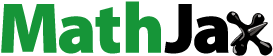
ABSTRACT
This study investigated the effects of liquid nitrogen quick-freezing, −35°C air-blast freezing, and −18°C refrigerator direct-freezing on quality retention of frozen giant freshwater prawns using various indicators: microscopic structure, physicochemical, texture, and color properties. The results showed that the shelf lives of prawns treated with liquid nitrogen quick-freezing, −35°C air-blast freezing, and −18°C refrigerator direct-freezing were about 20, 12, and 8 weeks, respectively, in terms of total volatile basic nitrogen content and K-value. Meanwhile, liquid nitrogen quick-freezing could effectively improve texture property of frozen giant freshwater prawn. These findings confirm that liquid nitrogen quick-freezing is a promising method to extend the shelf life of frozen prawns.
Introduction
Freshness is an important factor that influences the quality, consumer acceptability, and commercial value of prawns. Rapid deterioration of prawns quality after death is considerably associated with the autolysis and microbial spoilage throughout postmortem storage.[Citation1] To obtain prawns with premium quality, frozen storage is the major method for preserving prawns by decreasing temperature to inhibit most microbial growth, enzyme activity, and physicochemical reactions.[Citation2] However, some quality deterioration of prawn inevitably happens during frozen storage, especially the indexes of texture, color, and flavor because of osmotic removal of water, muscle fiber destruction caused by ice crystals, as well as aggregation of myofibrillar protein.[Citation2–Citation4]
The current studies find that the freezing rate has close relation with the quality of aquatic products during frozen storage.[Citation5] Hong et al.[Citation5] analyzed the effects of different freezing treatments on the quality changes of bighead carp heads during ice storage and found that the treatment of freezing at −40°C for 12 h showed lower values of TVB-N, pH, and thiobarbituric acid reactive substances (TBARS) in bighead carp heads compared to those frozen directly at −18°C after 3 months of frozen storage. Boonsumrej et al.[Citation6] evaluated the quality changes of tiger shrimp (Penaeus monodon) frozen using air-blast and cryogenic freezing. The results indicated that freezing the shrimps under the air-blast freezer at the air velocity of 6 m/s had the least freezing loss and same cutting force as the fresh shrimps. In addition, some studies also showed that shrimps frozen by liquid nitrogen retained the quality well which was used as a safe coolant in a simple and rapid freezing method. Goncalves and Ribeiro[Citation7] concluded that the use of liquid nitrogen freezing improved process yield when compared to the use of spiral freezer. At present, some literatures have reported that the influence of a single or two of the freezing methods on the quality of aquatic products, the information regarding the comparison of liquid nitrogen quick-freezing, −35°C air-blast freezing, and −18°C refrigerator direct-freezing that affecting the quality characteristics of prawn for frozen storage is still limited.
Giant freshwater prawn (Macrobrachium rosenbergii) is one of the most important crustacean species cultured in China. According to a report of 2017 China Fishery Statistical Yearbook, the production of giant freshwater prawn was estimated to 132,678 t in 2016.[Citation8] The prawn is a popular food for consumers because of its delicacy and rich nutrient. However, it has the seasonal characteristic and quality deterioration occurs during frozen storage. At present, producers have found that the quality of prawns treated by −35°C air-blast freezing would deteriorate after 2–3 months of storage. Therefore, developing an effective freezing method to improve quality and extend the shelf life of the frozen giant freshwater prawns is potentially valuable. The objective of this study is to investigate the effect of liquid nitrogen quick-freezing compared with −35°C air-blast freezing and −18°C refrigerator direct-freezing on the quality and predict the shelf life of freshwater prawns by evaluating the physicochemical and texture properties during frozen storage.
Materials and methods
Materials
A total of 15 kg fresh live giant freshwater prawns (M. rosenbergii) with an average weight of 18 ± 2 g was selected in uniform size and purchased from a local market (Vanguard, Wuxi, Jiangsu, China) in August and transported to the Food Processing Technology Lab of Jiangnan University within 20 min. The prawns were killed by slurry ice immediately.
