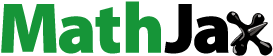
ABSTRACT
Clove oil was emulsified in 1% w/w chitosan (CC emulsions) and 2.5% w/w sodium alginate matrix (CA emulsions) containing Tween 80 as the surfactant. Different homogenization speeds (5,000, 10,000, 15,000 and 20,000 rpm) were used to produce the emulsions, and the stability of the emulsions during storage (29 days) was determined. The stability of the emulsions containing clove oil prior to the solidification process was assessed when chitosan and sodium alginate were used as encapsulating materials. Different homogenization speeds resulted in polydisperse emulsions with a size of 2–3 μm and 90% of stability after 29 days of storage. Different homogenization speeds did not significantly affect the concentrations of the active compounds contained in the emulsions. However, these concentrations changed significantly after 29 days of storage when sodium alginate was used to make the emulsions and the homogenization speeds were ≥ 10,000 rpm. High temperature caused by the high viscosity of the solution and high energy dissipation during homogenization suggested that the emulsions composed of sodium alginate were unstable. Chitosan enabled a longer processing time during the clove oil encapsulation process compared to sodium alginate, when emulsification by homogenization was used. The stability of the emulsion of the clove oil-in-chitosan matrix prior to the solidification step was superior.
Introduction
Clove oil is an essential oil extracted from the flowers, buds, leaves, and stem of the clove tree (Syzygium aromaticum) containing active compounds including eugenol, eugenol acetate, and β-caryophyllene[Citation1–Citation4], which are responsible for the functional properties of the oil (e.g. anti-oxidant activity[Citation5–Citation7], free radical scavengers[Citation8,Citation9], anti-stress[Citation10], and anti-microbial agent[Citation11–Citation13]). Clove oil can be applied to any product, such as food, health, and personal care products, but its application must be done with great care. Although clove oil is categorized generally recognized as safe (GRAS) by the United States Food and Drug Administration (US FDA) as a food additive, it is toxic for human cells via ingestion and injection, and an oral dose of 3.75 g/kg body weight is lethal. During storage, clove oil is chemically unstable in the presence of air, light, moisture, and high temperatures.[Citation14]
Other products often contain eugenol as one of their ingredients. Eugenol is slightly soluble in water, with an octanol-water partition coefficient of approximately two[Citation15], and therefore clove oil shares this property slightly soluble in water. The formulation of encased clove oil or eugenol, such as microencapsulation, nanoencapsulation, or liposomes, could address the limitations of clove oil/eugenol in terms of toxicity and stability in environmental conditions, for example, solubilize in water phase.[Citation16–Citation18] In addition, encapsulated eugenol is suggested to have enhanced functional activities, compared to un-encapsulated eugenol.[Citation13,Citation19] Nanoliposome-encapsulated clove oil has selective, longer acting antimicrobial activity compared to the free oil, due to the oil in the liposome being released only at infectious sites.[Citation12,Citation20] Clove oil encapsulated in β-cyclodextrin (BCD) has the greatest minimum inhibitory concentration and bactericidal concentration (MIC, MBC) against Salmonella Typhimurium LT2. However, free eugenol has higher MIC and MBC compared to encapsulated eugenol.[Citation21] Other studies suggest that encapsulating clove oil or eugenol using nanoparticles or nanoemulsions increases the stability of the compounds.[Citation16,Citation22,Citation23] Recent findings demonstrate that encapsulating clove oil and salvia oil using nanoliposomes improves the stability of the oils prior to administration.[Citation12,Citation24]
One of the successful parameters for encapsulating clove oil or its active compounds is the selection of a material that will act as a capsule wall or shell. Selection of this material is determined by properties of the core, microencapsulation techniques, and dehydration processes.[Citation25] Many food-grade materials have been studied for the use in encapsulating clove oil or eugenol, such as chitosan and sodium alginates, which were used in this study. Alginates are a group of naturally occurring polysaccharides, and their commercial form in a sodium salt has been widely used for encapsulating cells, pharmaceutical compounds, and essential oils.[Citation26–Citation30] Formulating alginate-based particles can only be achieved using a cross-linking formation, such as ionic cross-linking, covalent cross-linking, thermal gelation, and cell-crosslinking.[Citation31] Alginate is a suitable material to encapsulate natural antioxidant compounds. Sodium alginate hydrogel beads formed by ionic cross-linking with CaCl2 do not interact with lemon balm extract, and the antioxidant activity of the extract did not change after encapsulation.[Citation32] However, encapsulation efficiency of oil containing drug has been reported to be inversely proportional to the size of alginate-based particles.[Citation26] Encapsulation efficiency is greatly influenced by the degree of cross-linking. Therefore, alginate concentration, oil volume fraction, and alginate type are important parameters in designing the capsule.[Citation33] Encapsulation efficiency of various essential oils in cross-linked alginate has been reported to be 90%–94%, and their antifungal activity is maintained for a longer time period, compared to unencapsulated essential oils.[Citation30]
Chitosan is another polymer that is often used for encapsulation. The main difference to sodium alginate is the charge: chitosan has a positive charge, and alginate has a negative charge. Chitosan is a partial N-deacetylated derivative of chitin, and it is naturally abundant. Chitosan is well known for its applications in drug-delivery systems.[Citation34] It is able to form a gel at low pH, which is non-toxic and easily absorbed in the Human gut.[Citation35] Chitosan exhibits high antimicrobial activity against various pathogenic and spoilage microorganisms and is widely used in food preservation.[Citation36] In addition, chitosan has received worldwide interest in food applications due to its GRAS and its biodegradable[Citation37] biocompatible[Citation38] capabilities, and it has ability to form films, membranes, beads, fibers, and particles.[Citation35] Various essential oils have been successfully encapsulated using chitosan.[Citation39–Citation45] The concentration of chitosan significantly affects encapsulation efficiency of citronella oil, that is, increasing chitosan concentration decreases the efficiency of encapsulation.[Citation39] The size of the capsules is affected by mechanical forces, such as stirring or homogenization speed[Citation39] or homogenization pressure[Citation46], that is, within the nanometre to micrometre scale. Nanoscale capsules are consistently made in the form of liposome, in which liposome with an initial size of 115 nm increased to 250 nm when the liposome was coated by thiolated chitosan. However, the encapsulation efficiency and drug loading significantly increases when the liposome is coated.[Citation47]
The encapsulation process of an essential oil is generally done by emulsification followed by solidification.[Citation16,Citation42,Citation48] There are few reports in the literature describing the stability of the emulsion droplets, as the intermediate product during encapsulation process. It is generally accepted that the emulsions are likely to be stable during the solidification process. The stability of the emulsion stability is important in batch processes of encapsulation, where emulsification and solidification are not continuous processes. The stability would affect the capsules resulting from the solidification process. One study describing emulsion stability prior to solidification[Citation33] suggests that the loading concentration of the alginates and oil affects the stability of the oil-in-water emulsion droplets within one hour observation before solidification. This study examines the stability of clove oil in chitosan and sodium alginate matrix in the form of an oil-in-water emulsion. The waiting period prior to solidification was represented as storage time.
