ABSTRACT
Hypertension represents one of the most common maladies of western civilizations caused by bad dietary practices and lack of exercise. Angiotensin-I-converting enzyme inhibitors (ACEi) have been shown to be very efficient in the prevention of hypertension. ACEi are also implicated (by their ability to reduce the product of ACE activity namely angiotensin-II levels, which has oncogenic and increased cell proliferation effects) to be efficient anticancer agents. Therefore, we evaluated the potential antihypertension capacities of anacardic acids purified from Cashew (Anacardium occidentale Linn.) nut shell liquid by inhibition of ACE using analytical reverse-phase high performance liquid chromatography. The ability to inhibit ACE showed the following relation: anacardic-3 (IC50 = 12 µM) > anacardic acid-4 (IC50 = 20 µM) > anacardic acid-2 (IC50 = 33 µM) > anacardic acid-1 (IC50 = 39 µM) as compared to the positive control captopril (IC50 = 6.85 nM). Salicylic acid lacking a phytyl side chain was negative. The capacity of purified anacardic acids to inhibit ACE thereby represents the most potent naturally available plant chemicals described so far, as a potential means of reducing hypertension. The inhibition of angiotensin II production indicated that anacardic acids could also have utility in cancer prevention and recurrence, and reduction of cardiotoxicity during chemotherapy.
Introduction
Hypertension is a major public health problem caused by an unhealthy diet, lack of physical exercise and obesity. Inhibition of angiotensin converting enzyme (ACE) has been identified as a main therapeutic target in controlling high blood pressure. Angiotensin II (Ang II), the biologically active peptide of the renin–angiotensin system (RAS), is a major regulator of blood pressure and cardiovascular homeostasis, and is also recognized as a potent mitogen. Ang II is produced by cleavage of the inactive decapeptide Angiotensin I (Ang I) by ACE detected in the circulation or bound to the cell membrane. Ang I itself is produced by enzymatic cleavage of the angiotensinogen precursor by renin. In addition, production of plasma Ang II, a local RAS has been shown to be functional in various organs, leading to production of Ang II that may have a paracrine or autocrine function.
Ang II is implicated in cardivascular diseases, and is associated with a role in increasing vascular inflammation.[Citation1] Administration of ACE inhibitors, results in an increase of both antioxidant enzymes, and decreased levels of lipid peroxidation, whereas angiotensin II elevates oxidative stress status as judged by increased levels of lipid peroxidation, and a decrease in the antioxidant enzymes, superoxide dismutase and glutathione peroxidase.[Citation2]
ACE inhibitors (ACEi) have been used successfully, as potent antihypertension drugs over the past 20 years, and Lever et al.[Citation3] also proferred the provocative hypothesis that ACEi may have a protective role against cancer. This was supported by the data of Herr et al.,[Citation4] who observed a reduced incidence of breast and lung cancer in patients undergoing long-term treatment with the ACEi captopril, lisinopril, or enalapril. Radin et al.[Citation5] also implied that these compounds may be effective in anticancer therapy. Of significance is that Raimandi et al.[Citation6] recently showed in a meta-analysis that ACE inhibitor therapy no effects with regards to breast cancer specific survival. The potential of plant secondary metabolites as a source of new ACE inhibitors remains underexploited, although the extracts of a large number of plant species and some individual compounds have been screened for this target.[Citation7,Citation8] Anacardium occidentale Linn. (Cashew), a member of the family Anacardiaceae, is a tropical tree indigenous to Brazil, which is now also extensively cultivated in India and east Africa. India is the largest producer of Cashew nut, accounting for almost 50% of global exports. Cashew nut shell liquid (CNSL) is an important by-product of Cashew nut production.[Citation9] The potential annual availability of this material, which accounts for about 32% of the shell, is enormous. The biologic activities of CNSL components, specially the anacardic acids, have attracted considerable attention, in the areas of, for example, inhibition of α-glucosidase, invertase and aldose reductase,[Citation10] inhibition of tyrosinase,[Citation11] uncoupling effects on liver mitochondria, [Citation12] antioxidant activity, as well as xanthine oxidase inhibition.[Citation9] Anacardic acids also exhibit antitumor activities, by blockade of numerous enzymes and molecules, especially histone acetyltransferase[Citation13,Citation14] and modulate NF-KB signaling.[Citation15] Further reports demonstrate that anacardic acid can sensitize tumor cells to ionizing radiation in cancer therapy[Citation16] and inhibits protein SUMOylation by blocking formation of the E1-Small Ubiquitin-like Modifier (SUMO) intermediate.[Citation17] Schultz et al.[Citation18] showed that anacardic acid-3 inhibits cell proliferation, cell cycle progression, and apoptosis in an estrogen receptor–dependent manner by reducing ER-DNA interaction and inhibiting ER-mediated transcriptional responses. Carvalho et al.[Citation19] also demonstrated that supplementation with anacardic acids has a protective role against oxidative and inflammatory mechanisms in the lungs of mice exposed to diesel exhaust, and there is also a patent from Yi et al.,[Citation20] claiming their use as angiogenesis inhibitors for prevention and treatment of neovascularization related diseases, such as tumors, arthritis, psoriasis, and eye disease.