Freezing treatment
After washing with cold distilled water, raw prawns (headed and unpeeled) were randomly divided into three groups. One group was frozen with liquid nitrogen quick-freezing (S1) by immersing samples in liquid nitrogen supplied by Liquid nitrogen container (Chart BioMedical Co., Ltd., Chengdu, China). Ten prawns per batch were frozen in about 2 L liquid nitrogen and neatly 1 L liquid nitrogen was consumed for freezing 1 kg prawn. The −35°C air-blast freezing (S2) was carried out at −35°C using an air-blast instant freezer (Luo Cheng Cateping Equipment Co., Ltd., Shanghai, China). Meanwhile, the remaining group was directly frozen in −18°C refrigerator (S3). All prawns were frozen individually. The freezing process was finished when the temperature at the center of the sample reached −18°C. The changes in internal temperature of samples during freezing for each method were monitored using a TP9000 digital thermometer (Toprie Electronic Co., Ltd., Guangdong Province, China). After inserting the thermometer probe with diameter about 1 mm into the second abdominal segment of the prawn, the prawn with probe was then placed at the central of the group of prawns. The prawns were removed immediately when the internal temperature of prawn reached −18 ± 0.5°C (S1: 20 s, S2: 11 min, and S3: 110 min, respectively) and then randomly sealed in polyethylene bags. One bag was used for each sampling day. All the freezing experiments were conducted in six times. Then, the prawns were stored in a refrigerator with −18 ± 0.5°C for 20 weeks. The prawns were randomly taken for analysis after thawing at 4 ± 0.5°C on the sampling points of the 0th (fresh), 4th, 8th, 12th, 16th, and 20th week of storage.
Microscopic analysis
From frozen stored sample (stored at −18°C for 1 day), six pieces (approximately 5 mm in length) were cut from the center, transversally to the muscle fiber, using a blade previously cooled to −18°C. This operation was done in a walk-in freezer to ensure a perfect cold chain. Microscopic analysis of samples was determined to Su et al.[Citation9] with some modifications in staining method. The slice samples were immersed in an orange G aqueous solution (0.5% orange G, 1% acetic acid, 98.5% distilled water) for 2 min for coloration of fiber muscle. Finally, the samples were sealed on glass plates using a mounting medium for microscope preparation. Thus, in total for each treatment, there were six slides for microscopic analysis.
Physicochemical quality evaluation
Thawing loss (%) was determined after thawing. The exudate in the polyethylene bags and on the surface of the prawns was wiped up by using filter paper. The thawing loss was calculated as follows:
W1 is the weight of the prawn, exudate, and polyethylene bag; W2 is the weight of the prawn and polyethylene bag; and W3 is the weight of polyethylene bag.
The pH value of sample was determined according to Yu et al.[Citation10] Five grams of sample was homogenized with 50 mL cold distilled water using a T10 Basic Ultra-Turrax homogenizer (IKA Werke GmbH & Co. KG, Staufen, Germany) at a speed of 13,500 rpm for 1 min, and the pH of the homogenate was determined using a SevenEasy pH meter (Mettler-Toledo GmbH, Schwerzenbach, Switzerland).
Total volatile basic nitrogen (TVB-N, mg N/100 g sample) content was estimated according to the current Chinese industry standard method for fishery products (SC/T3032-2007). Five grams of minced sample was homogenized with 45 mL perchloric acid (0.6 M), and the suspension was filtered. The supernatant (5 mL) was distilled after the addition of 5 mL 30 g/L NaOH and phenolphthalein indicator using a KDN-103F Kjeldahl Apparatus (Shanghai Qianjian Instrument Co., Ltd., Shanghai, China). The distillate was collected in a flask containing 10 mL of 30 g/L boric acid with methyl red–bromocresol green mixed indicator. Then, the boric acid solution was titrated with a 0.01-M hydrochloric acid standard solution.
TBARS value was measured using colorimetric methods according to Benjakul and Bauer.[Citation11] Briefly, minced muscle (5 g) was homogenized thoroughly with 20 mL 10% (w/v) trichloroacetic acid solution for 1 min. After filtration, the extract solution was mixed with the same volume of 0.02 M TBA solution and heated for 20 min in a boiling water bath. The absorbance of cooled solution was measured at 532 nm using a UV–vis spectrophotometer (UV-1000, Techcomp Co., Ltd., Shanghai, China), against a blank prepared from 5 mL TBA and 5 mL three chloroacetic acid (TCA) solutions. The TBARS value was calculated from the standard curve and expressed as mg malonaldehyde (MDA)/kg sample.
