ABSTRACT
Stability of milk drinks could be improved by adding high methoxyl pectin. In this work, effect of the modified high methoxyl pectin (MHMP) on the fermented milk beverage (FMB) was investigated by the characteristics of adsorption, viscosity, rheological properties, particle size, zeta-potential (ζ-potential), microstructure, and phase behavior. The low concentration of MHMP (<0.2%, w/v) and final pH (<4.0) might easily cause the instability among the particles inducing from the bridging flocculation. The FMB system obviously had a good stability as the adding of 0.4% MHMP. Meanwhile, the particle size had a slight downward trend with decreasing the value of pH. The increase of MHMP concentration could increase the properties of viscosity and ζ-potential. However, the ζ-potential of the system had a decreasing trend by reducing the pH of the FMB with the same concentration of MHMP. The microstructure of the FMB was thick and dense compared with the sample without MHMP. As the concentration of MHMP was above 0.4%, the FMB system presented the uniform dispersion and only slight stratification water occurred for the phase behavior. Besides, it was also indicated that most part of MHMP was not adsorbed onto the casein micelles but was involved in a network with MHMP/casein complexes in the FMB.
Introduction
During the acidified process of milk drinks, a firm space-filling network of the aggregated casein micelles was created. The formation of this network was induced by the weakening electrostatic interactions and steric forces between the casein micelles.[Citation1,Citation2] To prevent the gel/network formation, the stabilizers were required that could avoid casein micelles from aggregation. High methoxyl pectin (HMP) was widely applied to stabilize the acidified milk drinks, where it prevented the problem of sedimentation caused by the flocculation of casein micelles in the system.[Citation3,Citation4] Pectin has a chain structure, α-(1→4)-linked d-galacturonic acid units, interrupted in a few places by a comparatively small amount of so-called rhamnogalacturonan with neutral polysaccharide side chains. Some of the carboxyl groups are methyl esterified, and pectin with more than 50% of esterified carboxyl groups is defined as HMP.[Citation3,Citation5,Citation6] The stability of the fermented milk beverage (FMB) was substantially the problem of how to prevent the extensive aggregation of the casein micelles, which could lead to a macroscopic gel. Pectin was introduced that could increase the steric repulsion between the casein micelles and prevent the flocculation. Casein micelles in the normal milk were generally assumed to stay in the suspended state due to the steric repulsive interactions among the micelles. As the acidification of milk, the value of pH was around 4.0 and the native stabilization of casein micelles failed. The separation of calcium phosphate from casein micelles could appear as adjusting the acidity. The acid coagulation formed as the pH reduced to the isoelectric point. During acidification, colloidal calcium phosphate could dramatically disassociate from casein micelles. That change was also considered to be related to the collapse of the extended conformation of k-casein chains.[Citation7,Citation8] These chains protruded from the surface of the casein micelle in order to maximize their entropy as pH value was about 6.7.[Citation9] The same tendency to maximize k-casein chains resulted in the micelles to have a repulsive mutual interaction because overlap of k-casein chains of the neighboring micelles could induce a reduction in entropy of the chains.[Citation2,Citation10] This phenomenon was named as steric stabilization.[Citation2,Citation10,Citation11]
The stabilization by pectin was hypothesized to involve the blockwise distribution of the charges along the casein micelles. The adsorption of pectin chain on the surface of casein micelles would take place only at the charged blocks, while the uncharged stretches in between the form entropy-rich loops, which extended into the solution of the system.
At the low pH, these loops could offer a repulsive interaction between the casein micelles as the same way as k-casein chains at pH 6.7. In the acidic milk drink, it showed that hydrophilic colloid was added to the system before adjusting acidity. It could interact with the denatured casein during the process of acidification to achieve a stable system. However, it was also hypothesized that hydrophilic colloid was still able to play a stabilizing role to the system with the denatured casein. Therefore, it was necessary to take acidic milk drink as research objects and study the mechanism between the HMP and the denatured casein.