Materials and methods
Materials
Clove oil with purity of 99.5% (Rectified Clove Leaf Oil 85860, CAS # 8000–34-8) and eugenol with reported purity of 99.5% (USP 906, CAS # 97–53-0) were kindly provided by PT. Indesso Aroma, Indonesia. Clove oil and eugenol were extracted from clove leaf oil by fractional distillation. Miglyol 812N, a medium chain triacylglyceride (MCT) mixture, was purchased from Cremer Oleo GmbH Co. KG (Hamburg, Germany). Medium molecular weight of chitosan with 75–85% degree of deacetylation (CAS # 9012–76-4, Sigma Aldrich, USA) and sodium alginate with viscosity of 80–120 mPa.s in 10 g/L at 20°C (Wako Pure Chemical Industries Ltd., Osaka, Japan) were used in the continuous phase. Polyoxyethylene (20) sorbitan monooleate (Tween 80®, CAS # 822187, Merck, Germany) was used as the surfactant. Suprapur® Acetic acid (glacial) 100% (CAS # 64–19-7, Merck, Germany) was used to dissolve chitosan. n-hexane (GR ACS Reag., Ph Eur, CAS #110–54-3, Merck, Germany) and methanol (Merck, Germany) were used to dilute clove oil.
Characterization of eugenol and clove oil
Clove oil and eugenol were characterized in terms of its compounds using gas chromatography mass spectrometry (GC-MS) analysis. GC-MS (Agilent Technology 7890B, 5977A, USA) analysis was performed using non-polar capillary column (DB-5MS, 30 m × 0.25 mm, film thickness 0.25 µm, Agilent). Oven temperature conditions were: 40ºC for 1 min, the heating profile from 40°C to 280°C at a rate of 10°C/min. Injector temperature was 280°C. The carrier gas was helium at a linear velocity of 1 mL/min and pressure of 48.75 kPa. 100 µL of clove oil and eugenol was diluted 100 times with hexane, and then, 0.2 µL of sample was injected to the column. The compounds were identified by matching their mass spectra with those from GC-MS libraries (NIST 14 and Wiley Registry).
Eugenol identification was specifically confirmed by calculating Kovats retention index (RI), in addition to library matching. Eugenol and two references of n-alkane hydrocarbons were eluted in GC-MS using five non-isothermal methods in order to determine the RI as follows: first, the temperature was held at 70°C for 1 min, and then it was increased from 8 to 230°C for 10 min with helium flow rate of 1 mL/min; second, the temperature was held at 50°C for 1 min, and then it was increased from 15 to 280°C for 4 min with helium flow rate of 1 mL/min; third, the temperature was held at 40°C for 1 min, and then it was increased from 10 to 280°C for 5 min with helium flow rate of 0.8 mL/min; fourth, the temperature was held at 40°C for 1 min, and then it was increased from 10 to 280°C for 5 min with helium flow rate of 1.3 mL/min; and the last, the temperature was held at 40°C for 1 min, and then it was increased from 10 to 280°C for 5 min, with helium flow rate of 1 mL/min. The retention times were used to calculate RI using Eq. (1).
where is the retention time, subscript n and N indicate the reference of n-alkane hydrocarbons eluting immediately before and after eugenol, respectively, and z is the difference of number of carbon atoms between n and N.
Viscosity measurement
Viscosity of 1% (w/w) chitosan and 2.5% (w/w) sodium alginate was measured using a concentric-cylinder measuring system (CC17) attached to the Peltier temperature device for concentric-cylinder system (C-PTD 200, Rheometer Anton Paar MCR301, Anton Paar GmbH, Austria). Shear rate sweep with up ramp from 1 s−1 to 500 s−1 and down ramp from 500 s−1 to 1 s−1 was performed at 30°C. The measurement was performed in duplicate for each sample, and the average viscosity as the function of shear rate was presented.
Preparation of emulsions
Clove oil-in-water emulsions were prepared according to the method of Terjung et al.[Citation46] with modification. The oil phase consisted of Miglyol 812N and clove oil with ratio of 1:1. The continuous phase was either chitosan solution or sodium alginate solution containing 2% (w/w) Tween 80. 1% (w/w) Chitosan solution was prepared according to the method of Hosseini et al.[Citation42] Solution of 2.5% (w/w) sodium alginate was prepared according to the method of Chan.[Citation33] The emulsion consisted of 10% (w/w) of the oil phase and 90% (w/w) of the continuous phase. The oil phase was slowly dispersed into the continuous phase during homogenization using a high-speed homogenizer (T25 Ultra-Turrax®, IKA, Germany) equipped by S25N – 25F dispersing element (IKA, Germany). The homogenization speed was set at 5,000–20,000 rpm for 10 min. The emulsification was performed in ice-water mixture to avoid excessive heat during homogenization. The emulsions were prepared in two batches for each continuous phase. The emulsions were further analyzed directly after preparation, which was labeled as the emulsion at day 0, and after storage, which was labeled as emulsion at day 7, 14, 21, and 28, respectively, except for analysis of active compounds of clove oil. The prepared emulsions were stored in the dark at 8–10ºC.
Measurement of fluid properties
The densities of the continuous phase and the dispersed phase were measured with a portable density meter (DA-130N, KEM, Kyoto Electronics, Kyoto, Japan) at 27°C. The measurement of each phase was performed in triplicate. The static equilibrium interfacial tension between the oil mixture and the continuous phase, either chitosan or sodium alginate solution, containing Tween 80 was measured using a pendant drop method (PD-W, Kyowa Interface Science Co., Ltd., Saitama, Japan). A drop of chitosan or sodium alginate solution was suspended in the oil mixture, and the interfacial tension between chitosan/sodium alginate solution and the oil mixture was recorded. The interfacial tension between water and the oil mixture was also measured as the reference. The measurements were done in triplicate, and the results were presented as the average interfacial tension.
Zeta potential
The ζ-potential of chitosan solution, alginate solution, and freshly made emulsions were measured using Zetasizer (Zetasizer NanoZS, Malvern Instruments, UK). On each measurement, three to ten measurements were performed. The results were presented as the mean value of ζ-potential.
Stability evaluation
Size of emulsion droplets
The mean diameter of emulsions was measured by particle size analyzer (CILAS 1190, France) that works on the laser diffraction principle. The measurement for each batch of emulsions was performed in triplicate, and the results were reported as the volume mean diameter (d4,3).
Physical stability
Physical stability of emulsion was evaluated by visual observation based on the resistance of the emulsion systems against coalescence or Ostwald ripening that led to separation in the emulsion system. The appearance of separation that might occur in the emulsion systems was quantified according to Sapei et al.[Citation49] with slight modification. Freshly made emulsions were poured into 20 mL glass vial with airtight screw-cap to a height of ~6 cm. The height of layer in emulsions with color other than milky white was measured every 7 days. The emulsion stability against separation was defined as follows:
where S is the stability, h0 is the initial height of emulsion in the glass vial, and ht is the height of emulsion at the measuring time.
