% purity of dry matter basis and DP of 8–13 was provided by SENSUS International (Kuala Lumpur, Malaysia). Xanthone of 97% purity, gum Arabic and gelatin from porcine skin (type A) and tween 80 were purchased from Sigma-Aldrich (Missouri, USA) and canola oil (Naturel, Lam Soon Edible Oils Sdn. Bhd., Shah Alam Malaysia) from the local supermarket. All the other solvents were of analytical grade.
Preparation of spray-dried O/W emulsion
In our preliminary studies, it was found that the HLB value of 15.0 shows optimum O/W emulsion stability. Oil-in-water (O/W) emulsion was prepared by dissolving frutafit IQ in 70°C aqueous solution of 1% (v/v) tween 80 as the surfactant and xanthone in 70°C canola oil. The core of 1:30 (xanthone: canola oil) (w/w) ratio was homogenised into the aqueous phase containing frutafit IQ and surfactant at 1:4 (core: frutafit IQ) (w/w) ratio at 15,000 rpm for 2 min with Ultra-Turrax homogenizer (T25 Basic IKA LabortechNK, Königswinter, Germany) to form O/W emulsion of 20% total solid content and Hydrophile-Lipophile Balance (HLB) value of 15.0. In 100 mL solution, the amount of xanthone would be 0.13 gm. These conditions were selected based on preliminary studies (data not shown). The emulsion was then spray dried in a laboratory spray dryer (SD1500, Labquip, Petaling Jaya, Malaysia). Inlet and outlet temperature, blow set and feed flow rate were kept constant at 200°C, 90°C, 50 Hz and 600 mL/h, respectively. The obtained powders were stored in amber bottles in a desiccator until further analysis.
Preparation of oven-dried complex coacervates
Two percent (w/v) of type A gelatin and gum Arabic, each were prepared using 2 gm of gelatin or gum Arabic in 100 mL of distilled water at 45°C. One mL of 10% Tween 80 solution was added slowly into xanthone (0.5 g) as a wetting agent to form xanthone suspension. Gelatin and gum Arabic solution were mixed together and the xanthone suspension was added into the mixture. pH of the mixture was then reduced to 4.0 using 0.5 N or 0.1 N hydrochloric acids (HCl) to induce complex coacervation. The mixture was stirred at 300 rpm and allowed to cool slowly (0.27°C min−1) to room temperature and was further cooled to 15°C using an external ice bath with a thermometer and hot water and ice alternatively. The mixture was kept agitated at 150 rpm for 6 h at 15°C to harden the coacervates. After 6 h, the coacervate-rich layer was recovered by centrifugation at 1,500 rpm for 7 min. The supernatant was washed with 50% w/w isopropyl alcohol to remove untrapped xanthone and to dehydrate the coacervates. The isopropyl alcohol was removed from the sample by centrifuging the mixture at 1500 rpm for 2 min. This was repeated by a second wash with 75% w/w isopropyl alcohol. The sample was dried at 50°C for 6 h. The dried coacervates were collected in amber bottles stored in a desiccator with silica until further analysis.
Powder characterization
Standard curve of xanthone
High performance liquid chromatography (HPLC) (SD1500, Labquip, Petaling Jaya, Malaysia) analysis was conducted using a reversed phase HPLC column (Agilent ZORBAX Eclipse Plus C18; 250 × 4.6 mm, 5 μm) and a mobile phase comprising of 90% methanol in 0.1% formic acid at flow rate of 1 mL/min for 10 min. The standard curve of xanthone was constructed in the range of 5.0–40.0 μg/mL at 1 µL injection volume at 237 nm (y (Absorbance) = 11.009x (Concentration (μg/mL)); R2 = 0.9994).
Encapsulation yield (EY)
Encapsulation yield was determined according to Eq. (1)[Citation25]:
Loading capacity and encapsulation efficiency (EE)
Loading capacity also referred to as drug content or drug loading capacity in literature and encapsulation efficiency (EE) of the samples was calculated based on the reported method of Govender et al.[Citation26] with modification. Ten mg of powder was dissolved in 5 mL water at 37°C and shaken overnight. Then 5 mL of methanol was added. The solution was then filtered through a 0.45 μm filter before analysing by HPLC at 237 nm. Loading capacity was followed up to calculate EE and both were determined by the following equations:
Xanthone aqueous solubility
Aqueous solubility of the samples was evaluated as propsed by Dixit, Kini, and Kulkarni.[Citation27] An excess amount of samples (equivalent to 10 mg of xanthone) was added to 10 mL of water at 45°C and shaken overnight. The solution was centrifuged at 4000 g for 5 min. The supernatant was collected, filtered through 0.45 μm filter membrane, and diluted with methanol appropriately. The solution was then filtered through a 0.45 μm filter before analysing by HPLC at 237 nm. The aqueous solubility of xanthone was compared with a control, which was xanthone of 97% purity.