The studies of how pectin interacted with the casein aggregates in acidic milk drinks had been investigated in the researches.[Citation12–Citation14] It had been shown that pectin adsorbed via the electrostatic interactions in the diluted acidified milk systems.[Citation15,Citation16] The adsorption occurred at the value of pH was less than or equal to 5.0. Different theories had been proposed for the specific stabilizing mechanism. Steric stabilization could be responsible, where part of the pectin adsorbed to the casein aggregate surface and the others protruded from the surface as loops and dangling the tails with the casein micelles. The entropy of the protruding pectin chains could be assumed to contribute to the stability due to the introduction of steric repulsion among the casein aggregates.[Citation17]
The phenomenon of precipitation in the FMB was the critical bottleneck. The theoretical research on how different kinds of pectin stabilized the FMB was worth exploring. Besides, pH and the relative concentration of a biopolymer were the significant factors affecting the electrostatic protein–pectin interactions.[Citation18–Citation21] The aim of the present work was to study the interaction between the special modified HMP (MHMP) and casein and to research the stability of the MHMP in the FMB system.
Materials and methods
Materials
The special MHMP was provided from Weiyi Chemical Co. Ltd. (Suzhou, China), which was commercial and widely used in China. The starter was the mixed bacteria YF-3331 consisting of Lactobacillus delbrueckii subsp. bulgaricus and Streptococcus thermophilus. The skim milk powder was obtained from Fonterra Co-operative Group (Wellington, New Zealand).
Preparation of the FMB
The FMB was prepared as the method of the previous study with some modification.[Citation22] Food grade sugar (8%, w/v) was dispersed to the dissolved MHMP solution. After shearing breaking, the fermented milk and the distilled water were added to MHMP solution with the certain concentrations of MHMP ranged from 0% to 0.6% (w/v). The pH of milk sample was adjusted with the food grade citric acid (10%, w/v). The final sample was prepared containing 1% (w/v) protein. The sample was preheated to 65°C before the homogeneous at 25 Mpa. It was pasteurized at 95°C for 15 min. After cooling, the FMB sample was stored at 4°C.
MHMP adsorption and viscosity in the FMB
The FMB without MHMP was centrifuged at 25,000g for 1 h (Biofuge Stratos, Heraeus, Co., Germany). The supernatant was used as solvent to prepare the samples containing various amounts of MHMP (0–0.6%, w/v). The adsorption of the MHMP in the samples was adjusted at pH 4.0. The viscosity of the supernatant samples including different MHMP concentrations was measured with AR-G2 rotational rheometer (AV, Co., America) at 25°C, with a diameter of 40 mm, one taper stainless steel plate and the gap of 1 mm.[Citation22] The adsorption of pectin in the samples was adjusted at pH 4.0. The FMB was put in the Brookfield viscometer (DV-II+Pro, Brookfield, Co., America) at 25°C. The data displayed on the viscometer after 30 s were recorded. Before every measurement, the viscometer was cleaned and recalibrated.
Dynamic rheology
The FMB sample was put in the AR-G2 rheometer to test the dynamic viscoelasticity. The concentration of MHMP and sugar was 0.4% (w/v) and 8% (w/v), respectively. Value of pH was 4.2. The samples were let alone for 2 min to release the residual stress. After experiencing precut at a shear rate of 0.1 s−1 for 3 min, the measurements were performed to test the storage shear modulus (G′) and the loss shear modulus (G″) at 25°C.[Citation22] The scanning field ranges from 0 to 3600 s. The determination was carried out at the range of the linear viscoelastic region.
Distribution of particle size
The distribution of particle size in the FMB was measured using a Malvern Master sizer 2000 (Malvern instruments Ltd., Worcs., England). The instrument was set up according to the following parameters: refractive index of the particles of 1.50, a sample adsorption of 0.01, and a refractive index of the solvent 1.330.[Citation14,Citation22] Measurements were performed at 25°C with stirring speed 2800 r min−1 and the ultrasonic cleaning was performed for 70 s. The FMB sample was diluted with 10 times volume of deionized water.