Microscopy
A drop of emulsion was placed onto a microscope slide and gently covered with a cover slip. The emulsion was observed under a light microscope (BestScope International Ltd., China) equipped with CCD camera and connected to PAX-it imaging software for image acquisition. The observation was performed at 27°C.
Active compounds of clove oil in the continuous phase
The active compounds of clove oil in the continuous phase were determined by separating the emulsions from the continuous phase as the first step, according to Terjung et al.[Citation46] with modification. About 2 mL of the emulsion was placed into centrifuge tubes and centrifuged at 10,000 × g for 60 min at 4ºC (Sorvall Fresco, USA). A sample from the clear, upper continuous phase was withdrawn with syringe. Active compounds contained in this phase were determined using GC-MS analysis. Samples of 100 µL were then mixed with 100 µL methanol in order to extract dissolved clove oil in the continuous phase. The mixture of sample and methanol was then diluted with 1 mL hexane (1st dilution). The 1st dilution was allowed to stand for 1 h at room temperature, and then it was sonicated for 20 min. The sample from the 1st dilution was further diluted with hexane until reasonable height of chromatograms in GC-MS was obtained. In total, the continuous phase was diluted 50000 × with hexane. The sample volume injected to GC-MS column was 0.2 µL, and the measurement setup of GC-MS was the same as the measurement set up described in characterization of eugenol and clove oil. The analysis of the active compounds was performed at 1, 8, 15, 22, and 29 days after storage. The concentration of a detected compound (% C) was calculated by comparing peak area of the detected compound (Ad) with the total area of all detected compounds (Ac) as follows:
Statistical analysis
All experiments were done in parallel, each sample was measured in duplicates, and the data was processed using SAS® program. The effects of homogenization speed and storage time to emulsion size, emulsion stability, and content of the active compounds in the emulsions were justified by analyzing the data using one-way analysis of variance (ANOVA) followed by Duncan’s multiple range test (DMRT). p values < 0.05 were considered as statistically significant.
Results and discussion
Characteristics of eugenol and clove oil
GC-MS analysis indicated that eugenol had a purity of 99.4%, containing 0.6% of un-identified traces. This value was similar to that published by the manufacturer (99.5%). Eugenol had different retention times when five non-isothermal methods were programmed into GC-MS. The methods number one to five resulted in retention times of 10.92, 9.04, 12.98, 12.20, and 12.61 min, respectively. Eugenol was found to have different retention times, but similar RI, that is, 1365 with coefficient of variance of 0.13%. This RI value was used as the reference to determine eugenol in further analysis.
The chromatogram analysis of Indonesian clove oil identified 19 compounds, with a total of 96.84% containing 3.16% of un-identified traces, shown in . A typical histogram obtained from gas chromatography is shown in with three major peaks indicating eugenol (43.66%), caryophyllene (29.17%), and humulene (16.98%). Eugenol acetate, which was reported to be one of the major compounds[Citation1–Citation4], was not detected in this study. Wenqiang et al. (2007)[Citation4] reported eugenol acetate in clove oil extracted from buds of clove plants to be between 3.9% and 19.4%, which was dependent on the extraction method used. The extraction of clove oil using hydro and steam distillation resulted in the lowest amount of eugenol acetate extracted, but high amounts of caryophyllene and humulene. Kegley et al. (2010)[Citation2] reported that eugenol acetate in clove oil could be as low as 0.5%. However, other factors may govern the relative quantities of different compounds in clove oil, such as plant genetics, climate, soil and cultivation techniques, the part of the plant extracted, and the extraction method. Clove oil in this study originated from the leaves, and hydro/steam distillation was used to extract the oil. This could explain the un-identified eugenol acetate, and other factors, as described previously. Nineteen compounds in clove oil were detected in this study. Across the literature, there are a range of compounds reported, for example, 11 to 20 chemical compounds in Chinese clove bud oil[Citation4], 11 compounds in Indonesian clove oil, and 20 compounds in Indian clove oil[Citation50], 38 compounds in Bangladesh clove leaf oil, and 31 compounds in the clove bud oil[Citation51], 18 compounds from Turkish clove bud oil[Citation52], and16 compounds from Egyptian clove bud oil.[Citation53] However, all reported studies indicate that the main chemical compound in clove oil is eugenol.
Table 1. Chemical composition of Indonesian clove oil. The sequences in the table are from the lowest to the highest tr.
Density and interfacial tension of the continuous and dispersed phases
The oil mixture between clove oil and MCT with a ratio of 1:1 resulted in an oil density of 994.5 ± 0.5 kg/m3. Clove oil density is reported to be higher than the density of water.[Citation54] Mixing clove oil with MCT decreased the oil density. The density of the chitosan solution was 1004.9 ± 0.1 kg/m3 and that of the alginate solution was 1013.20 ± 0.05 kg/m3. The interfacial tension between clove oil–MCT mixture and water was 8.5 ± 0.3 mN/m. This value decreased when water was replaced by a solution of chitosan or sodium alginate containing Tween 80. The interfacial tension between the oil mixture and chitosan solution containing Tween 80 was 0.6 ± 0.1 mN/m. A slightly lower value was obtained from an oil mixture and sodium alginate solution containing Tween 80, that is, 0.5 ± 0.1 mN/m. These lower values of interfacial tension are required by the oil and chitosan/sodium alginate solution to form emulsions.
Viscosity
The viscosity of sodium alginate and chitosan solutions during up ramp and down ramp is shown in . Sodium alginate solution showed distinct shear thinning, whereas chitosan solution had only a slight shear thinning. This is in agreement with other studies describing the flow behaviour of sodium alginate[Citation55,Citation56] and chitosan.[Citation57] At 30°C, the viscosity values of 1% w/w chitosan solution were in 187.2 mPa s at 1 s−1 and 83.7 mPa s at 500 s−1, and values of 2.5% w/w sodium alginate solution were 797.2 mPa s at 1 s−1 and 351.8 mPa s at 500 s−1. Coefficients of variance obtained for all data points were less than 1%, which indicated accurate measurements. The differences in viscosity values and the flow behavior could be attributed to the molecular weight, the material from which alginate or chitosan was extracted, ionic strength, temperature, and other processing conditions. The viscosity returned to its original state upon down ramping, indicating re-entanglement of the structures. This may suggest the structures of both polymers are unchanged after homogenization.
ζ-potential
confirms that chitosan and sodium alginate have positive and negative charges, respectively. Developing the two polymers for encapsulating clove oil did not change their charges; emulsions of clove oil-in-chitosan (CC emulsions) and of clove oil-in-sodium alginate (CA emulsions) took on the original charges of the polymers. The ζ-potential is a magnitude of repulsive interaction between colloidal particles and is commonly used as an indication for emulsion stability.[Citation58] The experiments showed that freshly made CC and CA emulsions had a ζ-potential of 32.07 mV and −49.8 mV, respectively. These values indicated that the fresh emulsions were stable. Riddick (Citation1968)[Citation59] mentioned that a ζ-potential value of greater than ± 60 mV indicates an emulsion system with excellent stability, and greater than ± 30 mV indicates an emulsion system with good physical stability.