K-value (%) was determined according the method described by Yu et al.[Citation12] Nucleotides were extracted with 6% (v/v) cold perchloric acid solution. Two grams of sample was homogenized with 10 mL extracting solutions and centrifuged at 10,000g for 5 min at 4°C (4K15 centrifuge, Sigma Laborzentrifugen GmbH, Osterode, Germany). The extraction process was repeated once and the combined supernatants were neutralized immediately to pH 6.5–6.8 with 10 and 1 M KOH solutions. The precipitate in neutralized solution was removed by centrifugation (3000g, 5 min) and the supernatant was diluted to 25 mL with cold distilled water. The final solution was filtered using 0.22 μm membrane filter (Dongkang Technology Co., Ltd., Tianjin, China) and ATP-related compounds were determined using HPLC (Waters e2695, Milford, Massachusetts, USA), equipped with a photo-diode array detector (Waters 2998) and a Waters C18 column (5 μm, 4.6 mm id × 250 mm). The mobile phase was 98% potassium phosphate buffer (0.05 M, pH 6.8) and 2% methanol, and detection wavelength was 254 nm. The standards including ATP (≥98.5%), ADP (≥95%), AMP (≥99%), IMP (≥98%), Hx (≥99%), and HxR (≥98%) (Sigma-Aldrich Co., Shanghai, China) were detected separately and used for calculation of each compound based on the peak areas provided by the HPLC software. The formula for K-value was as follows:
Instrumental texture profile analysis
Instrumental texture profile analysis was carried out using a TA.XT Plus texture analyzer (Stable Micro Systems, Surrey, UK) equipped with a cylindrical probe (P/36R). The shell less prawns were compressed twice to 40% of their original height at a testing speed of 1 mm/s. Besides, the pretest and posttest speeds were also 1 mm/s. Hardness, springiness, chewiness, and resilience were performed in six replications and calculated using the Texture Expert software version 1.22 (Stable Micro Systems, Surrey, UK).
Color measurement
The color of the second abdominal segment of a shell less prawn muscle was measured according to the method of Dai et al.[Citation13] using the L*, a*, b* mode of CIE with a chromometer (Model CR-400, Konica Minolta, Osaka, Japan). The measurement of L* (indicator of lightness), a* (indicator of redness/greenness), b* (indicator of yellowness/blueness), and dE* (chromatic value) was performed in six replications.
Statistical analysis
The results were expressed as the mean of three determinations except central temperature, instrumental texture, color, and sensory analysis, which were carried out six times. Statistical analysis was performed using SPSS 19.0 (SPSS Inc., Chicago, Illinois, USA). A Duncan’s test was used for determining significant differences (p < 0.05).
Results and discussion
Central temperature determination
In the freezing process, the freezing rate is closely related to the nucleation and growth of ice crystals in aquatic products.[Citation9] The freezing curves obtained for the prawns with three different freezing methods are summarized in . The temperature range from −2 to −5°C in prawns was specified as the maximal crystallization zone.[Citation14] The times through the maximal crystallization zone were 0.1, 1.2, and 15 min for S1, S2, and S3, respectively, and the freezing rates of S1 and S2 were much faster than that of S3. The times through the maximal crystallization zone were calculated for the average of six freezing experiments. According to Bello et al.,[Citation15] rapid freezing produces small ice crystals which can be distributed evenly and cause minimum dislocation of tissue ultrastructural components. Therefore, liquid nitrogen quick-freezing and −35°C air-blast freezing might play the positive role in keeping the quality of giant freshwater prawn for later frozen storage compared with −18°C refrigerator direct-freezing.
Ice crystal microstructure analysis
shows the micrographs of fresh and frozen (−18°C for 1 day) samples tissues. Unfrozen fresh prawn sample was used as the control for the purpose of microstructure comparison with the frozen treatments. S1 had uniform and the smallest ice crystals. The small ice crystals of granular shape dispersed throughout the sample without specific orientation, indicating that ice nucleation occurred throughout the prawn. As a result, there was less damage to tissues and cell structures by S1. S2 showed relatively bigger ice crystals due to the slower freezing rate in the process compared with S1. In addition, ice crystals formed by S3 showed the largest size with very irregular shapes, which was similar to the result reported by Su et al.[Citation9] S3 was the slowest freezing process among groups, resulting in the biggest ice crystals. Thus, liquid nitrogen quick-freezing generally contributed to reduce mechanical damage of tissues and cell structures and reserve the maximum attainable food quality.
