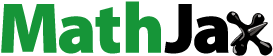
ABSTRACT
In this study, the effects of extrusion combined with cellulase on the structural, physicochemical, and functional properties of bamboo shoots dietary fiber were evaluated. Extrusion-cellulase treatment increased the contents of soluble dietary fiber (22.17 g/100 g dry solids). The scanning electron microscopy and Fourier transformed infrared spectroscopy analyses showed that the extrusion-cellulase treatment altered the structure of bamboo shoots dietary fiber without destroying the main components. Bamboo shoots dietary fiber modified by extrusion-cellulase treatment showed improved water-holding capacity (9.34 g/g dry solids), oil-holding capacity (10.74 g/g dry solids), swelling capacity (8.32 mL/g dry solids), cation exchange capacity and functional properties, including glucose adsorption capacity (158 mg/g dry solids), cholesterol adsorption capacity (9.8 mg/g dry solids (pH 2), and 11.4 mg/g dry solids (pH 7)), and nitrite ion adsorption capacity (29 μg/g dry solids (pH 2) and 21 μg/g dry solids (pH 7)). This experiment provided a theoretical basis for the modification of bamboo shoots dietary fiber, and the bamboo shoots dietary fiber modified by extrusion-cellulase can be useful as a fiber-rich ingredient in functional foods.
Introduction
China has the largest bamboo industry in the world. Worldwide, more than 2 million tons of bamboo shoots are consumed annually, of which approximately 1.3 million tons are produced in China.[Citation1] Bamboo shoots are a rich source of dietary fiber (DF),[Citation2] and DF is currently a common ingredient in breakfast cereals, pasta, shredded cheese, sauces, mustard, beverages, fruit cereals, and bakery products.[Citation3] It not only adds negligible calories to a product but also improves the texture. DF has beneficial physiological effects for human beings; previous studies have shown that the DF could chelate a variety of toxic metabolites produced by the food residue in the intestinal fermentation process, such as Ca2+, Zn2+, NO2−, acrylamide, and heavy metal ions.[Citation4,Citation5] Therefore, DF is associated with reduced risk of obesity, diabetes, cardiovascular disease, and certain types of cancers, and some evidence exists about prebiotic DF and its benefits to intestinal functions such as stool bulking, regularity, and consistency of depositions.[Citation6] The processing methods and functional properties of DF are related to its physicochemical and honeycomb structure. Compared with insoluble dietary fiber (IDF), soluble dietary fiber (SDF) not only exhibits more functional properties, but also possesses a greater capacity to provide viscosity, and acts as emulsifier. Moreover, SDF generally provides better texture and taste in comparison with IDF, and it is therefore easily applied in food processing.[Citation7,Citation8]
Bamboo shoots dietary fiber (BSDF) is an inexpensive alternative to fiber derived from wheat, oat, corn, potato, pea, soybean, and apples. The application of BSDF in food processing is increasing because of its extensive biological activities, such as antibacterial, antitumor, anticancer, immune regulation, etc.[Citation1] A recent study showed that BSDF could prevent obesity and improve lipid profiles in mice fed with high-fat diet by modulating the gut microbiota.[Citation9] Bamboo shoots have high amounts of fiber; however, the DF in bamboo shoots is mostly insoluble, and the low content of SDF in DF limits its applications in food industry. To date, biological fermentation and chemical methods have been developed to modify BSDF and increase SDF content in foods, but fermentation conditions are difficult to control, and chemical methods with limited reaction conditions lead to low SDF conversion efficiency and introduce detrimental chemical groups.[Citation10,Citation11] Therefore, it is important to develop a modification method that improves DF quality and increases SDF content in bamboo shoots. Numerous studies have shown that the thermal and chemical process improved specific physicochemical and functional properties of DF by decreasing particle size rather than by increasing SDF content.[Citation12,Citation13] However, it has been shown that enzymolysis can increase SDF content.[Citation14] Extrusion treatment is a popular physical modification method of fiber-rich products, which can improve the appearance, flavor, texture, and nutritional quality of foods.[Citation13] Cellulase can hydrolyze cellulose and hemicellulose components, which most likely led to increased exposure of functional groups and influences the bioactivity of DF.[Citation7]
The objective of this study was to increase the SDF content of BSDF by extrusion-cellulase treatment. The structural, physicochemical properties (water-holding capacity (WHC), oil-holding capacity (OHC), swelling capacity (SC), cation exchange capacity (CEC)), and functional properties (glucose adsorption capacity (GAC), cholesterol adsorption capacity (CAC), nitrite ion adsorption capacity) of different BSDF samples were evaluated.
Materials and methods
Materials
Fresh Nan bamboo shoots were supplied by Guizhou Red Chishui Group Co., Ltd. (Guizhou, China), and amylase (enzyme activity: ≥3700 U/g), neutral protease (enzyme activity: ≥60,000 U/g), and cellulase (enzyme activity: ≥20,000 U/g) were purchased from the Beijing Aoboxing Biotechnology Co., Ltd. (Beijing, China). The other reagents were of analytical grade.
Preparation of BSDF samples before treatment
Bamboo shoots were ground by a grinder (FW100, Tianjin Taisite Co. Ltd, Tianjin, China) and passed through a 40-mesh screen. Protein and starch were removed with protease and amylase as described by Li et al.[Citation11] Then, the bamboo shoot flour was extruded by a laboratory-scale twin-screw extruder (DS-32II model, Jinan, China). Initially, the optimum temperature, moisture content, and screw speed were evaluated by single-factor experiments. Extruded samples were dried for 24 h in a draft oven at 75°C (GZX-9140MBE Oven, Shanghai, China). The powder was passed through a 60-mesh screen. The optimized protocol for the enzymatic treatment of BSDF after treatment by extrusion was a cellulase/substrate ratio of 20 U/g for 240 min at pH 4.5 and 50°C.[Citation7] The bamboo shoot flour treated with extrusion-cellulase was heated in a boiling water bath for 15 min to terminate the enzymatic hydrolysis and allowed to cool to room temperature.