In this study, alkyl phenols from CNSL were analyzed. Anacardic acids, cardols, and cardanols were fractionated as classes, and the individual anacardic acids were purified to homogeneity, for measurement of ACE inhibition capacity in comparison to captopril, a known potent ACE inhibitor.
Materials and methods
Chemicals and reagents
ACE, calcium hydroxide, captopril, ethyl acetate, trifluoroacetic acid (TFA), HCl, hippuric acid, N-hippuryl-L-histidyl-L-leucine (HHL), sodium chloride, and Trizma base were obtained from Sigma-Aldrich (Deisenhofen, Germany); acetonitrile from Fluka/Riedel de Haen (Seelze, Germany); acetic acid, DMSO, n-hexane, and salicylic acid from Merck (Darmstadt, Germany); while ellagic acid, maclurin, mangiferin, luteolin, and taxifolin were obtained from Extrasynthese (Lyon Nord, Genay, France). Pentagallate was purified from Mango seed extracts as described by Barreto et al. (2008). All solutions were made up in either doubly distilled water, DMSO, or methanol.
Cashew nut shell liquid
The CNSL used in this study was a kind gift from Luquesio Petrola de Melo Jorge (Beraba LTDA).
Isolation of phenolic antioxidants from CNSL
Separation of anacardic acids, cardanols, and cardols from CNSL (10 g) was conducted as described by Paramashivappa et al.[Citation21] Briefly, anacardic acids were isolated as their calcium salts, and the acid-free CNSL was treated with ammonia, and extracted with hexane/ethyl acetate (98:2) to separate the mono-phenolic cardanol components, followed by ethyl acetate/hexane (80:20) extraction to yield the cardols.
High-performance liquid chomatography electrospray ionization mass spectrometry (HPLC-ESI-MS)
HPLC-ESI-MS was conducted on an Agilent 1100 HPLC, coupled to an Agilent single quadrupole mass-selective detector (HP 1101; Agilent Technologies, Waldbronn, Germany). Chromatographic separation of solutions in methanol (10 µL) was conducted using a C18, reverse-phase (5 µm), column (250 × 4.6 mm2 I.D.; Latek, Eppelheim, Germany). For separation of the anacardic acids, the mobile phase (1.0 mL/min) consisted of 2% acetic acid in water (solvent A) and acetonitrile (solvent B) with the following gradient: 50% A:50% B to 100% B in 20 min. and continuing at 100% B for 10 min. until completion of the run. Detection of phenolic compounds was by means of UV absorbance (A) at 257, 278, and 340 nm at room temperature. Mass spectra in negative-ion mode were generated under the following conditions: fragmenter voltage = 100 V, capillary voltage = 2500 V, nebulizer pressure = 30 psi, drying gas temperature = 350°C, mass range = 100–1500 D.