ζ-potential
Before the measurements, the sample was blended with 10 times volume of deionized water. The value of pH was adjusted by 10% citric acid. Changes of zeta-potential (ζ-potential) were tracked using nano ZS laser particle size analyzer (Malvern Instruments Ltd., UK), after absorbing 1 mL into the sample pool. Experiments were carried out at 25°C and the refractive index was 1.55.[Citation22]
Scanning electron microscopy
The FMB sample was tiled in vessel and cooled to −18°C for 1 h in the freezer and freeze-dried at −52°C for 24 h, with vacuum degree of 5.9 Pa. After the treatment, the small wafer was achieved. The size was 3 mm×3 mm and the thickness was 1.5 mm. It was sputtered by vacuum ion to obtain a platinum film and observed with the instrument of the scanning electron microscopy (SEM) (INCAPentaFET×3, INCA Oxford). The observation was performed at 25°C. The test distance was 6.8 nm. The accelerating voltage was 5 kV.
Phase behavior of the FMB
MHMP was added into the FMB at the concentrations of 0.2–0.6%. The FMB sample without MHMP was as the reference. Value of pH was adjusted to 3.8, 4.0, 4.2 with the 10% citric acid solution, respectively. The sample was assembled in a stoppered glass tube and stored in the freezer at 4°C for 7 days for the observation.
Statistical analysis
Experimental data were statistically analyzed using PASWStatistics18.0 Software (SPSS Inc., USA). One-way and univariate ANOVA were applied to examine the effects of different treatments. Data were expressed as mean value ± standard deviation. Duncan’s multiple range test was performed for post hoc multiple comparisons with the level of significance set at P < 0.05.
Results and discussion
Adsorption of MHMP in the FMB
The relationship between the MHMP concentrations and the viscosity was described in ). To obtain the MHMP concentration in the serum of different samples, a calibration curve was made according to the viscosity of the serum as a function of the MHMP concentration and the result was shown in ). A linear function of the logarithm of the concentration was used for the interpolation according to the method of Tromp et al.[Citation12] The solid content, regarded as the section that MHMP was not adsorbed onto the casein gel particles, was separated from the yellowish supernatant, accounted as non-adsorbed MHMP.
Figure 1. (a) Correlation curve for the viscosity of the serum with different concentration of MHMP in FMB. (b) The adsorption of MHMP in the FMB. MHMP: Modified high methoxyl pectin; FMB: fermented milk beverage.
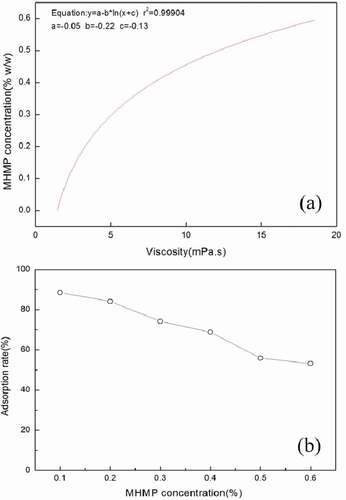
It could be found that as the concentration of MHMP was inferior to 0.2%, non-adsorbed MHMP in the supernatant was low and the adsorption rate was more than 80%. In the FMB system, less amount of MHMP contained in the supernatant reasoned from the concentration of MHMP was less than 0.2%. Then, the system was in an unstable state and prone to casein flocculation. Up to 0.3% MHMP, the adsorption rate decrease could be considered the MHMP adsorption reached to the saturation in the system and it was more stable prior to the addition below 0.2% MHMP.
The adsorption amount of MHMP in the FMB was shown in . It could be found that increasing the addition amount of MHMP in the acidic milk system resulted in the increase of adsorption amount. Compared to the lower concentration, the adsorption amount of MHMP had a significant increase as the addition amount was above 0.4% in the FMB (P < 0.05). However, there was no significantly different in the FMB as the adsorption amount was from 0.4% to 0.6% (P > 0.05) and it was almost keeping in the level of (0.27–0.31 g)/100 g. It could be inferred that increasing the amount of MHMP could promote the adsorption amount on the surface of the casein particles.