Determination of solubility
Previously described methods [Citation15] were applied to measure the contents of total dietary fiber (TDF), SDF, and IDF in unmodified bamboo shoots dietary fiber (BSDF1), extrusion treatment bamboo shoots dietary fiber (BSDF2), and extrusion-cellulase-treated bamboo shoots dietary fiber (BSDF3).
Scanning electron microscopy (SEM)
The structural changes of BSDF were determined by performing SEM (S-3400N, Hitachi, Ltd., Tokyo, Japan) before and after treatment. The dried samples were ground and passed through a 200-mesh sieve, placed on double-sided conducting adhesive tape, and coated with a 10 nm gold layer. Representative micrographs were taken at 500× and 3000× magnifications.
Fourier transformed infrared spectroscopy (FTIR)
Samples were thoroughly mixed with KBr (1:200, w/w) and pelletized. The IR spectra of samples were recorded with a Tensor 27 spectrometer (Nicolet is50, United States Thermo Fisher Scientific Co., Ltd.) from 400 to 4000 cm−1 with 32 scans and a 4 cm−1 resolution.
Water-holding capacity (WHC)[Citation16]
Samples (1 g dry solids) were hydrated with 25 mL of distilled water at room temperature for 18 h and centrifuged at 4000 rpm for 15 min. The weight of the residue was recorded both prior to drying (fresh weight) and after drying at 80°C (dry weight) until a constant weight was obtained. Each test was carried out in triplicate, and the readings were averaged. WHC was calculated by using Eq. (1):
where m1 = weight of the fresh residue (g) and m0 = weight of the dry residue (g).
Oil-holding capacity (OHC)[Citation17]
A total of 1.0 g of BSDF dried samples was dissolved in 25 mL corn germ oil using magnetic stirring at the required temperature for 30 min and incubated for 2 h at room temperature. Next, the mixture was centrifuged at 4000 rpm for 15 min. The supernatant was decanted from the centrifuge tube and dried at 80°C to constant weight. Each test was carried out in triplicate, and the readings were averaged; OHC was calculated by using Eq. (2):
where m = weight of dry BSDF(g), m0 = weight of centrifuge tube (g), and m1 = weight of the BSDF after absorbing oils and centrifugal tube.
Swelling capacity (SC)
The SC was determined according to a previous method with minor modification.[Citation15] Samples of 1.0 g each were hydrated with 10 mL distilled water in a graduated test tube at room temperature for 18 h. The volume of the dry samples and wet samples were recorded. SC was expressed as the volume of water (mL) per sample weight (g). Each test was carried out in triplicate, and averages were taken. SC was calculated by using Eq. (3):
where m = weight of dry BSDF (g), v0 = volume of dry BSDF (mL), and v1 = volume of BSDF after absorbing moisture.
Cation exchange capacity (CEC)
The CEC was based on the method described by Requena et al.[Citation6] Dry BSDF samples were immersed in 0.1 mol/L HCl. After 48 h, the excess acid was removed by distilled water, and the residue was freeze-dried. The samples (0.25 g) were dissolved in 100 mL of 15% NaCl solution and stirred well. Phenolphthalein (0.5%) was used as indicator; then, the mixture was slowly titrated with 0.01-mol/L NaOH solution and shaken for 5 min until fading at the end of titration was achieved, the consumption of pH in the process of titration was recorded, and a relationship diagram of pH value and consumption of VNaOH was created.
Glucose adsorption capacity (GAC)
GAC was determined following the method of Chen, Gao & Yang,[Citation18] with some modifications. The GAC of the DF was determined in vitro. Each sample (0.1 g) was mixed with 20 mL of glucose solution (50 mmol/L). The mixture was shaken at 37°C and 120 rpm for 2 h and then centrifuged at 4000 rpm for 15 min. The supernatant (1.0 mL) was collected in a graduated test tube, 3.0 mL of DNS reagent was added, and the mixture was immersed in boiling water for 5 min. After cooling to room temperature, the sample volume was made up with distilled water, and the absorption was analyzed using a spectrophotometer at 550 nm.
Cholesterol adsorption capacity (CAC)
The CAC of the fiber samples was investigated according to the method described by Xu, Jiao, Yuan & Gao,[Citation19] with minor modification. A fresh egg yolk was diluted by 8 volumes of distilled water, and homogenized by stirring. Dried samples (10 g) were mixed with 45 g of egg yolks. The mixture was adjusted to pH 2.0 (simulated stomach) or pH 7.0 (simulated intestine). The mixture was shaken at 37°C and 120 rpm for 2 h. After being cooled down to room temperature, the mixture was centrifuged at 4000 rpm for 25 min. The supernatant (4 mL) was collected, and the absorbance was measured using a spectrophotometer at 550 nm by the O-phthalaldehyde method. The CAC was expressed as gram of cholesterol absorbed per grams of sample.
