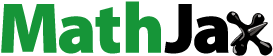
ABSTRACT
The effect of epichlorohydrin (0.5%) as a cross-linking agent on physicochemical, rheological, morphological, and in vitro digestion properties of pearl millet starches from different cultivars was studied. Degree of cross-linking (DC) ranged between 40.61% and 89.75%, lower values of DC were observed for cv.HC-10 and cv.HHB-67 whereas higher values were observed for cv.HHB-223 and cv.GHB-732. Cross-linked starch from cv.GHB-732 showed the lowest amylose content, swelling power, and solubility as compared to other cultivars. Rheological properties of starches during heating showed their elastic behaviour. G′ value was much higher than the G′′ value at all frequency values for starch pastes. Plots of shear stress (σ) versus shear rate (γ̇) data for cross-linked starch pastes were fitted to Herschel-Bulkley model and yield stress (σo), flow behaviour index (n), and consistency index (K) were evaluated. Scanning electron micrographs (SEM) investigations revealed that cross-linked starch granules had slightly rough surfaceand grooves with slight fragmentations. Readily digestible starch (RDS) of cross-linked starches varied from 46.1% to 50.6%, cv.GHB-732 had the lowest value. Slowly digestible starch (SDS) and resistant starch (RS) content of cross-linked starches ranged from 34.5% to 36.4% and 13.6% to 19.4%, respectively. cv.HC-10 had the highest value for SDS content while the highest RS content was observed for cv.GHB-732. In comparison to native starches, cross-linked modification decreased SDS and RDS content whereas RS content was increased.
Introduction
Native starches have limited use due to high paste temperature, viscosity, opaqueness, higher retrogradation, and syneresis of their gels.[Citation1] During processing, texture and appearance of the product is altered due to retrogradation, so to overcome these undesirable changes starch needs to be modified.[Citation2] Starch modification is mostly achieved through derivatization, e.g., by esterification, etherification, cross-linking, and decomposition (acid or enzymatic hydrolysis and oxidization of starch) or physical treatment such as heat and moisture.[Citation3] Native starch can be modified with different cross-linking agents such as epichlorohydrin, sodium trimetaphosphate, sodium tripolyphosphate, and POCl3.[Citation4] During starch modification with epichlorohydrin (EPI), there is a formation of ether linkages between the cross-links and the hydroxyl groups in the starch, which gives distarch glycerols of high mechanical strength and resistance to pH.[Citation5] Cross-linking of starch is affected by different factors such as starch source, cross-linking reagent concentration, extent of substitution, pH, reaction time, and temperature.[Citation6] For stabilized granular structure and restricted swelling, cross-linked starch is most commonly used in food industry.[Citation4]
Pearl millet (Pennisetum glaucum L.), one of the important type of millets, belongs to the family gramineae and it is widely cultivated around the world primarily in India and Africa. Suma and Urooj[Citation7] reported starch content in pearl millet in the range from 62.8% to 70.5% among different Indian genotypes. Due to decreased water supplies, decreased area under corn cultivation, adverse salinity, low cost of growing pearl millet crop, starch from pearl millet needs to be explored more so that it can become better substitute than other conventional starch sources. Many researchers have investigated the properties of cross-linked starches from different sources, including corn,[Citation8,Citation9] wheat,[Citation9,Citation10] potato,[Citation11,Citation12] oat[Citation13], and rice.[Citation14] Currently, there is little information available on the properties of chemically modified pearl millet starch that can be used further in many food applications. Hence, the objective of present study is to examine the effect of epiclorohydrin as a cross-linking agent on physicochemical, morphological, rheological, and in vitro digestibility properties of starch from pearl millet cultivars commonly grown in India.
Material and methods
Materials
Pearl millet cultivars (cv.) (HC-10, HHB-67, HHB-223, HHB-226, and W-445) were procured from Chaudhary Charan Singh Haryana Agriculture University, Hissar, India whereas cv.GHB-732 was procured from Pearl Millet Research Station, Jamnagar, Gujarat, India.