Table 1. The adsorption of MHMP in the fermented milk beverage.
Effect of MHMP concentration and value of pH on the viscosity
The Brookfield viscosity of the FMB stabilized by the MHMP was shown in . Large casein aggregates were observed in the unstable samples at the low MHMP concentration (below 0.2%). The Brookfield viscosity could not be measured in these unstable FMB that increased from 6.0 to 7.8 mPa s with increasing the concentration of MHMP. As the concentration of MHMP was from 0.3% to 0.6%, the viscosity of the system increased from 11.4 to 30.4 mPa s. It could also be found that the viscosity of the FMB system had a slight trend to decrease with reducing the value of pH, especially in the concentration of 0.6%. Increasing the concentration of MHMP could improve the viscosity as the value of pH decreased from 4.2 to 3.8. These results might be attributed to two aspects. First, the charge of the casein micelle was positive during acidification. Lots of MHMP was adsorbed onto the casein micelles or the particles of milk gel by electrosorption.[Citation16,Citation17] The non-adsorbed MHMP decreased. Those influenced the stabilization of milk protein in the FMB system. Second, the conformation of MHMP chains was compacted with reducing the value of pH and that decreased the viscosity.
Dynamic rheology of the FMB with different concentration of MHMP
showed the time scanning curve of elastic modulus (G′) and loss modulus (G″) for the FMB. During the test, changes of G′ were found due to the formation and the rupture of the molecular structure in the network system. The smaller the fluctuation of G′, the better the stability of the system. As could be seen in , G′ was significantly higher than the loss modulus G″. The level of floating G′ was only in a small range, which indicated that the system obviously had a stable nature and a good stability as the adding of 0.4% MHMP. In the stable system, MHMP was supersaturated. A small portion of MHMP adsorbed on the surface of casein gel provided the steric hindrance, and the remaining no-absorbed portion could be determined by measuring the dynamic viscoelasticity to analyze whether they could play a role in stabilizing system.[Citation12,Citation23] There might exist a weak gel network structure in the FMB with MHMP. It could be inferred that non-adsorbed MHMP might enable to adsorb easily on the surface of gel particles, which could form a spatial network structure with MHMP/casein complex to delay the casein precipitation and to stabilize the FMB.
Effect of MHMP concentration and value of pH on the particle size
The evolution of particle size of the FMB as a function of pH was shown in . Change in the particle size of the FMB was irregular. As the concentration of MHMP increased to 0.2%, the flocculation was observed as the value of pH was below 4.0. That probably reasoned from the positive charge of casein increased and the MHMP was not sufficient to wrap the casein.[Citation19,Citation24] As the concentration of MHMP was above 0.4%, the particle size had a slight downward trend with decreasing the value of pH. The reason might be the MHMP was supersaturated and the decrease in the pH could reduce the negative charge the MHMP linear. The molecular force among the MHMP was weakened leading to the collapse in adsorption layer and the particle size reduced.
Effect of MHMP concentration and value of pH on ζ-potential
ζ-potential, calculated from the measured electrophoretic mobility of particles in the FMB, was shown as a function of pH (pH was from 3.8 to 4.2) in . During the gradually acidification, the net charge of casein changed from negative to positive. Consequently, the ζ-potential of the aggregates in the FMB without MHMP was positive.[Citation16] The value of pH in the system was less than the isoelectric point of casein in milk. Based on that, the surface of the casein was positive and the potential of the other test groups was negative. Therefore, taking the absolute value of ζ-potential to study, the greater the absolute value of ζ-potential was, the more stable the system was. The increase in the absolute value of the ζ-potential was observed in the FMB with the addition of MHMP (0.2–0.6%). It might be presumably cause by the adsorption of negatively charged MHMP molecules onto the positively charged protein aggregates. On the other hand, the ζ-potential of the system had a decreasing trend by reducing the pH of the FMB with the same concentration of MHMP. The decrease of pH increased the positive charge on the surface of the casein micelles. These changes in the charge distribution made the electrostatic repulsion small, which caused the decreasing of ζ-potential. As the concentration of MHMP exceeded 0.4%, the system was relatively stable, it was found that ζ-potential had not depended on the change of pH and remained substantially unaltered.