Nitrite ion adsorption capacity
BSDF samples (1.0 g) were mixed with 50 mL of sodium nitrite solution (100 μmol/L). The pH was adjusted to 2.0 (simulated stomach) and 7.0 (simulated intestine). The mixture was shaken for 2 h at 37°C. The residual concentration of nitrite ion was subsequently measured, and the mixture was centrifuged at 4000 rpm for 25 min. The absorption of the supernatant was analyzed using a spectrophotometer at 550 nm. Nitrite ion adsorption capacity was calculated using the Eq. (4):
where m1 = weight of nitrite ion before adsorbed (μg), m0 = weight of nitrite ion after adsorbed (μg), and m = weight of BSDF (g).
Statistical analyses
Differences among the DF groups were determined by ANOVA, the data were expressed as the mean ± standard deviation (SD), and p values less than 0.05 were considered to indicate a significant difference among treatment. Data were analyzed using SPSS 17.0 software.
Results and discussion
Solubility
TDF, SDF, and IDF in the unmodified and modified DF samples are shown in . The contents of TDF in the unmodified samples, BSDF2, and BSDF3 were 56.64 g/100 g dry solids, 57.12 g/100 g, 59.48 g/100 g dry solids, respectively. Compared to the unmodified samples and BSDF2, the content of TDF was slightly higher in BSDF3, which might be attributed to protein residue unfolding, denaturation, and dissociation after treated by extrusion combined with cellulose; Ma et al[Citation8] reported similar results. As shown in , the extrusion process did not have a significant effect on TDF content. The content of SDF was significantly higher in the modified samples than the unmodified samples. This increase in SDF could be primarily due to the transformation of some IDF to SDF during extrusion-cellulase treatment. As shown in , the SDF content of samples modified by extrusion-cellulase was higher than that of samples modified by extrusion. The SDF content was 4.22 g/100 g dry solids in the unmodified bamboo shoots; however, the SDF yield in bamboo shoots increased to 6.14 g/100 g dry solids after modification by extrusion. The increase in SDF following extrusion might be due to the breaking of the long macromolecular chains of DFs caused by the high temperature, high pressure, and shear force, which would have produced more soluble small molecule components. Compared with the SDF yield of unmodified BSDF, that of bamboo shoots modified by extrusion-cellulase was higher reaching 22.17%, and this higher SDF content might have been due to the hydrolysis of insoluble cellulose and hemicellulose to soluble cell wall polysaccharides by cellulase. Arcila, Weier & Rose[Citation20] reported that the transformation of IDF to SDF during extrusion and the formation of additional SDF was caused by transglycosylation.
Table 1. The TDF, SDF, and IDF contents of BSDF.
DF can be classified as soluble or insoluble. DF solubility is related to the fiber’s chemical and physical structure and can define its physiological functionality. Compared with IDF, SDF not only exhibited a greater number of functional properties but also possesses a greater capacity to provide viscosity, form gels, and act as an emulsifier. Zhang, Decker & Mcclements[Citation21] studied the influence of DF addition on the gastrointestinal fate of emulsified lipids, which were investigated using a simulated gastrointestinal tract (GIT). The results indicate that the rate and extent of lipid digestion can be controlled by adding DFs, which may be useful information for the rational design of functional foods and beverages.
Scanning electron microscopy (SEM)
SEM micrographs of BSDF before and after modification are shown in . SEM images (a, b, c; 500×) showed that the extrusion and cellulase modification altered granule appearance of BSDF – unmodified BSDF (a) showed a regular surface with a higher number of holes than the modified BSDFs, the unmodified BSDF was broken into smaller fragments by extrusion modification (b) and extrusion combined with cellulase modification (c), and obvious particle rupture was observed with modified BSDF. Significant differences were observed in the structure of the unmodified BSDF (d, 3000×), extrusion modification BSDF (e, 3000×), and extrusion combined with cellulase modification BSDF (f, 3000×). The unmodified BSDF (d) exhibited a compact structure and regular surfaces. In contrast, BSDF modified by extrusion (e) and BSDF modified by extrusion combined with cellulase (f) had uneven, irregular, rough surfaces and fiber adhesion, obvious particle rupture, rougher and looser structures were observed with in SEM images, which indicated cell wall fragmentation and deep groove formation, caused by extrusion or cellulase and their combined effects. The altered surface and microstructure resulted in the high binding capacity and adsorption capacity of the modified BSDFs.
Figure 1. SEM of BSDF before and after modification, SEM (a) standard unmodified BSDF (500×), (b) standard modified by extrusion (500×), (c) standard modified by extrusion combined with cellulase (500×), (d) standard unmodified BSDF (3000×), (e) standard modified by extrusion (3000×), and (f) standard modified by extrusion combined with cellulase (3000×).