Particle size distribution analysis
Particle size distribution of the samples was measured by laser light diffraction technique using a Mastersizer (Malvern Mastersizer 3000, Malvern Instruments Ltd., UK). Refractive index (RI) of 1.642 for xanthone, 1.665 for O/W emulsion with isopropyl alcohol as a dispersion medium, and 1.33 for coacervates with water as the dispersion medium.
Moisture content
Moisture content of the samples was determined according to AOAC official method 934.06 by drying 2.0 g of powder samples in a vacuum oven (VD115, Binder, Tuttlingen, Germany) at 70°C for 24 h until a constant weight was obtained.[Citation28] The moisture content (wet basis, w.b) of the powder was calculated according to Eq. (4):
Where mb = mass of powder before drying, ma = mass of powder after drying.
Bulk Density
Bulk density of the samples was determined according to Goula and Adamopoulos[Citation29] with slight modifications. Two g of powder was transferred to a 50 mL graduated cylinder and held on a vortex vibrator for 2 min. Bulk density was calculated based on the ratio of mass over volume of powder contained in the cylinder (g mL−1).
Colour
Colour (CIE L*, a* and b* colour coordinates) of the samples was measured using a Colourflex spectrophotometer (45/0 CX2367, HunterLab, Virginia, US). L* denotes the lightness of the sample ranging from 0 (black) to 100 (white), a* indicates greenness (-) or redness (+), and b* represents yellowness (+) and blueness (-).
Optical microscopy
Samples were placed on a microscope slide covered with a glass cover slip. Microscopy images of the sample were obtained using an optical microscope (Olympus BX50, Tokyo, Japan) with 10x objective lens equipped with Image Pro Plus 4.0 software.
Field emission scanning electron microscope (FESEM)
Samples were secured in stubs using double-faced copper adhesive tape and coated with a thin layer of platinum using a Quorum (Q150RS) division FESEM sputter coating system (East Sussex, England) for 15 min before being observed using FESEM microscope (FE-SEM) (Hitachi SU8010, Tokyo, Japan) at various desired magnifications at accelerating voltage of 5–15 kV to determine powder morphology.
Fourier transform infrared spectroscopy (FTIR)
FTIR spectroscopy was performed to study the interaction between core and wall material. Samples and their infrared spectra were measured between 600 and 4000 cm−1 using a Varian 640-IR FTIR spectrophotometer (Varian, Corona, United States) to study the interaction between core and wall material.
X-ray diffraction (XRD)
XRD patterns of samples were obtained using X-Ray diffractometer (D8-Advance, Bruker-AXS, Massachusetts, USA). The analysis was performed with a Cobalt target X-ray tube operating at 40 kV and 40 mA. The instrumental settings were set at 1.540ºA wavelength, 2θ range of 2°–60° at a step size of 2°/min.
Differential scanning colorimetry (DSC)
Melting behaviour of the samples was measured using differential scanning calorimetry (Perkin Elmer, DSC 4000, Massachusetts, US). Sample (5 mg) was placed in a standard aluminium pan, crimped and a pin hole was poked into the lid. The sample was heated from −20 to 210°C, with a constant heating rate of 5°C/min under continuous purging of nitrogen (20 mL/min). An empty sealed aluminium pan was used as a reference.
Statistical analysis
All data were measured in triplicates (n = 9) and reported as mean ± standard deviation unless otherwise stated. All statistical analyses were carried out using Statistical Package for the Social Sciences (SPSS version 16, Chicago, United States) and analysed using one-way analysis of variance (ANOVA) with posthoc Tukey’s test.
Results and discussion
EY, loading capacity and EE
The O/W emulsion yield of 60.8 ± 6.2% () was comparable to other reports on spray-dried active compounds in O/W emulsion. Hansen et al.[Citation10] reported a yield of 54–66% for drug Lu 28–179 and Dollo et al.[Citation11] with reported yield of 44–74% for spray-dried drug 5-PDTT. The EY of coacervates was similar to other complex coacervation studies using similar wall materials. Siow and Ong[Citation25] reported the garlic oil coacervates yield of 65–86% and Tirkkonen et al.[Citation18] achieved 42–80% yield of drug indomethacin coacervates. The loading capacity for both methods () was comparable with other studies.[Citation26] O/W emulsion loading capacity () indicates that 0.5% of the microparticle weight is composed of xanthone, while 5.2% for coacervates. This is expected as O/W emulsion uses less xanthone mass compared to coacervates in the formulation, thus most of the loading capacity is occupied by canola oil to solubilize xanthone.