Physicochemical quality evaluation
Thawing loss is unappealing to consumers in that it may affect juiciness, free amino acids, nucleotides, and texture.[Citation16] Thawing loss in each group of prawns during storage is summarized in (). A rapid increase in thawing loss was observed for the early stage, and then the thawing loss continued to increase gradually during the subsequent period of frozen storage for prawns treated with three freezing methods. However, the initial lag phases of 8, 2, and 0 weeks without significant (p > 0.05) changes in thawing loss values were observed for S1, S2, and S3, respectively. Meanwhile, the thawing loss values of S1 were lower than those of S2 and S3 during frozen storage. Thawing loss is mainly associated with the structure of muscle,[Citation17] thus lower thawing loss of S1 with the longer detention time attributed to the smallest ice crystals formed and well distributed in the cell and intercellular by liquid nitrogen quick-freezing as shown in .
Figure 3. Changes in (a) thawing loss, (b) pH, (c) TVB-N, and (d) TBARS of giant freshwater prawn during the storage at −18°C.
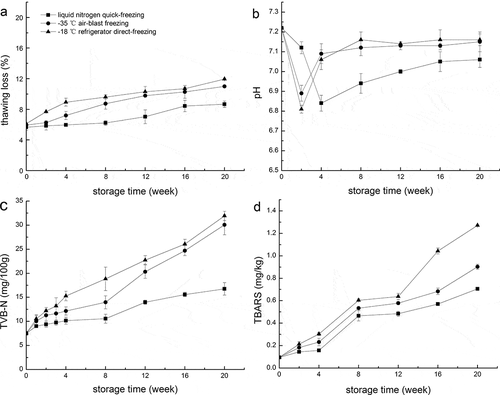
Changes in pH values of prawns during storage are shown in (). The pH values of S1, S2, and S3 sharply decreased (p ˂ 0.05) from 7.22 ± 0.02 (fresh) to 6.81 ± 0.02, 6.89 ± 0.04, and 6.84 ± 0.04, respectively, and then increased during the following stage of storage. The initial decrease in pH might be related to the generation of lactic acid by anaerobic glycolysis and the liberation of inorganic phosphate by the degradation of ATP during the rigor stage of prawn, while the later increase might be caused by the accumulation of alkaline compounds, such as biogenic amines and ammonia produced by the action of microorganisms and endogenous.[Citation10,Citation18,Citation19] In addition, the significantly higher growth rates of S2 and S3 were observed compared with S1 (p < 0.05) in the subsequent increasing stage, suggesting that liquid nitrogen quick-freezing might inhibit microbial spoilage and endogenous activities.
TVB-N, as a result of either bacterial or enzymatic degeneration, is one of important freshness indicators to assess spoilage degree of aquatic products.[Citation20] Changes in TVB-N content in all groups during storage are presented in (). The initial TVB-N content of 7.54 ± 0.43 mg N/100 g was determined for fresh prawn and the contents in groups increased gradually with the final values of 16.75 ± 1.30, 30.06 ± 2.07, and 31.93 ± 0.96 mg N/100 g for S1, S2, and S3, respectively. According to Liu et al.[Citation17] and the current Chinese hygienic standard for typical freshwater species (GB 2733-2015), the limit level of TVB-N content is 20.00 mg N/100 g. In the 12th week, the TVB-N levels of S2 (20.33 ± 3.40 mg N/100 g) and S3 (22.74 ± 0.92 mg N/100 g) exceeded the limit level, while TVB-N level of S1 (13.98 ± 0.44 mg N/100 g) still not reach the limit level until the 20th week. Apparently, S1 has better quality retention time than S2 and S3 for more than 8 weeks.
The variations in TBARS values for prawns during frozen storage are shown in (). Prawns started with a low TBARS value of 0.096 ± 0.008 mg MDA/kg, which was lower than value reported by Farajzadeh et al.[Citation21] The result showed that TBARS values increased gradually from 0.096 ± 0.008 to 0.705 ± 0.013, 0.902 ± 0.013, and 1.271 ± 0.005 mg MDA/kg at 20th week for S1, S2, and S3, respectively. The significantly (p < 0.05) higher TBARS values in S3 indicated that more off-flavor carbonyl compounds were generated as a result of fatty acid oxidation during frozen storage. In addition, TBARS value of 1–2 mg MDA/kg sample was regarded as the limit of acceptability according to Yu et al.[Citation22] The final values of S1 and S2 (S1 < S2) were still below the minimum limit, while that of S3 exceeded the minimum limit. These results indicated that rapid freezing was an effective measure to inhibit lipid oxidation in prawn, especially liquid nitrogen quick-freezing.