For the separation of hippuric acid from HHL by analytical HPLC, a similar column was used, and the mobile phase (1.0 mL/minute) consisted of 0.05% TFA in deionized water (solvent A) and 0.05% TFA in acetonitrile (solvent B) with the following gradient: 95% A to 60% B in 10 min, maintained for 2 min. and, returned to 5% B in 1 min. with equilibration for 5 min. Detection was by UV absorbance (A) at 228 nm. Instrument control and data handling were performed with the HP Chemstation software on a PC.
Semi-preparative HPLC and fraction collection
Semi-preparative HPLC was conducted on an Agilent 1100 liquid chromatograph fitted with a C18, reverse-phase (5 µm), column (250 × 10 mm2 I.D.; Latek, Eppelheim, Germany). For separation of individual anacardic acids, the mobile phase consisted of acetonitrile–water–acetic acid (80:20:1) run in isocratic mode (Shoba & Ravindranath, 1991) at a flow rate of 3.0 mL/min. The alkyl phenols in the eluant were detected with a UV diode-array detector (HP 1040M) set at 278 and 315 nm. Peaks eluting from the column were collected on a HP 220 Microplate Sampler, pooled and subsequently freeze-dried (Model alpha 1–4 LSE, Christ, Osterode, Germay).
Gas chromatography–mass spectrometry (GC-MS)
Analyses were performed exactly as described by Trevisan et al.[Citation9]
Electrospray ionization mass spectrometry (ESI-MS)
Analyses were performed exactly as described by Trevisan et al.[Citation9]
Angiotensin-I converting enzyme assay
The substrate HHL (5 mM–2.15 mg/mL) was dissolved in 50 mM Tris-HCl (pH 8.3) containing 0.3 M NaCl. Inhibitor solutions (25 µL dissolved in methanol) were added to the substrate solution (100 µL) and incubated at 37°C for 10 min. ACE solution (200 milliunits/mL, 50 µL) in 50 mM Tris-HCl, pH 8.3 containing 0.3 M NaCl (50 µL) was added and incubation continued at 37°C for 30 min with continuous agitation at 450 rpm. The reaction was terminated by addition of 1 M HCl (100 µL). Captopril was used as positive control and methanol was used as negative control. Following incubation the samples were analyzed by analytical reverse-phase HPLC. Initially, the mixtures and pure compounds were screened at a concentration of 1.0 mg/mL. When appreciable inhibition (>50%) of ACE was detected, they were then screened in the range 0–500 µM to ascertain the IC50. Of these only the anacardic acids were deemed sufficiently potent with regards to ACE inhibition and therefore were used to study the kinetics of the inhibition mechanism in the range 10–80 µM with variable concentrations of substrate (0.25–5.0 mM).
Statistics
The concentration of mixtures and purified phenolic compounds producing a 50% inhibition of oxidation (IC50) were determined using the Table Curve Program (Jandel Scientific, Chicago, IL).
Results and discussion
The capacity of anacardic acids isolated from CNSL and purified by semipreparative HPLC to act as ACEi was evaluated by a reverse-phase HPLC-based assay. Isolation and identification of anacardic acids in CNSL was similar to that described by Trevisan et al. [Citation9]
Inhibition of ACE by anacardic acids
CNSL realized an IC50 = 50 µM as determined in the ACE inhibition assay, and of its phenolic components () a mixture of anacardic acids showed by far the higher inhibition of ACE (IC50 = 18 µM) compared to cardanols and cardols (>500 µM) (). Further tests () on the purified compounds showed the following relation. Anacardic-3 (IC50 = 12 µM) > anacardic acid-4 (IC50 = 20 µM) > anacardic acid-2 (IC50 = 33 µM) > anacardic acid-1 (IC50 = 39 µM) as compared to the clinically used positive control captopril (IC50 = 6.85 nM). Additional phenolic compounds (), such as ellagic acid, pentagallate, maclurin, mangiferin, and luteolin were weakly positive with IC50 values between 500 µM and 1.0 mM, whereas salicylic acid and taxifolin were negative.
Figure 1. Structures of the alkyl phenols detected in Cashew nut shell liquid. I = anacardic acid-1, II = anacardic acid-2, III = anacardic acid-3, IV = anacardic acid-4, V = cardonol-1, VI = cardonol-2, VII = cardonol-3, VIII = cardol-1, IX = cardol-2.