Microstructure
The microstructure of the FMB with different concentration of MHMP at pH 4.0 was shown using the SEM (). As the magnification increased to 10,000 times, the microstructure of protein network could be clearly observed. It was shown that the microstructure of casein network was more significantly thick and dense compared with the sample without the addition of MHMP. The gap between the apertures also reduced. With increasing the concentration of MHMP, the cross linking degree of network structure became more tight and more fortified. In addition, it could be observed that when the concentration of MHMP increased to 0.6%, the excess MHMP was intertwined with the MHMP/casein gel network and gave the multilayer parcel to the protein. Under the acidic conditions, the denaturation and flocculation of casein resulted in the skeleton and the gap among the skeleton just filled with water. The clusters of casein formed gel network structure. These clusters were composed by the different sizes of the spherical piling casein particles, hardly constituting the continuous chain structure. It could be indicated that the substantial of the system at the acidic conditions was relatively low.
Figure 6. SEM micrographs of the FMB with MHMP. (A) without MHMP (B) 0.2% MHMP (C) 0.4% MHMP (D) 0.6% MHMP at scale 1000× (a) without MHMP (b) 0.2% MHMP (c) 0.4% MHMP (d) 0.6% MHMP at scale 10000×.
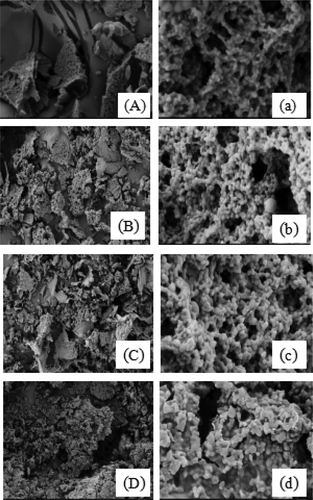
The microstructure of the FMB with a series of pH (from 3.4 to 4.2, gradient: 0.4, the concentration of MHMP was 0.4%) was observed in . As pH was 4.2, the gap was relatively large and the structure of the FMB was relatively loose. It was probably due to that the binding between MHMP and casein was not strong, resulting in the more loose network structure. With decreasing the value of pH, the system brought more positive charge, which might cause the MHMP adsorbed on the casein solidly. As pH was 3.8, the MHMP combined with casein closely. As the pH decreased to 3.4, there was a phenomenon that the network become incomplete, uneven, and looser. It could be speculated that the adsorption layer collapsed.[Citation25]
Phase behavior in the FMB
The phase behavior affected by the concentration of MHMP and the value of pH was shown in . It could be found that the phase behavior changed with the concentration of MHMP. There was a total separation between the casein aggregates and the serum in the FMB without the addition of MHMP after the storage. The addition of MHMP could make the supernatant decrease. When the concentration of MHMP was above 0.4%, the performance of the system presented the uniform dispersion and only slight stratification water occurred in ). As the concentration of MHMP was 0.2%, the apparent supernatant increased in ). It was considered that the low value of pH likely to cause the whey separation, which was not conducive to the stability of acidic milk drink. Nevertheless, the value of pH could not play the critical role on the stability as the concentration of MHMP reached to 0.6% in ). The reason might be that there was enough polysaccharide to completely cover the protein particles. MHMP required to saturate the surface of protein particles and that was referred to the full coverage. Based on that, the dispersion of protein particle was stable. With the final acidity of beverage decreased, the MHMP need to avoid the phase separation and more MHMP was demanded to stabilize the system in the low pH conditions. The adsorption of MHMP occurred to form the adsorption layer to provide the steric hindrance, which resulted from the positive charges carried by the surface of casein micelles.