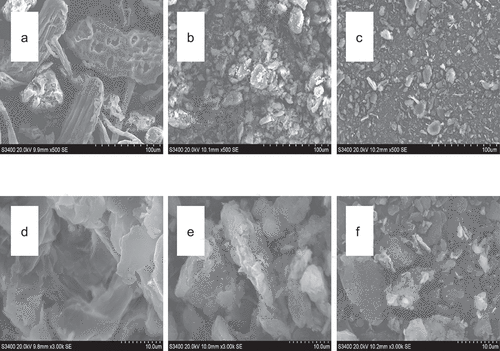
Fourier transformed infrared spectroscopy (FTIR)
FTIR spectra of unmodified, extrusion-modified and extrusion-cellulase modified BSDF are shown in . All absorption bands revealed that three types of DF-exhibited typical characteristics of polysaccharides. The main peaks approximately 3440, 2923, 1626, 1384, and 1078 cm−1 were attributed to the vibrations of O–H, C–H, C–O, COO–, and C–O–C, respectively.[Citation7] The band positions at approximately 3440 cm−1 and 2923 cm−1 were attributed to O–H and C–H stretching, respectively. Cellulose degradation contributed to a reduction in peak intensity and width in modified BSDFs, which indicated that methyl and methylene components of the hydrogen bonds in modified BSDFs were disrupted during extrusion/extrusion-cellulase treatment.[Citation22] The major absorption region at approximately 1735 cm−1 corresponded to the stretching of carbonyl groups and implied that more carbonyl groups and esters were generated in the process of modification. The characteristic absorption peak of the aromatic benzene in lignin was observed in three types of BSDF at 1626 cm−1, but the peak intensity and width at 1626 cm−1, 1510 cm−1, and 1384 cm−1 decreased in the BSDF modified by extrusion-cellulase, which was attributed to lignin degradation. The absorbance at approximately 1078 cm−1 and 778 cm−1 indicated the existence of a pyranoid ring structure in the molecules. These results suggest that extrusion treatment and cellulase can degrade lignin in BSDF and increase the relative amount of hemicellulose in BSDF, as reported by Park & Lee.[Citation23] Compared with the spectrum of unmodified BSDF, the spectrum of the modified BSDF showed redshifts. Furthermore, the peak intensity of the modified BSDF was greater than that of the unmodified BSDF, which was attributed to an increase in uronic acids and pectin in the former,[Citation24] indicating that extrusion and cellulase treatment severely destroyed the structure of organic molecules and increased the amounts of amorphous and soluble polysaccharides.[Citation25]
Physicochemical properties
The WHC is defined as the ability of a moist material to hold water when subjected to an external force. The OHC is used to assess the ability of DF to prevent oil loss during food processing and to reduce serum cholesterol levels by binding oil in the human digestive system.[Citation3] As shown in , significant increase was observed in WHC, OHC, and SC of the treated DFs relative to the values of the unmodified DF (p < 0.05). Extrusion increased the WHC, OHC, and SC of the DF from 4.58 g.g−1, 3.96 g.g−1, and 5.80 g.g−1 (initial value) to 7.70 g.g−1, 6.55 g.g−1, and 6.61 g.g−1, respectively. Some studies showed that BSDF subjected to a variety of forces led to the tight tissue structure of BSDF and the exposure to more pores, thereby facilitating the penetration of moisture and grease during the extrusion process. Thus, the WHC, OHC, and SC were improved by extrusion. Moreover, the surface features and overall charge density might also contribute to the water-/oil-holding capacity of DF.[Citation26,Citation27] The WHC, OHC, and SC of DF treated with cellulose increased to 9.34 g.g−1, 10.74 g.g−1, and 8.32 g.g−1, relative to the initial values, possibly due to the higher content of water-soluble components in the extrusion-cellulase-treated samples. Cellulase enzymolysis can reduce the molecular bonding and degree of polymerization of DF, thus increasing the surface area of its particles and exposing more hydrophilic groups. Because of the enhancement of dispersion, the DF particles are conducive to contact with water or oil. Moreover, the increased number of DF particles dissolved in water lead to swelling and stretching, which increase volume. Furthermore, the chemical composition of fibers can also affect water hydration properties, the surface features, overall charge density, and hydrophobic nature, which might contribute to the OHC of DF.[Citation27] WHC and OHC were positively correlated with the contents of SDF, and this result agrees with previous reports.[Citation28]
Table 2. Physicochemical properties of BSDF subjected to different treatments.
The physicochemical properties of DF (solubility, WHC, OHC, and SC) can be changed during the extrusion process and cellulase enzymolysis. DF can be classified as ordinary DF or high-quality DF; in high quality, the soluble content is greater than 10 g/100 g dry solids and the water retention capacity was greater than or equal to 7 g/g. Because of their beneficial physical and chemical properties, high-quality DFs have positive health effects on humans and can be used as food additives and functional ingredients in functional foods.[Citation29] BSDF, when used as an ingredient, improved the quality of “Amaretto” cookies.[Citation30] This present study showed that the addition of BSDF as an ingredient could help control moisture loss in high and intermediate moisture foods. Native DF from bamboo shoots was not commonly incorporated into food products due to its adverse effects on food quality, including negative effects on aesthetic and functional traits. For better use of DF in food, different modification methods have been employed to modify its physicochemical and functional properties related to health. For example, Zeng, Chen, Zhai, Wang & Xia[Citation31] reported the reduction of fat absorption by battered and breaded fish balls during deep-fat frying using fermented BSDF. BSDF modified by extrusion and a cellulase enzymatic method exhibited good physicochemical properties, which make it a suitable functional ingredient in food products.