K-value analysis
The changes of K-value in freshwater prawns during frozen storage are presented in . The initial K-value of fresh prawn was 2.08 ± 0.12% and the change trends in three groups were similar with continuous increase during following storage. Compared to S3, S1 and S2 significantly delayed the increase of K-value. At the end of storage, the K-value of S1 was only 19.6 ± 1.11%, much lower than the other two treatment groups (S2: 31.35 ± 2.08%, S3: 38.19 ± 2.10%). The increase of K-value was mainly attributed to degradation of IMP and the accumulation of HxR and Hx, which were possibly due to spoilage by both autolytic and microbial enzymes.[Citation10] Therefore, the lower K-value in S1 might be due to the lower enzyme activities. According to the previous evaluation criterion, K-value less than 20% was very fresh, from 20% to 50% was moderately fresh, and above 70% regarded as rejection point.[Citation23] The prawns with S1 were very fresh during the storage of 20 weeks; however, the moderate freshness appeared after the 12th and 8th week for S2 and S3. The shelf life based on K-value prediction model correlated well the results of TVB-N analysis.
Texture analysis
The instrumental texture parameters of prawn muscle are presented in . During the entire storage period, the values of hardness, springiness, chewiness, and resilience decreased sharply (p < 0.05) within the first 8 weeks and then changed slowly until the end of storage for the three freezing methods. After 8 weeks, no further significant (p > 0.05) decrease was observed in all groups of samples, suggesting that the early storage phase was responsible for the major changes in prawn texture. The changes of texture properties under the freezing conditions are particularly influenced by the two main factors: ice crystals formation and protein denaturation induced by endogenous enzymes and microbial activities.[Citation24,Citation25] The initially rapid decrease in hardness of S3 might be the accelerated damages of tissue structures caused by the big ice crystals as shown in . Therefore, texture properties such as hardness could be improved during frozen storage by rapid freezing, especially liquid nitrogen quick-freezing.
Table 1. Changes in texture properties of freshwater prawn during frozen storage.
Color measurement
Color is a principal determinant of prawn quality, being closely associated with freshness.[Citation2] Surface color parameters for the prawn muscle during frozen storage are shown in . All of the samples suffered a decrease of lightness (L*) decrease and gradual increases of redness (a*) and chromatic value (dE*) with the storage time. However, the changes of b* values were irregular both in storage period and different freezing treatments. The prawn muscle gradually lost glossiness because of water loss and concentration of pigment as frozen storage progressed which lead to the decrease of L* values.[Citation26] Possible reason suggested for a* changes was that phenoloxidase existed in prawn would react with dioxy phenylalanine to produce melanin even during frozen storage.[Citation27] Prawns treated by S1 with higher L* at the end of storage might be due to the formation of the small ice crystals induced less decline of water loss, which was accordance with the changes of thawing loss. However, no significant difference (p > 0.05) of L*, a*, and dE* was observed in S1, S2, and S3 during the frozen storage.
Table 2. Changes in color of freshwater prawn during frozen storage.
Conclusion
Rapid freezing methods including liquid nitrogen quick-freezing and −35°C air-blast freezing effectively maintained good quality of giant freshwater prawn during frozen storage, especially liquid nitrogen quick-freezing. The results of physiochemical, texture, and color analyses proved that liquid nitrogen quick-freezing successfully inhibited the thawing loss, texture softening, and chemical spoilage, as well as improved color quality of giant freshwater prawn. At the same time, the shelf life of prawns treated by liquid nitrogen quick-freezing was prolonged by about 4 and 8 weeks compared with −35°C air-blast freezing and −18°C refrigerator direct-freezing, respectively. Thus, this finding supports that liquid nitrogen quick-freezing has the potential to provide the fresh quality of frozen prawn with longer time.
Additional information
Funding
References
- Zhu, S.; Zhou, Z.; Feng, L.; Luo, Y. Postmortem Changes in Physicochemical Properties of Songpu Mirror Carp (Cyprinus Carpio) during Iced Storage. Food Bioscience 2015, 9, 75–79. DOI: 10.1016/j.fbio.2014.12.003.