Table 1. Physicochemical characteristics of pure xanthone, spray-dried O/W emulsion and oven-dried coacervates (Mean ± S.D, n = 9).
In the current study, the EE for O/W emulsion () was lower compared to that of Garcia et al.[Citation30] who reported 86% EE in the spray drying of alpha-tocopherol microspheres with acetylated inulin. Coacervates showed higher EY (73.8 ± 2.3%) and have a slightly lower EE (34.5 ± 4.0%) compared to O/W emulsion (), which was probably due to xanthone leaching out from the coacervates as a result of twice washing with isopropyl alcohol.[Citation18]
Particle size analysis and aqueous solubility
The spray-dried O/W emulsion had a unimodal size distributions as depicted in ). Size distribution parameters in indicate that 10% of the microparticles (D[v,10]) had a particle size smaller than 14.7 ± 2.84 μm, 50% (D[v,50]) not more than 44.2 ± 2.08 μm and 10% (D[v,90]) bigger than 113 ± 5.01 μm. The particle size measurement of coacervates shows a Trimodal distribution as represented in . imply that 10% of the coacervates (D[v,10]) are particle size smaller than 15.2 ± 1.9 μm, 50% (D[v,50]) not more than 92.6 ± 10.7 μm and 10% (D[v,90]) bigger than 221.7 ± 73.2 μm.
Figure 1. (a). Particle size distribution profile of spray-dried xanthone O/W emulsion. (b). Particle size distribution profile of oven-dried xanthone coacervates.
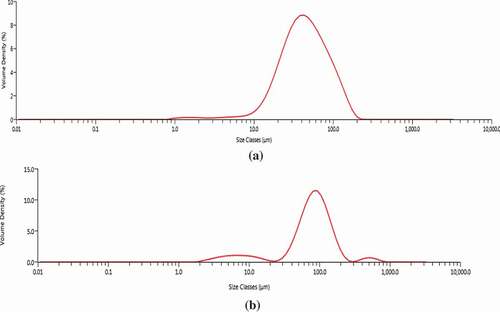
Comparing to pure xanthone, xanthone coacervates demonstrated about 1.6-fold increase in aqueous solubility, from 2.6 ± 0.5 μg/mL () to 4.1 ± 0.6 μg/mL (). On the other hand, O/W emulsion showed 37-fold improvement of aqueous solubility from 2.6 ± 0.5 μg/mL to 95.1 ± 10.9 μg/mL (). This shows that the solubilizing effect of oil and wall material contributed to the aqueous solubility improvement of xanthone in O/W emulsion. The increase in aqueous solubility of xanthone in coacervates is probably due to the solubilizing and wetting effect of the hydrophilic carrier.[Citation5,Citation24]
Moisture content, optical microscopy, colour and bulk density
Physical observation along with L*, a*, and b* coordinates () for the O/W emulsion and coacervates indicated that the powder was white in colour. Bulk density of the powder is the ratio of the mass of the solid particles and its volume including the interparticulate void volume.[Citation31] The bulk density of the O/W emulsion and coacervates were 0.2 ± 0.0 gmL−1 and 0.4 ± 0.0 gmL−1, respectively. The bulk density result is in agreement with microencapsulation of rosemary essential oil using inulin and blend of whey protein as wall material (0.23–0.35 gmL−1)[Citation32] and the encapsulation of flaxseed oil with maltodextrin (0.28 – 0.40 gmL−1).[Citation33] High bulk density powder occupies minor volume, thus utilizing a smaller amount of empty spaces, which is advantageous in terms of reducing the packaging cost.[Citation31]
Low moisture contents are favourable to prevent powder agglomeration as water acts as a plasticizer. The average moisture content of O/W emulsion was 0.7 ± 0.0% and met the recommended moisture content threshold for dry powder in food industries as it was below the suggested maximum value of 3–4%.[Citation13] Santana et al.[Citation31] also reported similar findings in spray dried pequi powder (0.3–1.9%). The low moisture content of the spray-dried powder could be explained by high inlet temperature during spray drying, which initiates fast water evaporation rate.[Citation31] On contrary, coacervates reported higher average moisture content of 9.4 ± 0.4%, similar to values as reported by Rocha-Selmi et al.[Citation34] for aspartame coacervates. The low oven drying temperature for the coacervates retained higher moisture compared to spray drying although the end product for both drying methods remained free flowing.
(A) shows the pure xanthone dispersed in water, while (B, C, D) presents the morphology for dried powders dispersed in water. Comparing with blank coacervate (. B) as the control, (C) indicates positive encapsulation of xanthone by complex coacervation wherein the xanthone particles are homogeneously distributed within the coacervate. Fine emulsion was observed in (D), indicating xanthone was dissolved in the O/W emulsion.