Cation exchange capacity (CEC)
DF can be reversibly exchanged with cations, and its CEC depends on various numbers of functional groups. This property is influenced by some carboxyl groups and hydroxyl side chain groups in the chemical structure of DF. In addition, CEC is affected by DF particle size. The CEC values of the three types of BSDF are displayed in . The smaller the volume of NaOH, the higher the CEC achieved at the same pH. As is apparent from in , the order of cation exchange capacities was: BSDF3 > SDF2 > BSDF1. The differences among the three types of BSDF were significantly higher at pH 3.5 ~ 9.6 than at pH < 3.5 or pH > 9.6. This increase in CEC in the modified DFs occurred not only through a reduced ion absorption capacity, but also through a change in the instantaneous concentration of ions, usually expressed as dilute solution concentration to enhance the conversion rate. The pH and penetration pressure in the digestive tract affect the CEC of DF. This present results can be explained why the effect of acid or alkali on CEC was neutralized by changes in the DF structure.[Citation32]
Glucose adsorption capacity (GAC)
GAC is a useful in vitro parameter to estimate the effect of DF on the adsorption of glucose in the GIT. The GAC values of the different DF samples are shown in . The adsorption of glucose was determined by the methods reported by Peerajit, Chiewchan & Devahastin.[Citation33] The standard curve is Y = 0.1724 + 0.0168. As shown in , BSDF1 was able to bind glucose (99 mg/g dry solids), and extrusion increased the glucose absorption capacity of BSDF to 122 mg/g dry solids. Following extrusion-cellulase treatment, the GAC of BSDF increased to 158 mg/g. According to the mechanism proposed by Ahmed, Sairam & Urooj,[Citation34] the adsorption capacity of DF in the GIT is affected by two factors. First, the adsorption capacity of glucose is affected by the SDF contents, the high viscosity of SDF delays of glucose adsorption in the GIT. The high proportions of SDF in BSDF2 and BSDF3 after the redistribution of DF composition positively affected GAC. Second, as reported by Mu et al,[Citation35] structural changes such as decreased particle size, increased surface area, and a more porous fiber network would allow the glucose molecules to be embedded in the porous fiber network, which likely improves the interaction between DF and glucose. As seen in , there was a significant increase of 23 mg/g dry solids and 36 mg/g dry solids, after treatment. The results indicated that the structure of DF from bamboo shoots became more porous due to the hydrolysis of cellulose and hemicellulose by extrusion and cellulase, leading to good hydration, which is consistent with the results regarding solubility and WHC.
Cholesterol adsorption capacity (CAC)
DF is capable of adsorbing cholesterol in both the stomach and small intestine.[Citation36] The binding capacities of fiber samples to cholesterol (pH 2.0 and pH 7.0) are shown in . The standard curve was Y = 0.0097 × −0.002. pH had a significant effect on cholesterol adsorption ability in all three types of DF, and the binding capacities to cholesterol in the small intestine were higher than those in the stomach. The results indicated that BSDF modified by extrusion-cellulase exhibited better health effects in terms of lowering the concentration of cholesterol in the small intestine, which is similar to the results from a recent study.[Citation18] The present results suggest that the adsorption capacity of the DF modified by extrusion and cellulose (11.4 mg/g dry solids at pH 7, 9.8 mg/g dry solids at pH 2) was higher than that of DF modified by extrusion alone (8.6 mg/g dry solids at pH 7, 7.5 mg/g dry solids at pH 8.6) and that of unmodified DF (5.7 mg/g dry solids at pH 7, 3.3 mg/g dry solids at pH 2). In another study, cholesterol dissolved in solution was adsorbed by BSDF in vitro, demonstrating that soluble fiber forms a gel in the small intestines, which can bind excessive blood cholesterol delivered by the action of the liver and is eventually expelled from the body.[Citation37] IDF has been related to intestinal regulation, whereas SDF is associated with a decrease in cholesterol levels. Previous studies showed that in the cholesterol adsorption process of DF, a single molecule adsorption layer is rapidly formed on the surface, and then a multi-molecular adsorption layer is formed by the gravitational pull between molecules. Moreover, the active group on the surface of DF can also directly chelate the cholesterol molecules.[Citation38] The present results are consistent with the findings regarding solubility. Moreover, following modification, BSDF exposed more polar groups and had fewer space obstacles, which was conducive to its adsorption capacity. Furthermore, fiber with high CEC can entrap, disintegrate, and destabilize the emulsion of lipid and cholesterol, leading to a decrease in diffusion by forming complex fiber/micelles that act as barriers to the diffusion or absorption of micelles. As a result, the lipids and cholesterol cannot be efficiently absorbed and utilized, resulting in the reduction of blood cholesterol.[Citation6] Therefore, BSDF modified by extrusion-cellulase exhibits the potential to balance cholesterol in the body and improving physiological functions. Studies conducted by Park & Deokyoung[Citation38] similarly confirmed the beneficial effects of consuming a high-fiber diet containing bamboo shoots in terms of lowering blood cholesterol levels and improving bowel functions in healthy young women. The properties of modified BSDF also make it useful as thickening, bodying, and emulsifying agents for food applications and as low-calorie ingredients for fiber enrichment and dietetic snacks. [Citation39]
Figure 5. Cholesterol adsorption capacity before and after modification. Lowercase letters indicate significant differences (p < 0.05), and bars indicate the standard deviation from three replicates. pH 2 standard in vitro simulation of human stomach, and pH 7 standard in vitro simulation of human small intestine.