Starch isolation and modification with epichlorohydrin
Starch was isolated from pearl millet grains by following the method described by Sandhu and Singh.[Citation15] Starch was modified by following the method described by Wurtzburg.[Citation16] Pearl millet starch (100 g, d.w.b.) was suspended in 150 ml of 0.5% NaOH. Epichlorohydrin (0.5% v/w, d.w.b. respectively) was slowly added with agitation on magnetic agitator (Spinot, India). After agitating for 5 h at room temperature, reaction was terminated by adjustment of pH of suspension to 5.0 with 1 M HCl. Starch was centrifuged (3,000 rpm for 5 min) and washed with distilled water. Washing with water and centrifugation steps were repeated until no gelatinous lumps were formed on starch surface. Thereupon, starch was neutralized with 1 M NaOH, centrifuged once more and air-dried.
Degree of cross-linking and amylose content
The relative degrees of cross-linking were calculated as described by Chatakanonda et al.[Citation17]
The amylose content of starch was determined by following the method described by Williams et al.[Citation18]
Swelling power, solubility, and pasting properties
The swelling power and solubility of starches were determined by following the method described by Leach et al.[Citation19] The pasting properties of pearl millet starch were determined using in-build starch cell in a Modular Compact Rheometer (Anton Paar, Austria). Starch slurries (1.5 g starch in 13.5 g distilled water) were held at 50°C for 1 min and then heated from 50°C to 95°C at a heating rate of 11°C/min, held for 2.0 min, cooled to 50°C at the same rate and again held at 50°C for 2 min. Each sample was analysed in triplicate. Peak viscosity (PV), breakdown (BV), setback (SV), final viscosity (FV), and pasting temperature (PT) were obtained from the pasting graph.
Dynamic properties
A small amplitude oscillatory rheological measurement was made for different starches with a Modular Compact Rheometer (Anton Paar, Austria) equipped with parallel plate system (4 cm diameter). The gap size was set at 1,000 μm. The strain and frequency were set at 2% and 10 rad/s, respectively, for all determinations. The dynamic rheological properties, such as storage modulus (G′), loss modulus (G″), and loss factor (tan δ) were determined for starches from different cultivars. Starch suspensions of 15% (w/w) concentration were loaded onto the ram of the rheometer and covered with a thin layer of low-density silicon oil (to minimize evaporation losses). The starch samples were subjected to temperature sweep testing and were heated from 45°C to 95°C at the rate of 2°C/min.[Citation20] Frequency sweep measurements were carried out by following the method described by Kaur and Singh[Citation21] with slight modification. For frequency sweep measurement, the starch slurry (15%, w/w) was prepared, manually stirred and then heated at 90°C in a water bath (Brookefield, TC-202, USA) followed by 3 min of stirring. The sample was allowed to cool at room temperature and then loaded on the ram of rheometer. Frequency sweep tests from 0.1 to 100 rad/s were performed at 25°C. The storage modulus (G′), loss modulus (G′′), and loss tangent (tan δ) were derived at 25°C.
Steady shear measurement
Steady shear properties were determined by following the method described by Park et al.[Citation22] with slight modifications. The sample preparation as described in frequency sweep measurement method was followed. The sample (10%) was sheared continuously from 1 to 1,000 s−1. In order to describe the variation in the rheological properties of samples under steady shear, the data was fitted to Herschel-Bulkley model:
where σ is the shear stress (Pa), σo is the yield stress, γ̇ is the shear rate (s−1), K is the consistency index (Pa.sn), and n is the flow behaviour index (dimensionless).
Morphological properties
Morphological properties were determined by using scanning electron microscope (Model EVOLS10 ZEISS, Oberkochen, Germany). Starch samples were suspended in ethanol to obtain a 1% suspension. One drop of the starch-ethanol solution was applied to an aluminium stub using double-sided adhesive tape. An accelerating potential of 5 kV was used during micrography.
In vitro starch digestibility
In vitro starch digestibility was analysed following the method described by Englyst et al.[Citation23] as modified by Chung et al.[Citation24] Porcine pancreatic alpha-amylase (No. 7545, Sigma-Aldrich, St. Louis, MO) and amyloglucosidase (No. 9913, Sigma-Aldrich) (3.89 g) was dispersed in water (25.7 ml) and centrifuged for 10 min at 2500 g, and 18.7 ml of supernatant was collected. Amyloglucosidase (No. 9913, Sigma-Aldrich) (1 ml) and deionized water (2 ml) were added to enzyme solution. The solution was freshly prepared for the digestion analysis.