Table 2. ζ-potential of hydrocolloids solutions and the emulsion systems.
Table 3. Clove oil in chitosan (CC) and sodium alginate (CA) matrix parameters with reference to homogenization speeds and storage time. (a) The influence of homogenization speeds and (b) storage time.
Effect of homogenization speed
Freshly made CC emulsions prepared at various homogenization speeds were homogenously milky white. The polydisperse emulsions are shown in . The polydispersity is generally obtained using conventional emulsification methods due to the methods using instruments with variable shear and pressure fields.[Citation60,Citation61] The freshly made CA emulsions were also polydisperse (). Polydispersity in CC and CA emulsions was also shown by the particle size distribution ()) that has two peaks of density distribution. Further, CC and CA emulsions were similar in color, and all homogenization speeds resulted in CA emulsions with a thin opaque layer at the top. This indicated that spontaneous instability may take place after preparation. In addition, there was a significant increase in temperature of CA emulsions after preparation that may affect the stability of the emulsions. Apparent viscosities of 2.5% w/w sodium alginate solution, which were four times higher than those of 1% w/w chitosan solution, could explain the temperature increase during homogenization. The movement of a highly viscous solution through the thin space between the rotor and stator of the homogenizer could result in a large amount of energy dissipating caused by frictional losses, accounting for the increase in temperature. Therefore, the emulsification was carried out in an ice-water mixture to avoid excessive heating. However, this was not sufficient to avoid a temperature increase in the CA emulsions. Other cooling methods, such as applying liquid nitrogen during homogenization, may provide better control against excessive heating.
Figure 3. Microscopy images of clove oil emulsions prepared at various homogenization speeds (a) chitosan and (b) sodium alginate. The scale bar is 10 μm.
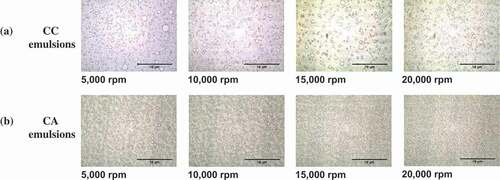
Figure 4. Droplet size and distribution of clove oil emulsions in chitosan (CC) and sodium alginate (CA) matrix. (a) Typical size distribution of CC and CA emulsions. (





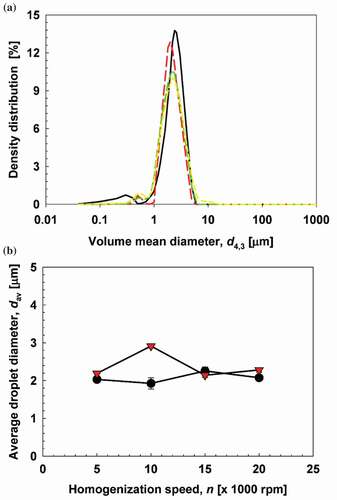
Freshly made CC and CA emulsions homogenized at 5,000 rpm had an average diameter of approximately 2– 3 μm. Increasing the homogenization speed to 10,000, 15,000 and 20,000 rpm also resulted in average diameters of approximately 2–3 μm. indicates that there was no significant effect of homogenization speed on the average diameter of the emulsion droplets. Others had reported that increasing the speed of the homogenizer reduced the size of the emulsion droplets. The reduced mean size of poly (D, L lactide-co-glycolide) (PLGA) nanoparticles was consistent with an increase in homogenization speed[Citation38] and that has been reported with water-in-oil emulsions containing vitamin C.[Citation62] Homogenization speeds greater than 12,000 rpm do not reduce the particle size[Citation38], and lowering the homogenization speed below 5,000 rpm results in incomplete homogenization.[Citation62] A speeds above 5,000 rpm, the high energy level exerted by 10,000, 15,000 and 20,000 rpm, was not favorable to further break up the emulsion. Therefore, the low energy produced by a homogenization speed of 5,000 rpm was sufficient to break up droplets of chitosan and sodium alginate containing clove oil-MCT mixture. This could be explained by the low interfacial tension between the clove oil-MCT mixture and chitosan solution or between the oil mixture and sodium alginate solution, that is, 0.6 mN/m and 0.5 mN/m, respectively. Purwanti et al. (2016)[Citation63] proposed that a reduction of interfacial tension between dispersed and continuous phases is one of the success factors in forming emulsions. The interfacial tension also correlates to the size of the emulsion droplets[Citation64,Citation65] in which low interfacial tension produces a smaller size emulsion droplet. Purwanti et al.[Citation54] reported the interfacial tension between clove oil and 0.1% sodium dodecylsulfate (SDS) solution was 2.82 mN/m resulting in a larger size of emulsion droplets compared to emulsion droplets composed of clove oil and 0.5% SDS solution, with an interfacial tension of 0.7 mN/m.
Physical stability
The resistance of CC and CA emulsions against separation is expressed as S (%), which is defined in Eq. (2), . The initial stability for CC emulsions was 100% at all homogenization speeds. The initial stability for CA emulsions was not 100% initially, indicating that instability had already occurred immediately after emulsion preparation. The instability of CA emulsions was marked by the formation of a thin layer at the top of the emulsion. This layer was slightly oily and probably the result of droplet flocculation that led to coalescence of oil droplets, as shown in the inserted images in and possibly Ostwald ripening. The lower density of the oil mixture compared to the density of the continuous phase result in the rising of oil when instability occurred. Stability of the CA emulsions in this study was not in agreement with other reported studies. Chan[Citation33] demonstrated completely stable emulsions were obtained within one hour using sodium alginate in a continuous phase, when palm oil loading was 10–40% (w/w) and the alginate concentration was 2.5% (w/v). The polarity of the oil could affect the stability of the emulsions[Citation63] and the polarity may be indicated by the interfacial tension between the oil and pure water. The interfacial tension between palm oil and water is in the range of 10.1–26.4 mN/m when measured at 60°C[Citation66], which is higher than the interfacial tension between clove oil-MCT mixture and water. Higher interfacial tension between the oil and aqueous phases would produce more a stable emulsion system, compared to emulsions with low interfacial tension, as reported previously by Purwanti et al. (2016).[Citation63]
Figure 5. Stability of emulsions during 28 days of storage. Solid lines show CC emulsions, and dotted lines show CA emulsions. (
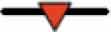
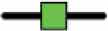


Although the initial stability of the CC emulsion was higher than CA emulsions, both CC and CA emulsions had similar stability values after 28 days of storage, that is, 91%. This could be attributed to continuous droplet flocculation and coalescence as a consequence of polydispersity in both emulsion systems, resulting in two separate layers being observed during storage. indicates that stability of CC emulsions changed significantly during 28 days; meanwhile, the stability of CA emulsions changed significantly to the same level of stability as that of CC emulsions during 21 days. This physical stability could imply importance of time frame to complete the encapsulation processes if clove oil is encapsulated within a chitosan and sodium alginate matrix. The encapsulation process, particularly the drying step, must be completed before the emulsions start to destabilize.