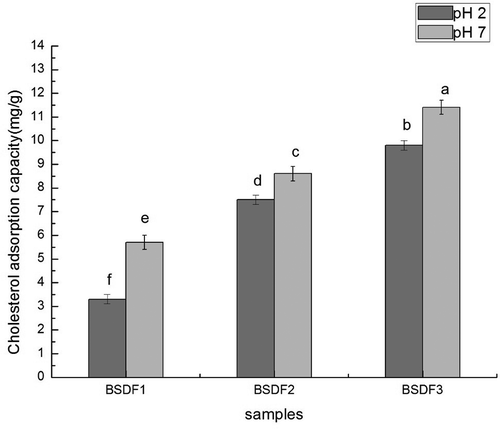
Nitrite ion adsorption capacity
Nitrite is an active ion that can react with secondary amines and amides under acid conditions to form N-nitroso compounds, many of which have been shown to be carcinogenic in animals. Nitrite scavenging capacity may play a protective role protect against the development of gastric cancer. The adsorption capacity of DF to nitrite is an important trait, as it contributes to the prevention of toxicity in humans due to nitrite overdose. In the present study, the nitrite ion absorption capacity was measured before and after modification. As shown in , the nitrite ion absorption capacity of BSDF increased after modification with extrusion-cellulase to 29.4 µg/g dry solids (at pH 2) and 21.3 µg/g dry solids (at pH 7). These results confirmed that extrusion-cellulase treatment efficiently improved the nitrite ion absorption capacity of BSDF. Previous studies have shown that such improvement is mainly due to the decrease in particle size, the increase in surface area, and the exposure of functional groups.[Citation40] Therefore, the nitrite ion adsorption capacity of BSDF modified by extrusion-cellulase was improved. Furthermore, the nitrite ion adsorption capacity of BSDF was greater at pH 7 than at pH 2. Mainly due to the increase of negative charge density in the DF surface with the increase in pH, the rejection to nitrite ion increased and reduced the adsorption capacity of DF.[Citation41]
Figure 6. Nitrite ion adsorption capacity before and after modification. Lowercase letters indicate significant differences (p < 0.05), and bars indicate the standard deviation from three replicates. pH 2 standard in vitro simulation of human stomach, and pH 7 standard in vitro simulation of human small intestine.
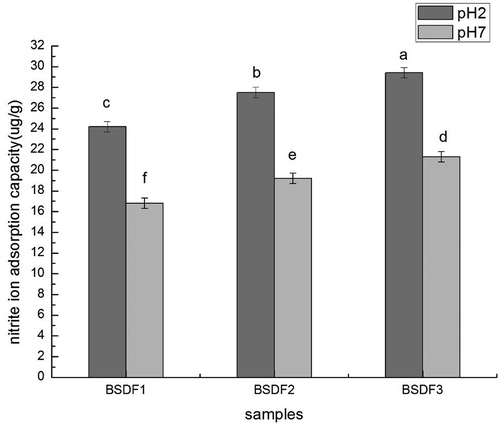
Based on the findings of this study, BSDF modified by extrusion-cellulase can be promoted as a modifier of viscosity, an additive of formulated products for decreasing calories, a sorbent for preventing fat upon cooking, and an adsorber or binder of bile acids to increase their excretion, which is associated with plasma cholesterol reduction.[Citation28] Extrusion-cellulase modification is a good method of BSDF modification, since it provides an adequate balance of SDF and IDF and enhances the physicochemical properties and functional properties of DF. Extrusion and cellulase enzymatic conditions, such as temperature, moisture, ratio and time, may change the contents as well as the physicochemical and physiological properties of DF.[Citation19,Citation22] Processing conditions of extrusion-cellulase have not been investigated in this study, and further studies are required to determine these conditions.
Conclusion
Extrusion-cellulase treatment markedly improved the content of SDF in bamboo shoots. Extrusion-cellulase hydrolysis significantly modified the microstructure of BSDF and enhanced its physicochemical and functional properties. The results revealed that BSDF modified by extrusion-cellulase exhibited good physicochemical and functional properties, which suggest it has potential applications in food products as a functional ingredient. BSDF modified by extrusion-cellulase treatment exhibited uneven, irregular, and rough surfaces, and adhered to adjacent fibers, and FTIR spectra revealed that extrusion-cellulase treatment degraded lignin and cellulose. The changes in the physical properties of modified BSDF were mainly related to the disruption of intermolecular bonds and changes in the crystalline structure during particle size reduction. Extrusion-cellulase can be applied for the modification of BSDF. Through extrusion-cellulase treatment, BSDF can be used as a good functional component to meet different requirements in functional foods. A future significant extension of this work would be an evaluation of its rheological and textural performances in food formulations.
Acknowledgments
The authors would like to thank Science and technology department of Guizhou province for the financial support under major subject of Guizhou science and technology (NY[2015]3024-2).
References
- He, M.-X.; Wang, J.-L.; Qin, H.; Shui, Z.; Zhu, Q.; Wu, B. Bamboo: A New Source of Carbohydrate for Biorefinery. Carbohydrate Polymers 2014, 111(20), 645–654. DOI: 10.1016/j.carbpol.2014.05.025.
- Santosh, S.; Lalitm, B.; Poonam, S. Bamboo Shoot Processing: Food Quality and Safety Aspect (A Review). Trends in Food Science & Technology 2010, 21(4), 181–189. DOI: 10.1016/j.tifs.2009.11.002.
- Navarrogonzález, I.; Garcíavalverde, V.; Garcíaalonso, J.; Periago, M.-J. Chemical Profile, Functional and Antioxidant Properties of Tomato Peel Fiber. Food Research International 2011, 44(5), 1528–1535. DOI: 10.1016/j.foodres.2011.04.005.
- Chen, J.; Zhao, Q.; Wang, L.; Zha, S.; Zhang, L.; Zhao, B. Physicochemical and Functional Properties of Dietary Fiber from Maca (Lepidium Meyenii Walp) Liquor Residue. Carbohydrate Polymers 2015, 132, 509–512. DOI: 10.1016/j.carbpol.2015.06.079.
- Qi, J.; Li, Y.; Masamba, K. G.; C-F, S.; Zhong, F. The Effect of Chemical Treatment on the in Vitro Hypoglycemic Properties of Rice Bran Insoluble Dietary Fiber. Food Hydrocolloids 2016, 52(08), 699–706. DOI: 10.1016/j.foodhyd.2015.08.008.