Conclusion
MHMP could maintain the system stability in the FMB. The adsorption rate decreased with increasing the concentration of MHMP. The adsorbed amount of MHMP increased to (0.27–0.31) g/100 g as the concentration of MHMP was from 0.4% to 0.6%. The system obviously had a stable dynamic viscoelasticity as adding 0.4% MHMP. The low concentration of MHMP (<0.2%) and final pH (<4.0) might easily cause the instability between the particles inducing from the bridging flocculation. The increase of MHMP concentration could increase the properties of viscosity and ζ-potential. As the concentration of MHMP was above 0.4%, there was no obvious stratification, although the value of pH decreased from 3.8 to 4.2. It could be suggested that reducing pH could lead to the whey separation and that also could enhance the interaction between MHMP and casein to make the dispersion of the FMB uniform.
Additional information
Funding
References
- Janhoj, T.; Frost, B. M.; Ipsen, R. Sensory and Rheological Characterization of Acidified Milk Drinks. Food Hydrocolloids 2008, 22, 798–806. DOI: 10.1016/j.foodhyd.2007.03.006.
- Zhao, H.; Zhou, F.; Peng, W.; Zheng, J.; Dziugan, P.; Zhang, B. The Effects of Carrageenan on Stability of Arachin and the Interactions between Them. Food Hydrocolloids 2015, 43, 763–768. DOI: 10.1016/j.foodhyd.2014.08.006.
- Daas, P. J. H.; Meyer-Hansen, K.; Schols, H. A.; De Ruiter, A.; Voragen, A. G. J. Investigation of the Non-Esterified Galacturonic Acid Distribution in Pectin with Endopolygalacturonase. Carbohydrate Research 1999, 318, 135–145. DOI: 10.1016/S0008-6215(99)00093-2.
- Fang, Y.; Al-Assaf, S.; Phillips, G. O.; Nishinari, K.; Funami, T.; Williams, P. A. Binding Behavior of Calcium to Polyuronates: Comparison of Pectin with Alginate. Carbohydrate Polymers 2008, 72, 334–341. DOI: 10.1016/j.carbpol.2007.08.021.
- Voragen, F.; Beldman, G.; Scholc, H. A. Chemistry and Enzymology of Pectins. In Advanced Dietary Fibre Technology; McCleary, B. V., Prosky, L., Eds.; Blackwell Science Ltd: Oxford, 2001. pp 379–398.
- Farah, N.; Masoodi, F. A.; Sajad, A. R.; Wani, S. M.; Adil, G. Emerging Concepts in the Nutraceutical and Functional Properties of Pectin - A Review. Carbohydrate Polymers 2017, 168, 227–239. DOI: 10.1016/j.carbpol.2017.03.058.
- Dalgleish, D. G.;. Casein Micelles as Colloids: Surface Structures and Stabilities. Journal of Dairy Science 1998, 81, 3013–3018. DOI: 10.3168/jds.S0022-0302(98)75865-5.
- De Kruif, C. G.;. Supra-Aggregates of Casein Micelles as a Prelude to Coagulation. Journal of Dairy Science 1998, 81, 3019–3028. DOI: 10.3168/jds.S0022-0302(98)75866-7.
- Roach, A.; Harte, F. Disruption and Sedimentation of Casein Micelles and Casein Micelle Isolates under High-Pressure Homogenization. Innovative Food Science & Emerging Technologies 2008, 9, 1–8. DOI: 10.1016/j.ifset.2007.03.027.
- Dalgleish, D. G.; Corredig, M. The Structure of the Casein Micelle of Milk and Its Changes during Processing. Annual Review of Food Science and Technology 2012, 3, 449–467. DOI: 10.1146/annurev-food-022811-101214.
- Schmitt, C.; Turgeon, S. L. Protein/Polysaccharide Complexes and Coacervates in Food Systems. Advances in Colloid and Interface Science 2011, 167, 63–70. DOI: 10.1016/j.cis.2010.10.001.