Size of emulsion droplets during storage
The average size of CC emulsions, prepared at all homogenization speeds, was relatively constant at approximately 2 μm during storage. The average size of CA emulsions was 2–3 μm during storage,
Figure 6. Average droplet diameter of CC and CA emulsions during 28 days of storage. The images show the course of emulsion coalescence. Solid lines show CC emulsions, and dotted lines shoe CA emulsions. (
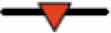
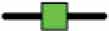
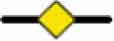
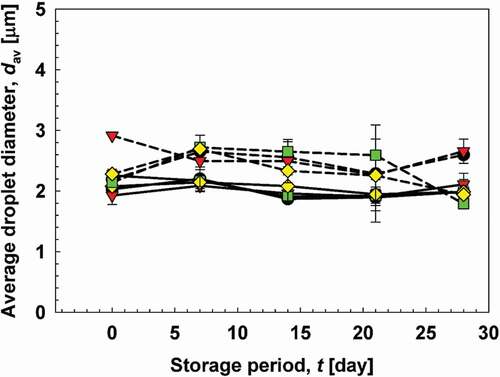
Based on the observations of various parameters described previously, there are a few hypotheses that can be proposed describing the stability phenomena of clove oil in chitosan or alginate matrix. High positive or negative charges of the polymers ensure repulsive interactions in the system that forces the emulsion droplets to be repel each other. Further, the stability of the emulsion droplets could be due to the high viscosity of the system attributed by chitosan and sodium alginate. However, there was still the possibility of droplet flocculation in the highly viscous system. The flocculation may be governed by the tendency of clove oil within the emulsion droplets to diffuse into the aqueous phase due to its water solubility properties.[Citation63] This mechanism is slightly different from the instability mechanism of the clove oil-in-water emulsion droplets under dilute conditions. In dilute emulsions, clove oil-in-water emulsion instability is suggested by diffusion and spontaneous emulsification (the diffused oil from large emulsion droplets spontaneously forms smaller droplets).[Citation54] To find the balance between stability-instability of clove oil in chitosan and sodium alginate matrix, further studies need to be performed.
Active compounds of clove oil in the continuous phase
Nineteen compounds were identified in clove oil, shown in . When clove oil was formulated in emulsions and the emulsions were stored, there were only three compounds that were detected in the continuous phase of the emulsions. Eugenol, caryophyllen, and humulene were detected in the continuous phase of CC and CA emulsions during storage. These compounds were quantified using Eq. (3). In CC emulsions, 71.1–87.9% of eugenol, 9.7–23.2% caryophyllene, and 2.3–5.9% humulene were detected in the continuous phase. No pattern of the concentration of each compound with increasing homogenization speed or during storage was observed. These compounds had the highest concentrations of all the compounds found in clove oil (). The presence of eugenol in the continuous phase may be the result of solubilisation, sequestering at the oil-water interface, or both.[Citation46] Purwanti et al. have also reported clove oil solubilizing into the continuous phase.[Citation56]
Concentrations of eugenol, caryophyllene, and humulene in the continuous phase of CA emulsions are similar to that in CC emulsions. However, a significantly lower concentration was measured for eugenol, and higher concentrations of caryophyllene and humulene, after 29 days of storage when the homogenization speeds were 10,000 rpm and greater. This could indicate a change in the clove oil quality when the emulsions were prepared at 10,000 rpm and greater speeds. The CA emulsions prepared at these speeds could sustain initial damage due to the temperature increasing significantly. The onset temperature for the boiling process of clove oil was 156.2°C, and the onset temperature of pure eugenol was 113.5°C.[Citation67] Further, the auto-oxidation process of free eugenol begins at 159°C.[Citation68] Nevertheless, current research demonstrates that eugenol in electrospun nanopolymer is unstable at temperatures of 50°C and greater.[Citation69] Having an ice-water mixture surrounding the processing chamber, the CA emulsions could reach 50–60°C after preparation, which did not occur in CC emulsions. The high temperature in CA emulsions is in agreement with McClements[Citation70], that is, most of the energy input at high homogenization speed is dissipated as heat. In this study, a higher temperature in CA emulsions could be attributed to the higher viscosity of sodium alginate, compared to that of chitosan. Dehydration and decomposition of sodium alginate occurs at approximately 100°C and 250°C, respectively.[Citation71] Moreover, temperatures up to 70°C were used to speed up the solubilisation process of sodium alginate.[Citation72] Therefore, modification of surface properties of sodium alginate due to excessive heating during homogenization at 10,000 rpm and greater did not cause instability of the system.
Conclusion
The emulsions of clove oil-in-chitosan matrix (CC) and clove oil-in-sodium alginate matrix (CA) were successfully prepared using a homogenization technique. The stability of the two prepared emulsion systems were different. Droplet size and concentration of clove oil compounds in the CC emulsions were stable during storage, regardless of the homogenization speed. However, phase separation of the phase was observed after 28 days of storage. This suggested that chitosan could be an appropriate material to encapsulate clove oil; however, all stages of encapsulation process, including drying, should be completed before the emulsion starts to destabilize. Results of this study suggested that the use of sodium alginate to encapsulate clove oil would not produce an emulsion system that was more stable than chitosan. The average size of the CA emulsion and concentration of eugenol decreased during storage, when a homogenization speed of more than 10,000 rpm was used to prepare the emulsions. Further, freshly made emulsions were physically unstable regardless the homogenization speed that was used. Therefore, chitosan would be the preferred material to encapsulate clove oil, compared to sodium alginate.
Acknowledgments
This research work was supported by Kirin Holdings Co., Ltd., Tokyo, under the scheme of UNU-Kirin Follow-up Research Program 2014-2016. The authors also thank PT Metrohm Indonesia for supporting Rheometer Anton Paar MCR301.
Additional information
Funding
References
- Chaieb, K.; Hajlaoui, H.; Zmantar, T.; Kahla-Nakhbi, A. B.; Rouabhia, M.; Mahdouani, K.; Bakhrouf, A. The Chemical Composition and Biological Activity of Clove Essential Oil, Eugenia Caryophyllata (Syzigium Aromaticum L. Myrtaceae): A Short Review. Phytotherapy Research 2007, 21, 501–506. DOI: 10.1002/ptr.2124.
- Kegley, S.; Conlisk, E.; Moses, M. Clove Oil (Eugenol) in Marin Municipal Water District: Herbicide Risk Assessment Ch. 6; Pesticide Research Institute: California, January 1 2010 2010; pp. 1–47.
- Milind, P.; Deepa, K.Clove: A Champion Spice. International Journal of Research Ayurveda & Pharmacy 2011, 2, 47–54.
- Wenqiang, G.; Shufen, L.; Ruixiang, Y.; Shaokun, T.; Can, Q. Comparison of Essential Oils of Clove Buds Extracted with Supercritical Carbon Dioxide and Other Three Traditional Extraction Methods. Food Chemistry 2007, 101, 1558–1564. DOI: 10.1016/j.foodchem.2006.04.009.