Aliquots of guar gum (10 ml, 5 g/l) and sodium acetate (5 ml, 0.5 M) were added to the starch samples (0.5 g, db) in a test tube. Seven glass balls (10 mm diameter) and 5 ml of enzyme solution were then added to each tube, following the incubation in a water bath (37°C) with agitation (170 rpm). Aliquots (0.5 ml) were taken at intervals and mixed with 4 ml of 80% ethanol, and the glucose contents in the mixture were measured using glucose oxidase and peroxidase assay kits (No. GAGO-20, Sigma-Aldrich). The total starch content in the starch samples was measured according to Englyst et al.[Citation23] The starch classification based on its digestibility was: readily digestible starch (RDS) as the starch that was hydrolyzed within 20 min of incubation, resistant starch (RS) as the starch not hydrolyzed with 120 min, and slowly digestible starch (SDS) as the starch digested during the period between 20 and 120 min.
Statistical analysis
The data reported in the tables were carried out in triplicate and they were subjected to one-way analysis of variance (ANOVA) using Minitab Statistical Software version 15 (Minitab, Inc., State College, USA).
Results and discussion
Degree of cross-linking, amylose content, swelling power, and solubility
Degree of cross-linking (DC) of cross-linked starches from different pearl millet cultivars varied from 40.6% to 89.7%, the highest and the lowest was observed for cv.GHB-732 and cv.HHB-67, respectively (). Cv.GHB-223 and cv.GHB-732 showed non-significant (p < 0.05) difference in DC. Various workers have reported DC values ranging from 51.3% to 99.1% for corn starches,[Citation8] 60.1% for potato,[Citation25] 35.9% to 82.4% for cassava starch[Citation5], and 7.17 to 87.29% for wheat starches.[Citation10] Amylose content of cross-linked starches ranged from 6.2% to 8.5% (), the values observed were lower than those observed for their counterpart native starches. The amylose content of native pearl millet starches has already been reported in our earlier publication (values varied from 13.6% to 18.1%).[Citation26] These findings are consistent with previous study reported by Sukhija et al.[Citation27], which reports that the introduction of phosphate groups in cross-linked starches result in strengthening of starch structure and lesser degradation of amylose, thereby resulting in decrease in amylose content.
Table 1. Degree of cross-linking (DC), amylose content (%), swelling power (g/g), and solubility (%) of cross-linked starches.
Swelling power (SP) of cross-linked starches varied from 6.72 to 7.64 (g/g), cv.HHB-223 had the highest value (). For native counterpart starches, the SP ranged from 14.1 to 17.9 g/g, respectively [Citation26] (Data reported in our earlier publication). Cross-linked modification alter the SP of modified starches, the observed values were lower than their counterpart native starches. These results are in agreement with previous results reported by Singh and Nath,[Citation28] they concluded that decrease may be due to formation of more gelly mass that hinders the penetration of water into starch that reduces the SP of cross-linked starch. The solubility of cross-linked starches significantly (p < 0.05) varied among cultivars and ranged from 3.4% to 9.0%, the highest for cv.HC-10 and the lowest was observed for cv.GHB-732 (). Solubility of native starches from different cultivars ranged from 10.4% to 16.2%[Citation26] (Data reported in our earlier publication). Solubility of modified starches decreased as compared to their native counterparts. Similar decrease in solubility of modified corn starch was found by Kurakake et al.[Citation29] The cross-linking modification increased the density of cross-links in the starch structure by which less disintegration of starch granules during gelatinization occurs, this decreases the solubility of cross-linked starch.[Citation5]
Pasting properties
Significant (p < 0.05) differences in pasting properties among different cross-linked starches were observed ( & ). Peak viscosity (PV) of modified starches varied from 299 to 1164 mPa.s, the highest and the lowest values were observed for cv.HHB-67 and cv.HHB-223, respectively. PV is regarded as the maximum viscosity attained by the sample and tendency of starch granules to swell freely before physical breakdown.[Citation30] DC affects PV of cross-linked starch pastes, lower DC results in higher PV and vice-versa. [Citation5,Citation10] Breakdown viscosity (BV) of modified starches ranged from 15 to 470 mPa.s, cv.GHB-732 had the lowest value, which indicates that it is more stable during shearing at high temperature as compared to modified starches from different cultivars. The decrease in BV of the modified starches could be attributed to the formation of cross-links between starch molecules which strengthens the swollen granules against breakage under conditions of high temperature and shear.[Citation5] Setback viscosity (SV) of starches varied from 50 to 152 mPa.s. PV, BV, TV (trough viscosity), SV, and FV (final viscosity) of modified starches decreased while pasting temperature (PT) was increased as compared to their native counterpart starches (). The cross-linking modification of starches has been reported to decrease PV, BV, SV and increase the PT of starches. [Citation10,Citation29,Citation31]
Table 2a. Pasting properties of cross-linked starches from different cultivars.