- Tromp, R. H.; De Kruif, C. G.; Van Eijk, M.; Rolin, C. On the Mechanism of Stabilisation of Acidified Milk Drinks by Pectin. Food Hydrocolloids 2004, 18, 565–572. DOI: 10.1016/j.foodhyd.2003.09.005.
- Sejersen, T. M.; Salomonsen, T.; Ipsen, R.; Clark, R.; Rolin, C.; Engelsen, S. B. Zeta Potential of Pectin-Stabilised Casein Aggregates in Acidified Milk Drinks. International Dairy Journal 2007, 17, 302–307. DOI: 10.1016/j.idairyj.2006.03.003.
- Jensen, S.; Rolin, C.; Ipsen, R. Stabilisation of Acidified Skimmed Milk with HM Pectin. Food Hydrocolloids 2010, 24, 291–299. DOI: 10.1016/j.foodhyd.2009.10.004.
- Parke, A.; Boulenguer, P.; Kravtchenko, T. P. Effect of the Addition of High Methoxy Pectin on the Rheology and Colloidal Stability of Acid Milk Drinks. International Conference and Industrial Exhibition on Food Hydrocolloid. 1994, 307–312.
- Tuinier, R.; Rolin, C.; De Kruif, C. G. Electrosorption of Pectin onto Casein Micelles. Biomacromolecules 2002, 3, 632–638. DOI: 10.1021/bm025530x.
- Pereyra, R.; Schmidt, K. A. Interaction and Stabilization of Acidified Casein Dispersions with Low and High Methoxyl Pectins. Journal of Agricultural and Food Chemistry 1997, 45, 3448–3451. DOI: 10.1021/jf970198f.
- Syrbe, A.; Bauer, W. J.; Klostermeyer, H. Polymer Science Concepts in Dairy Systems - An Overview of Milk Protein and Food Hydrocolloid Interaction. International Dairy Journal 1998, 8, 179–193. DOI: 10.1016/S0958-6946(98)00041-7.
- Maroziene, A.; De Kruif, C. G. Interaction of Pectin and Casein Micelles. Food Hydrocolloids 2000, 14, 391–394. DOI: 10.1016/S0268-005X(00)00019-9.
- Phillips, G. O.; Williams, P. A.; Wedlock, D. J. Gums and Stabilizers for the Food Industry; IRL Press: Oxford, 2002; 157–175 pp.
- Ru, Q.; Wang, Y.; Lee, J.; Ding, Y.; Huang, Q. Turbidity and Rheological Properties of Bovine Serum Albumin/Pectin Coacervates: Effect of Salt Concentration and Initial Protein/Polysaccharide Ratio. Carbohydrate Polymers 2012, 88, 838–846. DOI: 10.1016/j.carbpol.2012.01.019.
- Li, Y. H.; He, S. S.; Meng, Y. C.; Chen, J.; Wang, W. J. Studies on the Stability of Fermented Milk Beverage by the Addition of Whey Protein Concentrate. Journal of Food Process Engineering 2016, 39, 453–461. DOI: 10.1111/jfpe.2016.39.issue-5.
- Laurent, M. A.; Boulenguer, P. Stabilization Mechanism of Acid Dairy Drinks (ADD) Induced by Pectin. Food Hydrocolloids 2003, 17, 445–454. DOI: 10.1016/S0268-005X(03)00028-6.
- Kim, Y.; Wicker, L. Charge Domain of Modified Pectins Influence Interaction with Acidified Caseins. Food Hydrocolloids 2011, 25, 419–425. DOI: 10.1016/j.foodhyd.2010.07.011.
- Sedlmeyer, F.; Brack, M.; Rademacher, B.; Kulozik, U. Effect of Protein Composition and Homogenisation on the Stability of Acidified Milk Drinks. International Dairy Journal 2004, 14, 331–336. DOI: 10.1016/j.idairyj.2003.09.009.