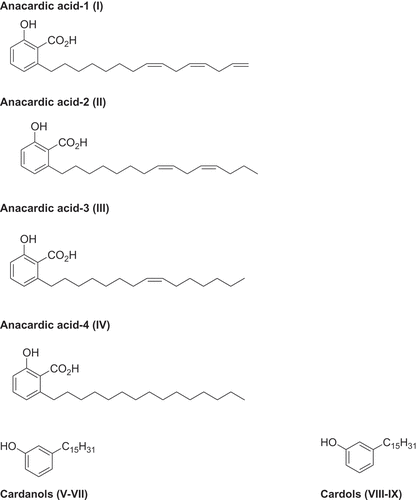
Table 1. Inhibition of Angiotensin I converting enzyme (ACE) by alkyl phenols, and anacardic acids purified to homogeneity by semi-preparative HPLC compared to selected reference and published data
In this assay anacardic acid-3 with one double bond in the phytyl side-chain was the more active (IC50 = 12 µM) as compared to anacardic acid-4 with no double bonds in the side-chain (IC50 = 20 µM). Anacardic acids-1 and -2 (three and two double bonds in the phytyl side chain respectively) with IC50 values of 33 and 39 µM, respectively, were not as potent with respect to anacardic acids-3 and -4, in contrast to that reported for free radical scavenging capacity and xanthine oxidase inhibition.[Citation9]
The kinetic data for the anacardic acids was entirely consistent with non-competitive inhibition as shown in and ). This is in contrast to that reported for flavan-3-ols and procyanidins where the mechanism of ACE inhibition was described as competitive.
Figure 2. Inhibition by different concentrations of anacardic acids of ACE activity as determined by analytical HPLC (a) and by anacardic acid-3 showing ± SEM (b).
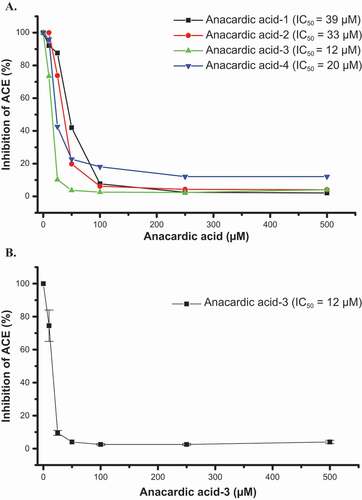
Figure 3. (b) Lineweaver Burk plot demonstrating non-competitive ACE inhibition by anacardic acid-1. Blue circle, control; red circle, anacardic acid-1 (40 µM).
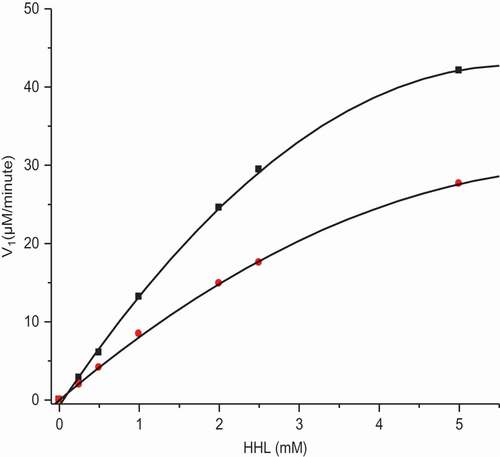
Figure 4. (a) Initial velocity of ACE inhibition by anacardic acid-1. Red circle, control; black square, anacardic acid-1 (40 µM).