FESEM study
Surface morphology of the samples was observed by FESEM as shown in . Both microencapsulation methods resulted in microparticles with a smooth surface and were mostly spherical. The lack of fissures or cracks on the surface for both microencapsulation methods indicated coverage of the wall materials over the core. In general, smooth surfaces and spherical particle shape are considered favourable in the food applications owing to their excellent flow properties.[Citation3] Gallardo et al.[Citation35] observed similar non-wrinkled surface morphology for encapsulated linseed oil and suggested that high solid content of the emulsion prevented the formation of vacuoles inside the particles while drying. In this work, 20% total solid content was used, while 30% in Gallardo et al.[Citation35]’s work. Beirao-da-costa et al.[Citation22] microencapsulated oregano oil with inulin and reported powder with average particle size of 3–4.5 μm. Average particle sizes of coacervates have also been reported for lycopene coacervates (61–144 μm)[Citation19], oleoresin and soybean oil coacervates (43–100 μm)[Citation16], all of which utilising gelatin-gum Arabic as the wall material. In the current study of both spray-dried O/W emulsion and coacervates, various sizes of microparticles were observed in the FESEM micrographs ( & B). These FESEM images indicated that microparticles agglomeration increased the particle size distribution ( & B) for both powders. A study performed on the microencapsulation of pequi oil also reported similar observation and difficulty in determining the mean particle sizes by dynamic scattering technique due to microparticles agglomeration.[Citation36]
Fourier transform infrared spectroscopy (FTIR)
FTIR spectroscopic studies were performed to determine the interaction between core and wall. The presence of xanthone in the encapsulated samples was confirmed by the appearance of a band from the stretching of carbonyl –C = O group of xanthone in the region of 1650 cm−1. Besides that, characteristic aromatic bands of xanthone assigned to C = C bending (1300–1700 cm−1) and C-H bending (600–1000 cm−1) also appeared in both the spectrum of the sample ( and ).[Citation37,Citation38] In , O/W emulsion shows bands at 1243.1, 1342.5, 1456.6 cm and 668.4 −1 corresponding to pure xanthone that was not observed in blank powder. Additionally, notable peaks at 757.1, 881.5, 1144.3, 1239.1, and 1455.4 cm−1 corresponding to pure xanthone appeared in xanthone coacervates () that were not observed in the blank coacervates. There is, however, a reduction in the intensity of xanthone characteristic bands at 600–1700 cm−1, for both microencapsulation methods especially in O/W emulsion, compared to that of pure xanthone (). This was probably due to the lower loading capacity (0.5 ± 0.1%) of xanthone in the O/W emulsion method. Both microencapsulation methods show no difference in the IR patterns of the physical mixture of xanthone, blanks, and the samples, indicating no interaction between the core and wall material.
X-ray diffraction (XRD)
XRD gives information on powders state whether it is crystalline or amorphous.[Citation39] Crystalline powder will yield sharp and defined peaks, whereas amorphous powder yields broad and diffuse peaks.[Citation39] O/W emulsion () showed the absence of pure xanthone diffraction peaks. As there was no clear peak in the diffractogram of spray dried xanthone in o/w emulsion, this suggests that xanthone was converted from crystalline to amorphous after spray-drying in oil. On the other hand, coacervates diffractogram shows sharp diffraction peaks corresponding to pure xanthone, indicating xanthone in its crystalline form ().
Figure 5. X-ray diffractograms of spray-dried xanthone in O/W emulsion, oven-dried xanthone coacervates and pure xanthone.
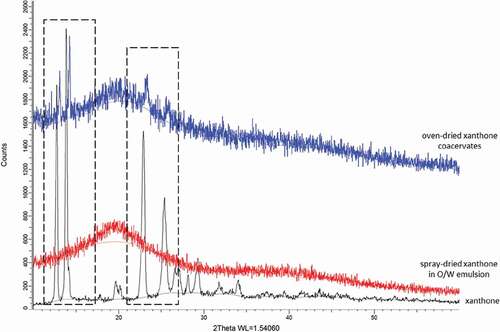
The drying temperature of 50°C used in coacervates was below the melting temperature of xanthone whereas the high drying temperature of 200°C in O/W emulsion was above the melting temperature of xanthone. This implies that drying temperature may play a role in amorphisation conversion wherein the high drying temperature of 200°C converted xanthone into amorphous state resulting in xanthone improved aqueous solubility. The reduction of diffraction peaks in coacervates indicates the decrease of percentage crystallinity of xanthone. The current result together with the particle size of the coacervates reveals that the enhancement of aqueous solubility is probably due to the decrease in xanthone crystallinity and increase in the surface area resulting in the improved wetting and solubility effect of the hydrophilic carrier.