Table 2b. Pasting properties of native starches from different cultivarsb.
Figure 1. Pasting properties of cross-linked starches from different pearl millet cultivars.
Cultivars are denoted by: A: HC-10; B: HHB-67; C: HHB-223; D: HHB-226; E: W-445; F: GHB-732.
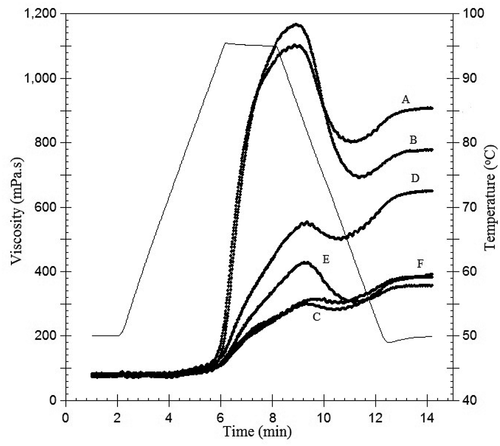
Dynamic shear properties
The rheological properties of cross-linked starches from different cultivars during heating are shown in . , ) illustrates changes in storage modulus (G′) and loss modulus (G′′), respectively, of modified starches during heating of suspensions. For all starches, G′ and G′′ increased progressively during heating. The values of G′ and G′′ ranged from 3613 to 6334 Pa and 352 to 441 Pa, respectively, for native pearl millet starches.[Citation26] G′ values of cross-linked starches ranged from 2603 to 4970 Pa, the highest and the lowest was observed for cv.W-445 and cv.HHB-223, respectively. Cross-linking modification significantly changes the rheological behaviour of starches. After modification, decrease in peak G′ was observed which may be due to the lower degree of swelling and thus less inter-granular interaction (curves for native starches not shown). Kaur et al.[Citation32] also reported that cross-linked potato starches show lower G′ value as compared to their native counterpart starch. The tan δ (ratio of G′′/G′) values varied from 0.06 to 0.10 (), which shows that all the samples were more elastic than viscous.
Table 3. Rheological properties of cross-linked starches from different cultivars during heating.
, , ) shows the change in the G′, G′′, and tan δ as a function of the frequency at 6.28 rad/s and 25°C for cross-linked starch pastes from different cultivars. G′ and G′′ increase with increase in frequency during the frequency sweep test. G′ and G″ are the measure of the energy stored and loss in a material or recoverable per cycle of deformation.[Citation33] G′ value was much higher than the G′′ valueat all frequency values for starch pastes from all the cultivars. G′ and G′′ values for native starches varied from 4749 to 6564 Pa and 163 to 228 Pa, respectively. The highest G′ and G′′ values were observed for starch pastes of cv.GHB-732 and cv.HHB-223, respectively while the lowest values were for cv.HC-10 (). Magnitude of G′ and G′′ for cross-linked starches varied from 3264 to 5076 Pa and 174 to 240 Pa, respectively. In contrary to native starches, the highest G′ and G′′ values for starch pastes were observed for cv.HC-10 and cv.GHB-732, respectively (). G′ and G′′ increased with increase in frequency showing frequency dependency. G′ and G′′ values of cross-linked starch pastes were observed lower than their native counterpart starch pastes. Kim and YooCitation[34] observed that frequency values decreases as the concentration of cross-linking reagent increases.