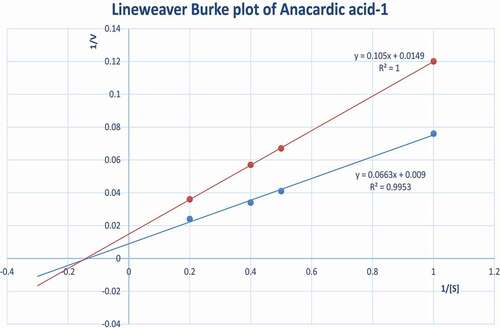
These data compare favorably with other published information[Citation7,Citation8] on secondary plant products and represent the lowest IC50 values for monophenols reported to date. The only other comparable data () is that of Actis-Goretta et al.,[Citation7] who reported similar values for procyanidin hexamers (IC50 = 10 µM), tetramers (IC50 = 12 µM), and pentamers (IC50 = 25 µM), whereas trimers (IC50 = 126 µM) and dimers (IC50 = 267 µM) and especially monomers, i.e. (–)-epicatechin (IC50 = 1781 µM) and (+)-catechin (IC50 = 1593 µM) were relatively inactive. Other less potent phytochemicals have been described[Citation22] namely rutin (IC50 = 71 µM) and cyanidin sambubioside and delphinidin sambubioside[Citation8] with IC50 values of 118 and 142 µM, respectively.
Salicylic acid, which is very similar in structure to the anacardic acids, was negative in the ACE inhibition assay, indicating the importance of the phytyl side-chain for blocking enzyme activity. The low inhibitory effects of cardols and cardonols lacking a carboxylic acid functional group indicates that this moiety may be a complementary group of the basic amino acid residue at the active site of ACE. Thus the hydrophobic tail and the carboxyl group are both important for ACE inhibitory activity. This has similarities to the mechanism described for the inhibition of xanthine oxidase[Citation9] and this is now under study in our laboratories.
It has also been reported that low molecular weight peptides[Citation23–Citation25] have IC50 values in the low µM range, but as for the anacardic acids bioavailability has yet to be established. Studies on bioavailability in preclinical models are therefore urgently required. The take home message here is that anacardic acids, major components present in Cashew products[Citation9] if taken in the diet, are likely to have health beneficial effects by lowering the risk of hypertension and maybe particularly useful as a replacement for generic antihypertension drugs in pregnant women, for whom ACE inhibitors are contraindicated due to the risk of fetal or neonatal renal failure and other complications.[Citation26] The ability of ACEi to reduce the generation of angiotensin II, the product of ACE, may have utility as oncolytic and oncopreventive measures in multiple types of cancer and also may prevent cardiotoxicity during chemotherapy. ACE inhibitors are approved by the FDA as a means to slow the growth of multiple cancer types.[Citation5]
Additional information
Funding
References
- Gan, W.; Ren, J.; Li, T.; Lv, S.; Li, C.; Liu, Z.; Yang, M. The SGK1 Inhibitor EMD638683, Prevents Angiotensin II–Induced Cardiac Inflammation and Fibrosis by Blocking NLRP3 Inflammasome Activation. BBA Molecular Basis of Disease 2018, 1864, 1–10. DOI: 10.1016/j.bbadis.2017.10.001.
- Bild, W.; Hritcu, L.; Stefanescu, C.; Ciobica, A. Inhibition of Central Angiotensin II Enhances Memory Function and Reduces Oxidative Stress Status in Rat Hippocampus. Progress in Neuro-Psychopharmacology & Biological Psychiatry 2013, 43, 79–88. DOI: 10.1016/j.pnpbp.2012.12.009.
- Lever, A. F.; Hole, D. J.; Gillis, C. R.; McCallum, I. R.; McInnes, G. T.; MacKinnon, P. L.;Meredith, P.A.; Murray, L.S.; Reid, J.L.; Robertson, J.W.K. Do Inhibitors of angiotensin-I-converting Enzyme Protect against Risk of Cancer? Lancet 1998, 352, 179–184. DOI: 10.1016/S0140-6736(98)03228-0.
- Herr, D.; Rodewald, M.; Fraser, H. M.; Hack, G.; Konrad, R.; Kreienberg, R.; Wulff, C. Potential Role of Renin-Angiotensin-system for Tumor Angiogenesis in Receptor Negative Breast Cancer. Gynecologic Oncology 2008, 109, 418–425. DOI: 10.1016/j.ygyno.2008.02.019.
- Radin, D. P.; Krebs, A.; Maqsudlu, A.; Patel, P. Our ACE in the HOLE. Anticancer Research 2018, 38, 45–49.