Differential scanning calorimetry (DSC)
DSC is used to study the melting of a crystalline, quantify amorphous content and glass transition temperature in powders.[Citation39] According to Ho et al.[Citation39], under DSC scanning via open pans, evaporation behaviour of water/volatile components between crystalline and amorphous powders is clearly differentiated. Under open pan condition, DSC scan of amorphous powders will result in a big endothermic hump over a wide range of temperature resulting from the evaporation of water/volatile molecules due to its porous structure.[Citation39] Whereas, the highly packed structure of crystalline powders restricts evaporation of water/volatile molecules and result in one or few sharp endothermic peak.[Citation39] A study on amorphous spray-dried α-cyclodextrin powder observed an endothermic hump between 40 to 170°C in the DSC spectra from water evaporation. This was also observed in the O/W emulsion and coacervates ( and ).[Citation40]
Figure 6. HPLC chromatograms at 237 nm of A: pure xanthone; B: spray-dried xanthone in O/W emulsion; C: oven-dried xanthone coacervates.
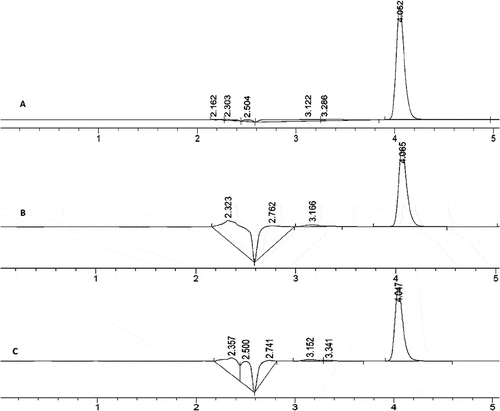
Figure 7. (a). DSC thermograms of spray-dried xanthone O/W emulsion, spray-dried blank O/W emulsion and pure xanthone under open pan condition. (b). DSC thermograms of spray-dried xanthone O/W emulsion under closed pan condition.
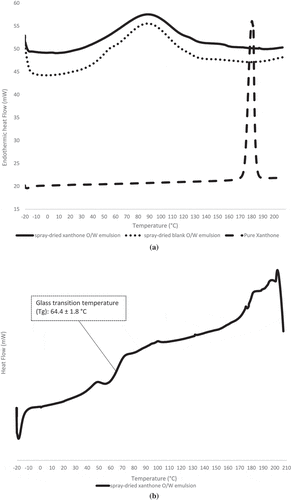
Figure 8. DSC thermograms of oven-dried xanthone coacervates, oven-dried blank coacervates and pure xanthone under open pan condition.
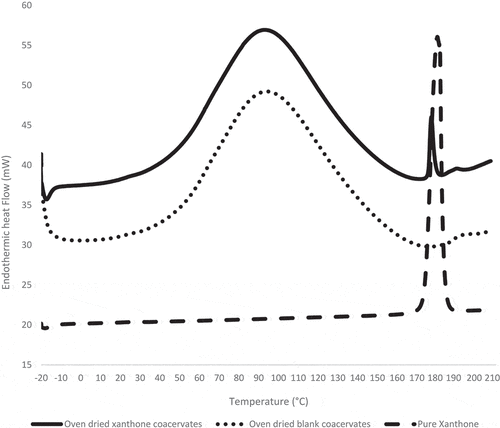
Xanthone showed a sharp endothermic melting peak at 177°C ( and ), which indicates its crystalline nature and confirms the previously reported melting temperature for xanthone.[Citation1,Citation3] DSC spectra of O/W emulsion shows the absence of xanthone melting peak () but FTIR () and HPLC () analysis confirmed the presence of xanthone in the O/W emulsion. Under closed pan conditions, O/W emulsion shows a glass transition temperature (Tg) of 64.4 ± 1.8°C (). This along with XRD result indicates xanthone amorphization. The current result further explains the enhancement of aqueous solubility of xanthone in the O/W emulsion, wherein the amorphous state of active substance induces higher dissolution rate and aqueous solubility.[Citation41–Citation42] A previous study has also reported the enhancement of aqueous solubility and physical stability of EUDRAGIT® E PO[Citation41] from amorphization. For oven-dried xanthone coacervates, the observed endothermic melting peak of xanthone at 177°C () confirmed xanthone in its crystalline state.