Table 4a. Rheological properties of native starches from different cultivars during frequency sweep.
Table 4b. Rheological properties of cross-linked starches from different cultivars during frequency sweep.
Steady shear properties
Plots of shear stress (σ) versus shear rate (γ̇) data for cross-linked starch pastes from different cultivars are shown in . The experimental data of flow behaviour for cross-linked starch pastes were fitted to Herschel-Bulkley model and yield stress (σo), flow behaviour index (n), and consistency index (K) were evaluated (). The steady shear properties were best described by the model with high determination coefficients (R2 = 0.991 to 0.999). σo, indicative of the minimum force required to initiate flow of starch paste of cross-linked starches varied from 0.27 to 3.2 Pa, the highest values for starch paste from cv.HC-10 and the lowest value was observed for starch paste from cv.HHB-67. K values of cross-linked starch pastes ranged from 0.16 to 0.77, paste from cv.GHB-732 had the highest value. σo and K values of pastes from cross-linked starches decreased as compared to the paste from native starches (values not shown). σo and K values for native starches varied from 25.6 to 183.4 Pa and 1.69 to 54.5 Pa.s, respectively, the highest value for cv.GHB-732 and w-445 were observed.[26] To characterize fluid and semi-fluid mostly n value is used, behaviour of pastes with n value of 1 indicates a Newtonian fluid, n value of less than 1 show a shear thinning, and n value of greater than 1 describing a shear thickening fluid behaviour.[Citation33] Kim and Yoo[Citation34] reported that decreased values of K and σo can be explained by the decreased swelling power and solubility of the cross-linked starch granules as compared to native starch granules due to lower water absorption of the starch granules caused by cross-linking reagent. Nunez-Santiago et al.[Citation35] stated that covalent bonds of cross-linking reactions reinforce starch granular structure and are less likely to break down under extended cooking, increased acidity, or agitation.
Table 5. Steady shear properties of cross-linked starches from different cultivars fitted with Herschel-Bulkley model.
Figure 2b. Changes in G” of cross-linked starches from different cultivars during heating.
Cultivars are denoted by: A: HC-10; B: HHB-67; C: HHB-223;D: HHB-226; E: W-445; F: GHB-732.
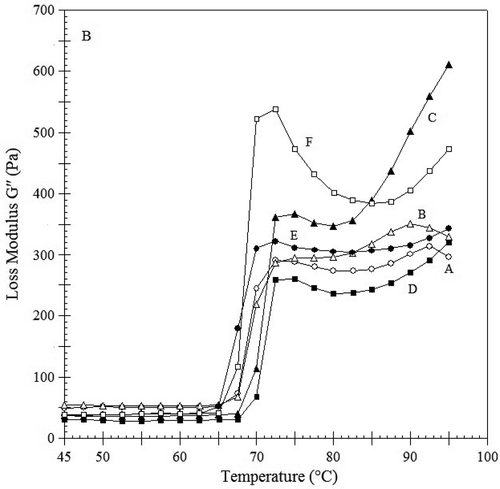
Morphological properties
Scanning electron micrographs (SEM) play an important role in the understanding of granular structure of native and modified starches. SEM is widely used to determine structural changes caused by chemical modifications.[Citation36] SEM of cross-linked pearl millet starches are shown in . SEM investigations revealed that the cross-linking caused slight changes in granular structure of starch as compared to native starch. Native starch granules were spherical, polygonal with well-defined edges, whereas for the cross-linked starch granules, slightly rough surface and the formation of grooves were observed that indicates slight fragmentation. These results were consistent with previous studies reported by Carmona-Garcia et al.[Citation37] and Koo et al.[Citation8] Van Hung and Morita[Citation2] reported that large granules surface is prone to greater damage after modification as compared to small granules.