- Wachirasiri, K.; Wanlapa, S.; Uttapap, D.; Puttanlek, C.; Rungsardthong, V. Changing in Processing Yield and Physical Properties of Frozen White Shrimp (Penaeus Vannamei) Treated with Lysine and Sodium Bicarbonate. International Journal of Food Science and Technology 2017, 52, 763–771. DOI: 10.1111/ijfs.13333.
- Shafieipour, A.; Sami, M. The Effect of Different Thawing Methods on Chemical Properties of Frozen Pink Shrimp (Penaeus Duorarum). Iranian Journal of Veterinary Medicine 2015, 9, 1–6.
- Ozogul, Y.; Durmus, M.; Balikci, E.; Ozogul, F.; Ayas, D.; Yazgan, H. The Effects of the Combination of Freezing and the Use of Natural Antioxidant Technology on the Quality of Frozen Sardine Fillets (Sardinella Aurita). International Journal of Food Science and Technology 2011, 46, 236–242. DOI: 10.1111/j.1365-2621.2010.02476.x.
- Hong, H.; Luo, Y.; Zhou, Z.; Bao, Y.; Lu, H.; Shen, H. Effects of Different Freezing Treatments on the Biogenic Amine and Quality Changes of Bighead Carp (Aristichthys Nobilis) Heads during Ice Storage. Food Chemistry 2013, 138, 1476–1482. DOI: 10.1016/j.foodchem.2012.11.031.
- Boonsumrej, S.; Chaiwanichsiri, S.; Tantratian, S.; Suzuki, T.; Takai, R. Effects of Freezing and Thawing on the Quality Changes of Tiger Shrimp (Penaeus Monodon) Frozen by Air-Blast and Cryogenic Freezing. Journal of Food Engineering 2007, 80, 292–299. DOI: 10.1016/j.jfoodeng.2006.04.059.
- Goncalves, A. A.; Ribeiro, J. L. D. Optimization of the Freezing Process of Red Shrimp (Pleoticus Muelleri) Previously Treated with Phosphates. International Journal of Refrigeration 2008, 31, 1134–1144. DOI: 10.1016/j.ijrefrig.2008.03.005.
- Anonymous. Fishery Yearbook in China in 2017; Chinese Agriculture Press: Beijing, 2017.
- Su, G.; Ramaswamy, H. S.; Zhu, S.; Yu, Y.; Hu, F.; Xu, M. Thermal Characterization and Ice Crystal Analysis in Pressure Shift Freezing of Different Muscle (Shrimp and Porcine Liver) versus Conventional Freezing Method. Innovative Food Science and Emerging Technologies 2014, 26, 40–50. DOI: 10.1016/j.ifset.2014.05.006.
- Yu, D.; Xu, Y.; Jiang, Q.; Xia, W. Effects of Chitosan Coating Combined with Essential Oils on Quality and Antioxidant Enzyme Activities of Grass Carp (Ctenopharyngodon Idellus) Fillets Stored at 4°C. International Journal of Food Science and Technology 2017, 52, 404–412. DOI: 10.1111/ijfs.13295.
- Benjakul, S.; Bauer, F. Biochemical and Physicochemical Changes in Catfish (Silurus Glanis Linne) Muscle as Influenced by Different Freeze–Thaw Cycles. Food Chemistry 2001, 72, 207–217. DOI: 10.1016/S0308-8146(00)00222-3.
- Yu, D.; Xu, Y.; Regenstein, J. M.; Xia, W.; Yang, F.; Jiang, Q.; Wang, B. The Effects of Edible Chitosan-Based Coatings on Flavor Quality of Raw Grass Carp (Ctenopharyngodon Idellus) Fillets during Refrigerated Storage. Food Chemistry 2018, 242, 412–420. DOI: 10.1016/j.foodchem.2017.09.037.
- Dai, Y.; Miao, J.; Yuan, S. Z.; Liu, Y.; Li, X. M.; Dai, R. T. Colour and Sarcoplasmic Protein Evaluation of Pork following Water Bath and Ohmic Cooking. Meat Science 2013, 93, 898–905. DOI: 10.1016/j.meatsci.2012.11.044.
- Pan, B. S.; Yeh, W. T. Biochemical and Morphological-Changes in Grass Shrimp (Penaeus-Monodon) Muscle following Freezing by Air Blast and Liquid-Nitrogen Methods. Journal of Food Biochemistry 1993, 17, 147–160. DOI: 10.1111/j.1745-4514.1993.tb00464.x.