Conclusion
This study demonstrated that natural polymers and without the use of organic solvent improved xanthone aqueous solubility by 37-fold via spray-dried O/W emulsion and 1.6-fold through oven-dried complex coacervation. However, a drawback to the O/W emulsion method is the high ratio (1:30) of oil to xanthone required to solubilize xanthone. Both microencapsulation methods produced white free-flowing powders with different physical properties. FTIR, XRD and DSC results indicated no interaction between xanthone and the wall material and decreased xanthone crystallinity in both methods. The solubilizing effect of the oil and wall material, particle size reduction and xanthone amorphization enhanced xanthone solubility in O/W emulsion, whilst the solubilizing effect of the wall material for coacervates was probably responsible for the improved aqueous solubility of coacervates. Spray-dried O/W emulsion is potentially incorporated as functional ingredients into food emulsion system such as in ice cream or salad dressing as well as in cosmetic and pharmaceutical products whilst oven-dried complex coacervation is potentially used in beverage and non-emulsion system as well as pharmaceutical industries. The current study serves as a model to improve aqueous solubility of other xanthone derivatives.
Additional information
Funding
References
- Jiang, D. J.; Dai, Z.; Li, Y. J. Pharmacological Effects of Xanthones as Cardiovascular Protective Agents. Cardiovascular Drug Reviews 2004, 22, 91–102. DOI: 10.1111/j.1527-3466.2004.tb00133.x.
- Walker, E. B.;. HPLC Analysis of Selected Xanthones in Mangosteen Fruit. Journal of Separation Science 2007, 30, 1229–1234. DOI: 10.1002/(ISSN)1615-9314.
- Teixeira, M.; Alonso, M. J.; Pinto, M. M.; Barbosa, C. M. Development and Characterization of PLGA Nanospheres and Nanocapsules Containing Xanthone and 3-Methoxyxanthone. European Journal of Pharmaceutics and Biopharmaceutics 2005, 59, 491–500. DOI: 10.1016/j.ejpb.2004.09.002.
- Wan, B.-K.; Siow, L.-F. Spray Dried Xanthone: Physicochemical Properties, Storage Stability and Controlled Release. Journal of Food Process Engineering 2016, 1–11. DOI: 10.1111/jfpe.12407.
- Aisha, A. F.; Ismail, Z.; Abu-Salah, K. M.; Majid, A. M. Solid Dispersions of Alpha-Mangostin Improve Its Aqueous Solubility through Self-Assembly of Nanomicelles. Journal of Pharmaceutical Sciences 2012, 101, 815–825. DOI: 10.1002/jps.22806.
- De Souza, J. R. R.; Feitosa, J. P. A.; Ricardo, N. M. P. S.; Trevisan, M. T. S.; De Paula, H. C. B.; Ulrich, C. M.; Owen, R. W. Spray-Drying Encapsulation of Mangiferin Using Natural Polymers. Food Hydrocolloids 2013, 33, 10–18. DOI: 10.1016/j.foodhyd.2013.02.017.
- Lim, Y.-H.; Siow, L.-F. Spray Dried Xanthone in Oil Emulsion Using Inulin as Wall Material. Journal of Food Processing and Preservation 2016, n/a-n/a. DOI: 10.1111/jfpp.13038.
- Jang, D.-J.; Jeong, E. J.; Lee, H.-M.; Kim, B.-C.; Lim, S.-J.; Kim, C.-K. Improvement of Bioavailability and Photostability of Amlodipine Using Redispersible Dry Emulsion. European Journal of Pharmaceutical Sciences 2006, 28, 405–411. DOI: 10.1016/j.ejps.2006.04.013.
- Humberstone, A. J.; Charman, W. N. Lipid-Based Vehicles for the Oral Delivery of Poorly Water Soluble Drugs. Advanced Drug Delivery Reviews 1997, 25, 103–128. DOI: 10.1016/S0169-409X(96)00494-2.
- Hansen, T.; Holm, P.; Schultz, K. Process Characteristics and Compaction of Spray-Dried Emulsions Containing a Drug Dissolved in Lipid. International Journal of Pharmaceutics 2004, 287, 55–66. DOI: 10.1016/j.ijpharm.2004.08.014.
- Dollo, G.; Le Corre, P.; Guerin, A.; Chevanne, F.; Burgot, J. L.; Leverge, R. Spray-Dried Redispersible Oil-In-Water Emulsion to Improve Oral Bioavailability of Poorly Soluble Drugs. European Journal of Pharmaceutical Sciences 2003, 19, 273–280. DOI: 10.1016/S0928-0987(03)00134-9.
- Chakraborty, S.; Shukla, D.; Mishra, B.; Singh, S. Lipid – An Emerging Platform for Oral Delivery of Drugs with Poor Bioavailability. European Journal of Pharmaceutics and Biopharmaceutics 2009, 73, 1–15. DOI: 10.1016/j.ejpb.2009.06.001.