- Jin, S.; Cho, K.-H. Water Extracts of Cinnamon and Clove Exhibits Potent Inhibition of Protein Glycation and Anti-Atherosclerotic Activity in Vitro and in Vivo Hypolipidemic Activity in Zebrafish. Food and Chemical Toxicology 2011, 49, 1521–1529. DOI: 10.1016/j.fct.2011.03.043.
- Misharina, T. A.; Samusenko, A. L.Antioxidant Properties of Essential Oils from Lemon, Grapefruit, Coriander, Clove, and Their Mixtures. Prikladnaya Biokhimiya Mikrobiologiya 2008, 44, 482–486.
- Yoshimura, M.; Amakura, Y.; Yoshida, T. Polyphenolic Compounds in Clove and Pimento and Their Antioxidative Activities. Bioscience Biotechnology and Biochemistry 2011, 75, 2207–2212. DOI: 10.1271/bbb.110491.
- Kim, I.-S.; Yang, M.-R.; Lee, O.-H.; Kang, S.-N. Antioxidant Activities of Hot Water Extracts from Various Spices. International Journal of Molecular Sciences 2011, 12, 4120–4131. DOI: 10.3390/ijms12064120.
- Wang, X.; Jiang, Y.; Wang, Y. W.; Huang, M. T.; Ho, C. T.; Huang, Q. Enhancing Anti-Inflammation Activity of Curcumin through O/W Nanoemulsions. Food Chemistry 2008, 108, 419–424. DOI: 10.1016/j.foodchem.2007.10.086.
- Singh, A. K.; Dhamanigi, S. S.; Asad, M. Anti-Stress Activity of Hydro-Alcoholic Extract of Eugenia Caryophyllus Buds (Clove). Indian Journal of Pharmacology 2009, 41, 28–31. DOI: 10.4103/0253-7613.48889.
- Keskin, D.; Toroglu, S.Studies on Antimicrobial Activities of Solvent Extracts of Different Species. Journal of Environmental Biology 2011, 32, 251–256.
- Cui, H.; Zhao, X.; Lin, L. The Specific Antibacterial Activity of Liposome-Encapsulated Clove Oil and Its Application in Tofu. Food Control 2015, 56, 128–134. DOI: 10.1016/j.foodcont.2015.03.026.
- Anwer, M. K.; Jamil, S.; Ibnouf, E. O.; Shakeel, F. Enhanced Antibacterial Effects of Clove Essential Oil by Nanoemulsion. Journal of Oleo Science 2014, 63, 347–354. DOI: 10.5650/jos.ess13213.
- Xiao, J. X.; Yu, H. Y.; Yang, J. Microencapsulation of Sweet Orange Oil by Complex Coacervation with Soybean Protein Isolate/Gum Arabic. Food Chemistry 2011, 125, 1267–1272. DOI: 10.1016/j.foodchem.2010.10.063.
- Authority, E. F. S.;.Conclusion on the Peer Review of the Pesticide Risk Assessment of the Active Substance of Clove Oil. European Food Safety Authority Journal 2012, 10, 2506–2549.
- Cortés-Rojas, D. F.; Souza, C. R. F.; Oliveira, W. P. Encapsulation of Eugenol Rich Clove Extract in Solid Lipid Carriers. Journal of Food Engineering 2014, 127, 34–42. DOI: 10.1016/j.jfoodeng.2013.11.027.
- Rana, I. S.; Rana, A. S.; Rajak, R. C. Evaluation of Antifungal Activity in Essential Oil of the Syzygium Aromaticum (L.) By Extraction, Purification and Analysis of Its Main Component Eugenol. Brazilian Journal of Microbiology 2011, 42, 1269–1277. DOI: 10.1590/S1517-83822011000400004.
- Park, M.-J.; Gwak, K.-S.; Yang, I.; Choi, W.-S.; Jo, H.-J.; Chang, J.-W.; Jeung, E.-B.; Choi, I.-G.Antifungal Activities of the Essential Oils in Syzygium Aromaticum (L.) Merr. Et Perry and Leptospermum Petersonii Bailey and Their Constituents against Various Dermatophytes. The Journal of Microbiology 2007, 45, 460–465.
- Chatterjee, D.; Bhattacharjee, P. Comparative Evaluation of the Antioxidant Efficacy of Encapsulated and Un-Encapsulated Eugenol-Rich Clove Extracts in Soybean Oil: Shelf-Life and Frying Stability of Soybean Oil. Journal of Food Engineering 2013, 117, 545–550. DOI: 10.1016/j.jfoodeng.2012.11.016.
- Lin, L.; Cui, H.; Zhou, H.; Zhang, X.; Bortolini, C.; Chen, M.; Liu, L.; Dong, M. Nanoliposomes Containing Eucalyptus Citriodora as Antibiotic with Specific Antimicrobial Activity. Chemistry Communications 2015, 51, 2653–2655. DOI: 10.1039/C4CC09386K.
- Hill, L. E.; Gomes, C.; Taylor, T. M. Characterization of Beta-Cyclodextrin Inclusion Complexes Containing Essential Oils (Trans-Cinnamaldehyde, Eugenol, Cinnamon Bark, and Clove Bud Extracts) for Antimicrobial Delivery Applications. LWT - Food Science and Technology 2013, 51, 86–93. DOI: 10.1016/j.lwt.2012.11.011.
- Majeed, H.; Liu, F.; Hategekimana, J.; Sharif, H. R.; Qi, J.; Ali, B.; Bian, -Y.-Y.; Ma, J.; Yokoyama, W.; Zhong, F. Bactericidal Action Mechanism of Negatively Charged Food Grade Clove Oil Nanoemulsions. Food Chemistry 2016, 197, 75–83. DOI: 10.1016/j.foodchem.2015.10.015.
- Ghosh, V.; Mukherjee, A.; Chandrasekaran, N. Eugenol-Loaded Antimicrobial Nanoemulsion Preserves Fruit Juice Against, Microbial Spoilage. Colloids and Surfaces B: Biointerfaces 2014, 114, 392–397. DOI: 10.1016/j.colsurfb.2013.10.034.
- Cui, H.; Zhou, H.; Lin, L. The Specific Antibacterial Effect of the Salvia Oil Nanoliposomes against Staphylococcus Aureus Biofilms on Milk Container. Food Control 2016, 61, 92–98. DOI: 10.1016/j.foodcont.2015.09.034.
- Gharsallaoui, A.; Roudaut, G.; Chambin, O.; Voilley, A.; Saurel, R. Applications of Spray-Drying in Microencapsulation of Food Ingredients: An Overview. Food Research International 2007, 40, 1107–1121. DOI: 10.1016/j.foodres.2007.07.004.
- Mokhtari, S.; Jafari, S. M.; Assadpour, E. Development of a Nutraceutical Nano-Delivery System through Emulsification/Internal Gelation of Alginate. Food Chemistry 2017, 229, 286–295. DOI: 10.1016/j.foodchem.2017.02.071.