- Raimondi, S.; Botteri, E.; Munzone, E.; Cipolla, C.; Rotmensz, N.; DeCensi, A.; Gandini, S. Use of Beta-Blockers, Angiotensin-Converting Enzyme Inhibitors and Angiotensin Receptor Blockers and Breast Cancer Survival: Systematic Review and Meta-Analysis. International Journal of Cancer 2016, 139, 212–219. DOI: 10.1002/ijc.30062.
- Actis-Goretta, L.; Ottaviani, J. I.; Keen, C. L.; Fraga, C. G. Inhibition of Angiotensin Converting Enzyme (ACE) Activity by Flavan-3-Ols and Procyanidins. FEBS Letters 2003, 555, 597–600. DOI: 10.1016/S0014-5793(03)01355-3.
- Ojeda, D.; Jiménez-Ferrer, E.; Zamilpa, A.; Herrera-Arellano, A.; Tortoriello, J.; Alvarez, L. Inhibition of Angiotensin Convertin Enzyme (ACE) Activity by the Anthocyanins Delphinidin- and cyanidin-3-O-sambubiosides From. Journal of Ethnopharmacology 2010, 127, 7–10. DOI: 10.1016/j.jep.2009.09.059.
- Trevisan, M. T. S; Pfundstein, B.; Haubner, R.; Würtele, G.; Spiegelhalder, B.; Bartsch, H.; Owen, R.W. Characterization of Alkyl Phenols in Cashew (Anacardium occidentale) Products and Assay of Their Antioxidant Capacity. Food and Chemical Toxicology 2006, 44, 188–197. DOI: 10.1016/j.fct.2005.06.012.
- Toyomizu, M.; Sugiyama, S.; Jin, R. L.; Nakatsu, T. α-Glucosidase and Aldose Reductase Inhibitors: Constituents of Cashew, Anacardium occidentale, Nut Shell Liquid. Phytotherapy Research 1993, 7, 252–254. DOI: 10.1002/ptr.2650070309.
- Kubo, I.; Kinst-Hori, I.; Yokokawa, Y. Tyrosinase Inhibitors from Anacardium occidentale Fruits. Journal of Natural Products 1994, 57, 545–551. DOI: 10.1021/np50106a021.
- Toyomizu, M.; Okamoto, K.; Ishibashi, T.; Chen, Z.; Nakatsu, T. Uncoupling Effect of Anacardic Acids from Cashew Nut Shell Oil on Oxidative Phosphorylation of Rat Liver Mitochondria. Life Sciences 2000, 66, 229–234. DOI: 10.1016/S0024-3205(99)00585-8.
- Balasubramanyam, K.; Swaminathan, V.; Ranganathan, A.; Kundu, T. Small Molecule Modulators of Histone Acetyltransferase P300. Journal of Biological Chemistry 2003, 278, 19134–19140. DOI: 10.1074/jbc.M301580200.
- Mai, A.; Rotili, D.; Tarantino, D.; Ornaghi, P.; Tosi, F.; Vicidomini, C.; Sbardella, G.; Nebbioso, A.; Miceli, M.;Altucci, L.; Filetici, P. Small-Molecule Inhibitors of Histone Acetyltransferase Activity: Identification and Biological Properties. Journal of Medicinal Chemistry 2006 49, 6897–6907. DOI: 10.1021/jm060601m.
- Sung, B.; Pandey, M. K.; Ahn, K. S.; Yi, T.; Chaturvedi, M. M.; Liu, M.; Aggarwal, B. B. Anacardic Acid (6-Nonadecyl Salicylic Acid), an Inhibitor of Histone Acetyltransferase, Suppresses Expression of Nuclear factor-kappaB-regulated Gene Products Involved in Cell Survival, Proliferation, Invasion, and Inflammation through Inhibition of the Inhibitory Subunit of Nuclear factor-kappaB Alpha Kinase, Leading to Potentiation of Apoptosis. Blood 2008, 111, 4880–4891.