- Barroso, A. K. M.; Pierucci, A. P. T. R.; Freitas, S. P.; Torres, A. G.; Rocha-Leão, M. H. M. D. Oxidative Stability and Sensory Evaluation of Microencapsulated Flaxseed Oil. Journal of Microencapsulation 2014, 31, 193–201. DOI: 10.3109/02652048.2013.824514.
- Soottitantawat, A.; Bigeard, F.; Yoshii, H.; Furuta, T.; Ohkawara, M.; Linko, P. Influence of Emulsion and Powder Size on the Stability of Encapsulated D-Limonene by Spray Drying. Innovative Food Science & Emerging Technologies 2005, 6, 107–114. DOI: 10.1016/j.ifset.2004.09.003.
- Aniesrani Delfiya, D. S.; Thangavel, K.; Natarajan, N.; Kasthuri, R.; Kailappan, R. Microencapsulation of Turmeric Oleoresin by Spray Drying and in Vitro Release Studies of Microcapsules. Journal of Food Process Engineering 2015, 38, 37–48. DOI: 10.1111/jfpe.12124.
- Alvim, I. D.; Grosso, C. R. F. Microparticles Obtained by Complex Coacervation: Influence of the Type of Reticulation and the Drying Process on the Release of the Core Material. Food Science and Technology (Campinas) 2010, 30, 1069–1076. DOI: 10.1590/S0101-20612010000400036.
- De Jaeghere, W.; De Geest, B. G.; Van Bocxlaer, J.; Remon, J. P.; Vervaet, C.; Antunes Da Fonseca, A. Formulation of Poorly Water-Soluble Drugs via Coacervation–A Pilot Study Using Febantel. European Journal of Pharmaceutics and Biopharmaceutics 2013, 85, 930–935. DOI: 10.1016/j.ejpb.2013.07.006.
- Tirkkonen, S.; Turakka, L.; Paronen, P. Microencapsulation of Indomethacin by Gelatin-Acacia Complex Coacervation in the Presence of Surfactants. Journal of Microencapsulation 1994, 11, 615–626. DOI: 10.3109/02652049409051111.
- Rocha-Selmi, G. A.; Favaro-Trindade, C. S.; Grosso, C. R. F. Morphology, Stability, and Application of Lycopene Microcapsules Produced by Complex Coacervation. Journal of Chemical 2013, 2013, 7. DOI: 10.1155/2013/982603.
- Burgess, D. J.; Ponsart, S. beta-Glucuronidase Activity following Complex Coacervation and Spray Drying Microencapsulation. Journal of Microencapsulation 1998, 15, 569–579. DOI: 10.3109/02652049809008241.
- Robert, P.; García, P.; Reyes, N.; Chávez, J.; Santos, J. Acetylated Starch and Inulin as Encapsulating Agents of Gallic Acid and Their Release Behaviour in a Hydrophilic System. Food Chemistry 2012, 134, 1–8. DOI: 10.1016/j.foodchem.2012.02.019.
- Beirão-da-Costa, S.; Duarte, C.; Bourbon, A. I.; Pinheiro, A. C.; Januário, M. I. N.; Vicente, A. A.; Beirão-da-Costa, M. L.; Delgadillo, I. Inulin Potential for Encapsulation and Controlled Delivery of Oregano Essential Oil. Food Hydrocolloids 2013, 33, 199–206. DOI: 10.1016/j.foodhyd.2013.03.009.
- Szydłowska-Czerniak, A.; Karlovits, G.; Hellner, G.; Dianoczki, C.; Szłyk, E. Effect of Enzymatic and Hydrothermal Treatments of Rapeseeds on Quality of the Pressed Rapeseed Oils: Part I: Antioxidant Capacity and Antioxidant Content. Process Biochemistry 2010, 45, 7–17. DOI: 10.1016/j.procbio.2009.07.016.
- Yan, C.; Zhang, W. Chapter 12 - Coacervation Processes. In Microencapsulation in the Food Industry; Gaonkar, A. G., Vasisht, N., Khare, A. R., Sobel, R., Ed.; Academic Press: San Diego, 2014.
- Siow, L. F.; Ong, C. Effect of pH on Garlic Oil Encapsulation by Complex Coacervation. Journal of Food Process Technology 2013, 4, 199.
- Govender, T.; Stolnik, S.; Garnett, M. C.; Illum, L.; Davis, S. S. PLGA Nanoparticles Prepared by Nanoprecipitation: Drug Loading and Release Studies of a Water Soluble Drug. Journal of Controlled Release 1999, 57, 171–185. DOI: 10.1016/S0168-3659(98)00116-3.