- Requena, M.; Gonzalez, C. A. N.; Barragan, L.-A.-P.; Correia, T.; Esquivel, J.-C.-C.; Herrera, R. Functional and Physico-Chemical Properties of Six Desert-Sources of Dietary Fiber. Food Bioscience 2016, 16, 26–31. DOI: 10.1016/j.fbio.2016.08.001.
- Ullah, I.; Yin, T.; Xiong, S.; Zhang, J.; Din, Z.-U. Structural Characteristics and Physicochemical Properties of Okara (Soybean Residue) Insoluble Dietary Fiber Modified by High-Energy Wet Media Milling. LWT-Food Science and Technology 2017, 82, 15–22. DOI: 10.1016/j.lwt.2017.04.014.
- Ma, -M.-M.; Mu, T.-H. Modification of Deoiled Cumin Dietary Fiber with Laccase and Cellulose under High Hydrostatic Pressure. Carbohydrate Polymers 2016, 136, 87–94. DOI: 10.1016/j.carbpol.2015.09.030.
- Li, X.; Guo, J.; Ji, K.; Zhang, P. Bamboo Shoot Fiber Prevent Obesity in Mice by Modulating the Gut Microbiota. Scientific Reports-UK 2016, 6, 32953. DOI: 10.1038/srep32953.
- Li, A.-P.; Xie, B.-X.; Zhong, Q.-P.; Wang, J. Study on Optimizing Bamboo Shoots Material Fermentation Conditions to Improve Dietary Fiber Quality by RSM. Chinese Journal of Food Science and Technology 2009, 30, 193–195.
- Li, A.-P.; Xie, B.-X.; Wang, J.; Tian, Y.-F. Comparison on the Preparation Method Function and Structure of Dietary Fiber from Bamboo Shoots. Journal of Chinese Institute of Food Science and Technolofy 2010, 10, 86–91.
- Chen, Y.; Ye, R.; Yin, L.; Zhang, N. Novel Blasting Extrusion Processing Improved the Physicochemical Properties of Soluble Dietary Fiber from Soybean Residue and in Vivo Evaluation. Journal of Food Engineering 2014, 120(1), 1–8. DOI: 10.1016/j.jfoodeng.2013.07.011.
- Peressini, D.; Foschia, M.; Tubaro, F.; Sensidoni, A. Impact of Soluble Dietary Fiber on the Characteristics of Extruded Snacks. Food Hydrocolloids 2015, 43, 73–81. DOI: 10.1016/j.foodhyd.2014.04.036.
- Cheng, L.; Zhang, X.; Hong, Y.; Li, Z.; Li, C.; Gu, Z. Characterisation of Physicochemical and Functional Properties of Soluble Dietary Fiber from Potato Pulp Obtained by Enzyme-Assisted Extraction. International Journal of Biological Macromolecules 2017, 101, 1004–1010. DOI: 10.1016/j.ijbiomac.2017.03.156.
- AOAC. Official Methods of Analysis of AOAC International, 16th ed.; 5th revision. Arlington, VA: AOAC International, 2005.
- López-Marcos, M.-C.; Bailina, C.; Viuda-Martos, M.; Pérez-Alvarez, J.-A.; Fernández-López, J. Properties of Dietary Fibers from Agroindustrial Coproducts as Source for Fiber-Enriched Foods. Food & Bioprocess Technology 2015, 8(12), 2400–2408. DOI: 10.1007/s11947-015-1591-z.
- Lan, G.; Chen, H.; Chen, J.; Tian, J. Chemical Composition and Physicochemical Properties of Dietary Fiber from Polygonatum Odoratum as Affected by Different Processing Methods. Food Research International 2012, 49(1), 406–410. DOI: 10.1016/j.foodres.2012.07.047.
- Chen, J.; Gao, D.; Yang, L.; Gao, Y. Effect of Microfluidization Process on the Functional Properties of Insoluble Dietary Fiber. Food Research International 2013, 54(2), 1821–1827. DOI: 10.1016/j.foodres.2013.09.025.
- Xu, H.; Jiao, Q.; Yuan, F.; Gao, Y. In Vitro Binding Capacities and Physico-Chemical Properties of Soluble Fiber Prepared by Microfluidization Pretreatment and Cellulase Hydrolysis of Peach Pomace. LWT-Food Science Technology. 2015, 63(1), 677–684. DOI: 10.1016/j.lwt.2015.03.033.
- Arcila, J. A.; Weier, S. A.; Rose, D. J. Changes in Dietary Fiber Fractions and Gut Microbial Fermentation Properties of Wheat Bran after Extrusion and Bread making. Food Research International 2015, 74, 217–223. DOI: 10.1016/j.foodres.2015.05.005.
- Zhang, R.; Zhang, Z.; Zhang, H.; Decker, E.-A.; Mcclements, D.-J. Influence of Emulsifier Type on Gastrointestinal Fate of Oil-In-Water Emulsions Containing Anionic Dietary Fiber (Pectin). Food Hydrocolloids 2015, 45, 175–185. DOI: 10.1016/j.foodhyd.2014.11.020.
- Honců, I.; Sluková, M.; Vaculová, K.; Sedláčková, I.; Wiege, B.; Fehling, E. The Effects of Extrusion on the Content and Properties of Dietary Fiber Components in Various Barley Cultivars. Journal of Cereal Science 2016, 68, 132–139. DOI: 10.1016/j.jcs.2016.01.012.
- Park, K.-H.; Lee, K.-Y.; Lee, H.-G. Chemical Composition and Physicochemical Properties of Barley Dietary Fiber by Chemical Modification. International Journal of Biological Macromolecules 2013, 60(6), 360–365. DOI: 10.1016/j.ijbiomac.2013.06.024.