- Sun, Y.; Jiang, X.; Chen, S.; Price, B. D. Inhibition of Histone Acetyltransferase Activity by Anacardic Acid Sensitizes Tumor Cells to Ionizing Radiation. FEBS Journal 2006, 580, 4353–4356. DOI: 10.1016/j.febslet.2006.06.092.
- Fukuda, I.; Ito, A.; Hirai, G.; Nishimura, S.; Kawasaki, H.; Saitoh, H.; Kimura, K.; Sodeoka, M.; Yoshida, M. Ginkgolic Acid Inhibits Protein SUMOylation by Blocking Formation of the E1-SUMO Intermediate. Chemical Biology 2009, 16, 133–140. DOI: 10.1016/j.chembiol.2009.01.009.
- Schultz, D. J.; Wickramasinghe, N. S.; Ivanova, M. M.; Isaacs, S. M.; Dougherty, S. M.; Imbert-Fernandez, Y.;Cunningham, A.R.; Chen, C.; Klinge,C.M. Anacardic Acid Inhibits Estrogen Receptor α-DNA Binding and Reduces Target Gene Transcription and Breast Cancer Cell Proliferation. Molecular Cancer Therapeutics 2010, 9, 594–605. DOI: 10.1158/1535-7163.MCT-09-0978.
- Carvalho, A. L. N.; Annoni, R.; Torres, L. H. L.; Durão, A. C. C. S; Shimada, A. L. B; Almeida F. M. Anacardic Acids from Cashew Nuts Ameliorates Lung Damage Induced by Exposure to Diesel Exhaust Particles in Mice1. Evididence-Based Complementary and Alternative Medicine 2013, 549879. Epub 2013 Feb 27. DOI: 10.1155/2013/549879.
- Yi, Z.; Zhang, X.; Zhang, J.; Wu, Y.; He, L.; Liu, M.;Lai, L.; Lu, B. Medicinal Application of Anacardic Acid as Angiogenesis Inhibitor for Preventing and Treating Neovascularization Related Diseases Such as Tumor, Arthritis, Psoriasis and Neovascular Disease. Faming Zhuanli Shenqing 2010. CN101647793-A; CN101647793-B (Chinese patent).
- Paramashivappa, R.; Phani Kumar, P.; Vithayathil, P. J.; Srinivasa Rao, A. Novel Method for Isolation of Major Phenolic Constituents from Cashew (Anacardium occidentale L.) Nut Shell Liquid. Journal of Agricultural and Food Chemistry 2001, 49, 2548–2551. DOI: 10.1021/jf001222j.
- Shaw, H.-M.; Wu, J.-L.; Wang, M.-S. Antihypertensive Effects of Ocimum Gratissimum Extract: Angiotensin-Converting Enzyme Inhibitor in Vitro and in Vivo Investigation. Journal of Functional Foods 2017, 35, 68–73. DOI: 10.1016/j.jff.2017.05.033.
- Ahn, C. B.; Jeon, Y. J.; Kim, Y. T.; Je, J. Y. Angiotensin I Converting Enzyme (ACE) Inhibitory Peptides from Salmon Byproduct Protein Hydrolysate by Alcalase Hydrolysis. Process Biochemistry 2012, 47, 2240–2245. DOI: 10.1016/j.procbio.2012.08.019.
- Asoodeh, A.; Yazdi, M. M.; Chamani, J. K. Purification and Characterisation of Angiotensin I Converting Enzyme Inhibitory Peptides from Lysozyme Hydrolysates. Food Chemistry 2012, 131, 291–295. DOI: 10.1016/j.foodchem.2011.08.039.
- Ko, S. C.; Lee, J. K.; Byun, H. G.; Lee, S. C.; Jeon, Y. J. Purification and Characterization of Angiotensin I-Converting Enzyme Inhibitory Peptide from Enzymatic Hydrolysates of Styela Clava Flesh Tissue. Process Biochemistry. 2012, 47, 34–40. DOI: 10.1016/j.procbio.2011.10.005.
- Solomon, C. G.; Seely, E. W. Hypertension in Pregancy. Endocrinology Metabolism Clinics of North America 2001, 40, 847–863. DOI: 10.1016/j.ecl.2011.08.009.