- Dixit, M.; Kini, A. G.; Kulkarni, P. K. Preparation and Characterization of Microparticles of Piroxicam by Spray Drying and Spray Chilling Methods. Research Pharmacology Science 2010, 5, 89–97.
- AOAC. Official Methods of Analysis of AOAC International, 17 ed.; AOAC: Gaithersburg, Maryland, 2000.
- Goula, A. M.; Adamopoulos, K. G. Spray Drying of Tomato Pulp: Effect of Feed Concentration. Drying Technology 2004, 22, 2309–2330. DOI: 10.1081/DRT-200040007.
- García, P.; Vega, J.; Jimenez, P.; Santos, J.; Robert, P. Alpha-Tocopherol Microspheres with Cross-Linked and Acetylated Inulin and Their Release Profile in a Hydrophilic Model. European Journal of Lipid Science and Technology 2013, 115, 811–819. DOI: 10.1002/ejlt.v115.7.
- Santana, A. A.; Kurozawa, L. E.; De Oliveira, R. A.; Park, K. J. Influence of Process Conditions on the Physicochemical Properties of Pequi Powder Produced by Spray Drying. Drying Technology 2013, 31, 825–836. DOI: 10.1080/07373937.2013.766619.
- Fernandes, R. V.; Borges, S. V.; Botrel, D. A. Gum Arabic/Starch/Maltodextrin/Inulin as Wall Materials on the Microencapsulation of Rosemary Essential Oil. Carbohydrate Polymers 2014, 101, 524–532. DOI: 10.1016/j.carbpol.2013.09.083.
- Carneiro, H. C. F.; Tonon, R. V.; Grosso, C. R. F.; Hubinger, M. D. Encapsulation Efficiency and Oxidative Stability of Flaxseed Oil Microencapsulated by Spray Drying Using Different Combinations of Wall Materials. Journal of Food Engineering 2013, 115, 443–451. DOI: 10.1016/j.jfoodeng.2012.03.033.
- Rocha-Selmi, G. A.; Bozza, F. T.; Thomazini, M.; Bolini, H. M. A.; Fávaro-Trindade, C. S. Microencapsulation of Aspartame by Double Emulsion Followed by Complex Coacervation to Provide Protection and Prolong Sweetness. Food Chemistry 2013, 139, 72–78. DOI: 10.1016/j.foodchem.2013.01.114.
- Gallardo, G.; Guida, L.; Martinez, V.; López, M. C.; Bernhardt, D.; Blasco, R.; Pedroza-Islas, R.; Hermida, L. G. Microencapsulation of Linseed Oil by Spray Drying for Functional Food Application. Food Research International 2013, 52, 473–482. DOI: 10.1016/j.foodres.2013.01.020.
- Carvalho Da Silva, L.; Alves Do Nascimento, M.; Guabiraba Mendes, L.; Ferro Furtado, R.; Correia Da Costa, J. M.; Luiz Herzog Cardoso, A. Optimization of Cashew Gum and Chitosan for Microencapsulation of Pequi Oil by Complex Coacervation. Journal of Food Processing and Preservation 2018, e13538. DOI: 10.1111/jfpp.13538.
- Purev, O.; Oyun, K.; Odontuya, G.; Tankhaeva, A. M.; Nikolaeva, G. G.; Khan Khalid, M.; Ali Shah Syed, T.; Voelter, W. Journal 2002, 57, 331.
- Negi, J. S.; Bisht, V. K.; Singh, P.; Rawat, M. S. M.; Joshi, G. P. Naturally Occurring Xanthones: Chemistry and Biology. Journal of Applied Chemistry 2013, 2013, 9. DOI: 10.1155/2013/621459.
- Ho, T. M.; Truong, T.; Bhandari, B. R. Methods to Characterize the Structure of Food Powders - a Review. Bioscience Biotechnology and Biochemistry 2017, 81, 651–671. DOI: 10.1080/09168451.2016.1274643.
- Ho, T. M.; Howes, T.; Bhandari, B. R. Characterization of Crystalline and Spray-Dried Amorphous α-cyclodextrin Powders. Powder Technology 2015, 284, 585–594. DOI: 10.1016/j.powtec.2015.06.027.
- Patel, B. B.; Patel, J. K.; Chakraborty, S. Solubility Enhancement Using Poly(Meth)Acrylate Based Solid Dispersions. Powder Technology 2015, 270, Part A, 27–38. DOI: 10.1016/j.powtec.2014.10.006.
- Yu, L. Amorphous Pharmaceutical Solids: Preparation, Characterization and Stabilization. Advanced Drug Delivery Reviews 2001, 48, 27–42. DOI: 10.1016/S0169-409X(01)00098-9.