- Ye, S.; Wang, C.; Liu, X.; Tong, Z. Deposition Temperature Effect on Release Rate of Indomethacin Microcrystals from Microcapsules of Layer-By-Layer Assembled Chitosan and Alginate Multilayer Films. Journal of Controlled Release 2005, 106, 319–328. DOI: 10.1016/j.jconrel.2005.05.006.
- Chávarri, M.; Marañón, I.; Ares, R.; Ibáñez, F. C.; Marzo, F.; del Carmen Villarán, M. Microencapsulation of a Probiotic and Prebiotic in Alginate-Chitosan Capsules Improves Survival in Simulated Gastro-Intestinal Conditions. International journal of food microbiology 2010, 142, 185–189. DOI: 10.1016/j.ijfoodmicro.2010.06.022.
- Shinde, U.; Nagarsenker, M.Microencapsulation of Eugenol by Gelatin-Sodium Alginate Complex Coacervation. Indian journal of pharmaceutical sciences 2011, 73, 311–315.
- Soliman, E. A.; El-Moghazy, A. Y.; El-Din, M. M.; Massoud, M. A. Microencapsulation of Essential Oils within Alginate: Formulation and in Vitro Evaluation of Antifungal Activity. Journal of Encapsulation and Adsorption Sciences 2013, 3, 48–55. DOI: 10.4236/jeas.2013.31006.
- Lee, K. Y.; Mooney, D. J. Alginate: Properties and Biomedical Applications. Progress in polymer science 2012, 37, 106–126. DOI: 10.1016/j.progpolymsci.2011.06.003.
- Najafi-Soulari, S.; Shekarchizadeh, H.; Kadivar, M. Encapsulation Optimization of Lemon Balm Antioxidants in Calcium Alginate Hydrogels. Journal of Biomaterials Science, Polymer Edition 2016, 27, 1631–1644. DOI: 10.1080/09205063.2016.1226042.
- Chan, E.-S.;. Preparation of Ca-Alginate Beads Containing High Oil Content: Influence of Process Variables on Encapsulation Efficiency and Bead Properties. Carbohydrate Polymers 2011, 84, 1267–1275. DOI: 10.1016/j.carbpol.2011.01.015.
- Pedro, A. S.; Cabral-Albuquerque, E.; Ferreira, D.; Sarmento, B. Chitosan: An Option for Development of Essential Oil Delivery Systems for Oral Cavity Care? Carbohydrate Polymers 2009, 76, 501–508. DOI: 10.1016/j.carbpol.2008.12.016.
- Kumar, M. N. R.;. A Review of Chitin and Chitosan Applications. Reactive and functional polymers 2000, 46, 1–27. DOI: 10.1016/S1381-5148(00)00038-9.
- Dutta, P. K.; Tripathi, S.; Mehrotra, G. K.; Dutta, J. Perspectives for Chitosan Based Antimicrobial Films in Food Applications. Food Chemistry 2009, 114, 1173–1182. DOI: 10.1016/j.foodchem.2008.11.047.
- Ratajska, M.; Strobin, G.; Wiśniewska-Wrona, M.; Ciechańska, D.; Struszczyk, H.; Boryniec, S.; Biniaś, D.; Biniaś, W.Studies on the Biodegradation of Chitosan in an Aqueous Medium. Fibres & Textiles in Eastern Europe 2003, 11, 75–79.
- Kwon, H.-Y.; Lee, J.-Y.; Choi, S.-W.; Jang, Y.; Kim, J.-H. Preparation of PLGA Nanoparticles Containing Estrogen by Emulsification–Diffusion Method. Colloids and Surfaces A: Physicochemical and Engineering Aspects 2001, 182, 123–130. DOI: 10.1016/S0927-7757(00)00825-6.
- Hsieh, W.-C.; Chang, C.-P.; Gao, Y.-L. Controlled Release Properties of Chitosan Encapsulated Volatile Citronella Oil Microcapsules by Thermal Treatments. Colloids and Surfaces B: Biointerfaces 2006, 53, 209–214. DOI: 10.1016/j.colsurfb.2006.09.008.
- Bonilla, J.; Vargas, M.; Atarés, L.; Chiralt, A. Physical Properties of Chitosan-Basil Essential Oil Edible Films as Affected by Oil Content and Homogenization Conditions. Procedia Food Science 2011, 1, 50–56. DOI: 10.1016/j.profoo.2011.09.009.
- Keawchaoon, L.; Yoksan, R. Preparation, Characterization and in Vitro Release Study of Carvacrol-Loaded Chitosan Nanoparticles. Colloids and Surfaces B: Biointerfaces 2011, 84, 163–171. DOI: 10.1016/j.colsurfb.2010.12.031.
- Hosseini, S. F.; Zandib, M.; Rezaeia, M.; Farahmandghavic, F. Two-Step Method for Encapsulation of Oregano Essential Oil in Chitosan Nanoparticles: Preparation, Characterization and in Vitro Release Study. Carbohydrate Polymers 2013, 95, 50–56. DOI: 10.1016/j.carbpol.2013.02.031.
- Martínez-Hernández, G. B.; Amodio, M. L.; Colelli, G. Carvacrol-Loaded Chitosan Nanoparticles Maintain Quality of Fresh-Cut Carrots. Innovative Food Science and Emerging Technologies 2017, 41, 56–63. DOI: 10.1016/j.ifset.2017.02.005.
- Ghaderi-Ghahfarokhi, M.; Barzegar, M.; Sahari, M. A.; Gavlighi, H. A.; Gardini, F. Chitosan-Cinnamon Essential Oil Nano-Formulation: Application as a Novel Additive for Controlled Release and Shelf Life Extension of Beef Patties. International Journal of Biological Macromolecules 2017, 102, 19–28. DOI: 10.1016/j.ijbiomac.2017.04.002.
- Sotelo-Boy´as, M.; Correa-Pacheco, Z.; Bautista-Ba˜nos, S.; G´Omez, Y. Release Study and Inhibitory Activity of Thyme Essential Oil-Loaded Chitosan Nanoparticles and Nanocapsules against Foodborne Bacteria. International Journal of Biological Macromolecules 2017, 103, 409–414. DOI: 10.1016/j.ijbiomac.2017.05.063.
- Terjung, N.; Löffler, M.; Gibis, M.; Hinrichs, J.; Weiss, J. Influence of Droplet Size on the Efficacy of Oil-In-Water Emulsions Loaded with Phenolic Antimicrobials. Food & function 2012, 3, 290–301. DOI: 10.1039/C2FO10198J.
- Li, R.; Deng, L.; Cai, Z.; Zhang, S.; Wang, K.; Li, L.; Ding, S.; Zhou, C. Liposomes Coated with Thiolated Chitosan as Drug Carriers of Curcumin. Materials Science and Engineering C 2017, 80, 156–164. DOI: 10.1016/j.msec.2017.05.136.
- Turasan, H.; Sahin, S.; Sumnu, G. Encapsulation of Rosemary Essential Oil. LWT - Food Science and Technology 2015, 64, 112–119. DOI: 10.1016/j.lwt.2015.05.036.