- Feng, Z.; Dou, W.; Alaxi, S.; Niu, Y.; Yu, L. Modified Soluble Dietary Fiber from Black Bean Coats with Its Rheological and Bile Acid Binding Properties. Food Hydrocolloids 2017, 62, 94–101. DOI: 10.1016/j.foodhyd.2016.07.032.
- Zhao, X.; Chen, J.; Wang, X.; Zhu, Q. Surface Characterization of Corn Stalk Superfine Powder Studied by FTIR and XRD. Colloids & Surfaces B Biointerfaces 2013, 104(3), 207–212. DOI: 10.1016/j.colsurfb.2012.12.003.
- Long, D.; Ye, F.; Zhao, G. Optimization and Characterization of Wheat Bran Modified by in Situ Enchanced CO2 Blasting Extrusion. LWT-Food Science and Technology 2014, 59(2), 605–611. DOI: 10.1016/j.lwt.2014.07.017.
- Zha, S.; Zhao, Q.; Chen, J.; Wang, L. Extraction, Purification and Antioxidant Activities of the Polysaccharides from Maca (Lepidium Meyenii). Carbohydrate Polymers 2014, 111, 584–587. DOI: 10.1016/j.carbpol.2014.05.017.
- Lv, J.-S.; Liu, X.-Y.; Zhang, X.-P.; Wang, L.-S. Chemical Composition and Functional Characteristics of Dietary Fiber-Rich Powder Obtained from Core of Maize Straw. Food Chemistry 2017, 227, 383–389. DOI: 10.1016/j.foodchem.2017.01.078.
- Ding, -S.-S.; Huang, L.-X.; Zhang, C.-H.; Xie, P.-J.; Zhang, Q. Research Progress on Preparation, Properties Determinations and Modification of Dietary Fiber. Food Science & Technology of Food Industry 2016, 37, 381–386.
- Stefano, F.; Luciano, P. Effects of Ingredients and Process Conditions on “Amaretti” Cookies Characteristics. International Journal of Food Science & Technology 2010, 43, 1395–1403.
- Zeng, H.; Chen, J.; Zhai, J.; Wang, H.; Xia, W. Reduction of the Fat Content of Battered and Breaded Fish Balls during Deep-Fat Frying Using Fermented Bamboo Shoot Dietary Fiber. LWT-Food Science and Technology 2016, 73, 425–431. DOI: 10.1016/j.lwt.2016.06.052.
- Benitez, V.; Molla, E.; Martin-Cabrejas, M.-A.; Aguilera, Y.; Esteban, R.-M. Physicochemical Properties and in Vitro Antidiabetic Potential of Fiber Concentrates from Onion By-Product. Journal of Functional Foods 2017, 36, 34–42. DOI: 10.1016/j.jff.2017.06.045.
- Peerajit, P.; Chiewchan, N.; Devahastin, S. Effects of Pretreatment Methods on Health-Related Functional Properties of High Dietary Fiber Powder from Lime Residues. Food Chemistry 2012, 132(4), 1891–1898. DOI: 10.1016/j.foodchem.2011.12.022.
- Ahmed, F.; Sairam, S.; Urooj, A. In Vitro Hypoglycemic Effects of Selected Dietary Fiber Sources. Journal of Food Science & Technology 2011, 48(3), 285–289. DOI: 10.1007/s13197-010-0153-7.
- Ma, -M.-M.; Mu, T.-H.; Sun, H.; Zhang, M.; Chen, J.; Yan, Z. Optimization of Extraction Efficiency by Shear Emulsifying Assisted Enzymatic Hydrolysis and Functional Properties of Dietary Fiber from Deoiled Cumin (Cuminum Cyminum L.). Food Chemistry 2015, 179, 270–277. DOI: 10.1016/j.foodchem.2015.01.136.
- Deepak, M.; Sheweta, B. Composition, Properties and Health Benefits of Indigestible Carbohydrate Polymers as Dietary Fiber: A Review. International Journal of Biological Macromolecules 2013, 61(10), 1–6. DOI: 10.1016/j.ijbiomac.2013.06.044.
- Zhang, N.; Huang, C.; Ou, S. In Vitro Binding Capacities of Three Dietary Fibers and Their Mixture for Four Toxic Element, Cholesterol, and Bile Acid. Journal of Hazardous Materials 2011, 186(1), 236–239. DOI: 10.1016/j.jhazmat.2010.10.120.
- Park, E.-J.; Deokyoung, J. Effects of Bamboo Shoots Consumption on Lipid Profiles and Bowel Function in Healthy Young Women. Nutrition 2009, 25(7–8), 723–728. DOI: 10.1016/j.nut.2009.01.007.
- Gunness, P.; Gidley, M.-J. Mechanisms Underlying the Cholesterol-Lowering Properties of Soluble Dietary Fiber Polysaccharides. Food & Function 2010, 1(2), 149–155. DOI: 10.1039/c0fo00080a.
- Zhu, F.-M.; Du, B.; Li, J. Effect of Ultrafine Grinding on Physicochemical and Antioxidant Properties of Dietary Fiber from Wine Grape Pomace. Food Science and Technology International 2014, 20(1), 55–62. DOI: 10.1177/1082013212469619.
- Ruan, C.-Y.; Tu, Z.-C.; Wang, H.; Liu, J.-K.; Xiao, S.-H.; Qin, X.-H. In Vitro Adsorption Capacity of Dietary Fibers from Soybean Dregs. Chinese Journal of Food Science 2014, 35, 109–112.