- Bello, R. A.; Luft, J. H.; Pigott, G. M. Ultrastructural Study of Skeletal Fish Muscle after Freezing at Different Rates. Journal of Food Science 1982, 47, 1389–1394. DOI: 10.1111/jfds.1982.47.issue-5.
- Xia, X.; Kong, B.; Liu, J.; Diao, X.; Liu, Q. Influence of Different Thawing Methods on Physicochemical Changes and Protein Oxidation of Porcine Longissimus Muscle. LWT - Food Science and Technology 2012, 46, 280–286. DOI: 10.1016/j.lwt.2011.09.018.
- Liu, D.; Liang, L.; Xia, W.; Regenstein, J. M.; Zhou, P. Biochemical and Physical Changes of Grass Carp (Ctenopharyngodon Idella) Fillets Stored at −3 and 0°C. Food Chemistry 2013, 140, 105–114. DOI: 10.1016/j.foodchem.2013.02.034.
- Mohan, C. O.; Ravishankar, C. N.; Lalitha, K. V.; Srinivasa Gopal, T. K. Effect of Chitosan Edible Coating on the Quality of Double Filleted Indian Oil Sardine (Sardinella Longiceps) during Chilled Storage. Food Hydrocolloids 2012, 26, 167–174. DOI: 10.1016/j.foodhyd.2011.05.005.
- Abdollahi, M.; Rezaei, M.; Farzi, G. Influence of Chitosan/Clay Functional Bionanocomposite Activated with Rosemary Essential Oil on the Shelf Life of Fresh Silver Carp. International Journal of Food Science and Technology 2014, 49, 811–818. DOI: 10.1111/ijfs.12369.
- Gao, M.; Feng, L.; Jiang, T.; Zhu, J.; Fu, L.; Yuan, D.; Li, J. The Use of Rosemary Extract in Combination with Nisin to Extend the Shelf Life of Pompano (Trachinotus Ovatus) Fillet during Chilled Storage. Food Control 2014, 37, 1–8. DOI: 10.1016/j.foodcont.2013.09.010.
- Farajzadeh, F.; Motamedzadegan, A.; Shahidi, S.-A.; Hamzeh, S. The Effect of Chitosan-Gelatin Coating on the Quality of Shrimp (Litopenaeus Vannamei) under Refrigerated Condition. Food Control 2016, 67, 163–170. DOI: 10.1016/j.foodcont.2016.02.040.
- Yu, D. W.; Li, P. Y.; Xu, Y. S.; Jiang, Q. X.; Xia, W. S. Physicochemical, Microbiological, and Sensory Attributes of Chitosan-Coated Grass Carp (Ctenopharyngodon Idellus) Fillets Stored at 4°C. International Journal of Food Properties 2016, 20, 390–401. DOI: 10.1080/10942912.2016.1163267.
- Saito, T.; Arai, K.; Matsuyoshi, M. A New Method for Estimating the Freshness of Fish. Bulletin of the Japanese Society of Scientific Fisheries 1959, 24, 749–750. DOI: 10.2331/suisan.24.749.
- Ocaño-Higuera, V. M.; Maeda-Martínez, A. N.; Marquez-Ríos, E.; Canizales-Rodríguez, D. F.; Castillo-Yáñez, F. J.; Ruíz-Bustos, E.; Graciano-Verdugo, A. Z.; Plascencia-Jatomea, M. Freshness Assessment of Ray Fish Stored in Ice by Biochemical, Chemical and Physical Methods. Food Chemistry 2011, 125, 49–54. DOI: 10.1016/j.foodchem.2010.08.034.
- Badii, F.; Howell, N. K. Changes in the Texture and Structure of Cod and Had Dock Fllets during Frozen Storage. Food Hyrdocolloids 2002, 16, 313–319. DOI: 10.1016/S0268-005X(01)00104-7.
- Zhu, L. G.; Brewer, M. S. Discoloration of Fresh Pork as Related to Muscle and Display Conditions. Journal of Food Science 1998, 63, 763–767. DOI: 10.1111/jfds.1998.63.issue-5.
- Savagaon, K. A.; Sreenivasan, A. Activation Mechanism of Pre-Phenoloxidase in Lobster and Shrimp. Fish Technology 1978, 15, 49–55.