- Sapei, L.; Naqvi, M. A.; Rousseau, D. Stability and Release Properties of Double Emulsions for Food Applications. Food Hydrocolloids 2012, 27, 316–323. DOI: 10.1016/j.foodhyd.2011.10.008.
- Hossain, M. A.; Al-Hashmi, R. A.; Weli, A. M.; Al-Riyami, Q.; Al-Sabahib, J. N. Constituents of the Essential Oil from Different Brands of Syzigium Caryophyllatum L by Gas Chromatography-Mass Spectrometry. Asian Pacific Journal of Tropical Biomedicine 2012, 2, S1446–S1449. DOI: 10.1016/S2221-1691(12)60435-3.
- Bhuiyan, M. N. I.;.Constituents of the Essential Oil from Leaves and Buds of Clove (Syzigium Caryophyllatum (L.) Alston). African Journal of Pharmacy and Pharmacology 2012, 6, 1260–1263.
- Alma, M. H.; Ertaş, M.; Nitz, S.; Kollmannsberger, H.Chemical Composition and Content of Essential Oil from the Bud of Cultivated Turkish Clove (Syzygium Aromaticum L.). Bioresources 2007, 2, 265–269.
- Nassar, M. I.; Gaara, A. H.; El-Ghorab, A. H.; Farrag, A.-R. H.; Shen, H.; Huq, E.; Mabry, T. J.Chemical Constituents of Clove (Syzygium Aromaticum, Fam. Myrtaceae) and Their Antioxidant Activity. Revista Latinoamericana de Química 2007, 35, 47–57.
- Purwanti, N.; Neves, M. A.; Uemura, K.; Nakajima, M.; Kobayashi, I. Stability of Monodisperse Clove Oil Droplets Prepared by Microchannel Emulsification. Colloids and Surfaces A: Physicochemical and Engineering Aspects 2015, 466, 66–74. DOI: 10.1016/j.colsurfa.2014.10.058.
- Ma, J.; Lin, Y.; Chen, X.; Zhao, B.; Zhang, J. Flow Behavior, Thixotropy and Dynamical Viscoelasticity of Sodium Alginate Aqueous Solutions. Food Hydrocolloids 2014, 38, 119–128. DOI: 10.1016/j.foodhyd.2013.11.016.
- Mancini, M.; Moresi, M.; Sappino, F. Rheological Behaviour of Aqueous Dispersions of Algal Sodium Alginates. Journal of Food Engineering 1996, 28, 283–295. DOI: 10.1016/0260-8774(95)00068-2.
- Shepherd, R.; Reader, S.; Falshaw, A. Chitosan Functional Properties. Glycoconjugate Journal 1997, 14, 535–542. DOI: 10.1023/A:1018524207224.
- Hunter, R. J.;. Zeta Potential in Colloid Science: Principles and Applications; Academic Press London, London, 1981; p. 386.
- Riddick, T. M.;. Control of Colloidal Stability through Zeta Potential; Livingston Pub. Co, New York, 1968.
- Vladisavljević, G. T.; Lambrich, U.; Nakajima, M.; Schubert, H. Production of O/W Emulsions Using SPG Membrane, Ceramic α-Al2O3 Membranes, Microfluidizer and a Microchannel Plate - a Comparative Study. Colloids and Surfaces A 2004, 232, 199–207. DOI: 10.1016/j.colsurfa.2003.10.026.
- Vladisavljević, G. T.; Kobayashi, I.; Nakajima, M. Production of Uniform Droplets Using Membrane, Microchannel and Microfluidic Emulsification Devices. Microfluid Nanofluid 2012, 13, 151–178. DOI: 10.1007/s10404-012-0948-0.
- Khalid, N.; Kobayashi, I.; Neves, M. A.; Uemura, K.; Nakajima, M. Preparation and Characterization of Water-In-Oil Emulsions Loaded with High Concentration of L-Ascorbic Acid. LWT - Food Science and Technology 2013, 51, 448–454. DOI: 10.1016/j.lwt.2012.11.020.
- Purwanti, N.; Ichikawa, S.; Neves, M. A.; Uemura, K.; Nakajima, M.; Kobayashi, I. β-lactoglobulin as Food Grade Surfactant for Clove Oil-In-Water and Limonene-In-Water Emulsion Droplets Produced by Microchannel Emulsification. Food Hydrocolloids 2016, 60, 98–108. DOI: 10.1016/j.foodhyd.2016.03.024.
- Lucassen-Reynders, E. H.; Kuijpers, K. A. The Role of Interfacial Prperties in Emulsification. Colloids and Surfaces 1992, 65, 175–184. DOI: 10.1016/0166-6622(92)80272-4.
- Peng, L.; Yang, M.-R.; Guo, -S.-S.; Liu, W.; Zhao, X.-Z. The Effect of Interfacial Tension on Droplet Formation in Flow-Focusing Microfluidic Device. Biomedical Microdevices 2011, 13, 559–564. DOI: 10.1007/s10544-011-9526-6.
- Ho, C. C.; Chow, M. C. The Effect of the Refining Process on the Interfacial Properties of Palm Oil. Journal of the American Oil Chemists’ Society 2000, 77, 191–199. DOI: 10.1007/s11746-000-0031-7.
- Santos, A. L.; Chierice, G. O.; Alexander, K. S.; Riga, A.; Matthews, E. Characterization of the Raw Essential Oil Eugeno Extracted from Syzygium Aromaticum L. Journal of Thermal Analysis and Calorimetry 2009, 96, 821–825. DOI: 10.1007/s10973-009-0030-7.
- Woranuch, S.; Yoksan, R. Eugenol-Loaded Chitosan Nanoparticles: I. Thermal Stability Improvement of Eugenol through Encapsulation. Carbohydrate Polymers 2013, 96, 578–585. DOI: 10.1016/j.carbpol.2012.08.117.
- Kayaci, F.; Ertas, Y.; Uyar, T. Enhanced Thermal Stability of Eugenol by Cyclodextrin Inclusion Complex Encapsulated in Electrospun Polymeric Nanofibers. Journal of Agricultural and Food Chemistry 2013, 61, 8156–8165. DOI: 10.1021/jf402923c.
- McClements, D.;. Food Emulsions: Principles, Practices and Techniques; CRC Press: Boca Raton, Florida, 2005.
- Soares, J. P.; Santos, J. E.; Chierice, G. O.; Cavalheiro, E. T. G. Thermal Behavior of Alginic Acid and Its Sodium Salt. Eclética Química 2004, 29, 57–64. DOI: 10.1590/S0100-46702004000200009.
- Fioramonti, S. A.; Perez, A. A.; Aríngoli, E. E.; Rubiolo, A. C.; Santiago, L. G. Design and Characterization of Soluble Biopolymer Complexes Produced by Electrostatic Self-Assembly of a Whey Protein Isolate and Sodium Alginate. Food Hydrocolloids 2014, 35, 129–136. DOI: 10.1016/j.foodhyd.2013.05.001.