In vitro digestibility properties
The RDS, SDS, and RS contents of cross-linked starches from different cultivars are shown in . RDS of cross-linked starches varied from 46.1% to 50.6%, the highest and the lowest values were observed for cv.W-445 and cv.GHB-732, respectively. SDS and RS content of starches ranged from 34.5% to 36.4% and 13.6% to 19.4%, cv.HC-10 and cv.GHB-732 had the highest values. RDS, SDS, and RS content of native pearl millet starches ranged from 46.3% to 51.6%, 37.2% to 38.7%, and 9.7% to 16.5%, respectively.[Citation26] Cross-linked modification decreased SDS and RDS content whereas RS content was increased as compared to their native counterpart starches (values not shown). RS has potential physiological benefits similar to dietary fibre and unique functional properties.[Citation38] Chung et al.[Citation39] reported that cross-linking modification using mixture of STMP and STPP, decreased SDS and RDS contents while increased the RS content for corn starch. Woo and Seib[Citation40] observed that RS increased after cross-linking modification for wheat, potato, corn, and rice starches. Shukri and Shi[Citation41] stated that high level of cross-linking reduces digestibility probably by strongly inhibiting the swelling of starch granules, which restricts access of amylase to starch molecules. Various health benefits of RS have been reported as prevention of colon cancer, hypoglycemic effects, substrate for the growth of the probiotics, and increase in absorption of minerals.[Citation42]
Table 6. In vitro digestibility of cross-linked starch from different cultivars.
Conclusion
Starch modified with epichlorohydrin (0.5%) caused significant changes in physicochemical, pasting, rheological, and in vitro digestibility properties. In comparison to native counterpart, decrease in values of amylose content, swelling power, and solubility of cross-linked starches was observed. Cross-linked starch from cv.GHB-732 showed the highest degree of cross-linking and the lowest value for amylose content, swelling power, and solubility. G′ of starches from different cultivars decreased when heated with cv.GHB-732 having the lowest value. Steady shear study using Herschel-Bulkley model revealed shear thinning behaviour of starch pastes from cross-linked starches. SEM analysis revealed slightly rough surface of granules with slight fragmentations. In vitro starch digestibility studies showed that cross-linked starch from different cultivars had high RS content.
Additional information
Funding
References
- Saartrat, S.; Puttanlek, C.; Rungsardthong, V.; Uttapap, D. Paste and Gel Properties of Low-Substituted Acetylated Canna Starches. Carbohydrate Polymers 2005, 61, 211–221. DOI: 10.1016/j.carbpol.2005.05.024.
- Van Hung, P.; Morita, N. Effects of Granule Sizes on Physicochemical Properties of Cross‐Linked and Acetylated Wheat Starches. Starch/Stärke 2005, 57, 413–420. DOI: 10.1002/star.200500417.
- Sandhu, K. S.; Sharma, L.; Kaur, M. Effect of Granule Size on Physicochemical, Morphological, Thermal and Pasting Properties of Native and 2-Octenyl-1-Ylsuccinylated Potato Starch Prepared by Dry Heating under Different pH Conditions. LWT Food Science and Technology 2015, 61, 224–230. DOI: 10.1016/j.lwt.2014.11.004.
- Ratnayake, W. S.; Jackson, D. S. Phase Transition of Cross-Linked and Hydroxypropylated Corn (Zea Mays L.) Starches. LWT Food Science and Technology 2008, 41, 346–358. DOI: 10.1016/j.lwt.2007.03.008.
- Jyothi, A. N.; Moorthy, S. N.; Rajasekharan, K. N. Effect of Cross‐Linking with Epichlorohydrin on the Properties of Cassava (Manihot Esculenta Crantz) Starch. Starch/Stärke 2006, 58, 292–299. DOI: 10.1002/star.200500468.
- Chung, H. J.; Woo, K. S.; Lim, S. T. Glass Transition and Enthalpy Relaxation of Cross-Linked Corn Starches. Carbohydrate Polymers 2004, 55, 9–15. DOI: 10.1016/j.carbpol.2003.04.002.
- Suma, P. F.; Urooj, A. Isolation and Characterization of Starch from Pearl Millet (Pennisetum typhoidium) Flours. International Journal of Food Properties 2015, 18, 2675–2687. DOI: 10.1080/10942912.2014.981640.
- Koo, S. H.; Lee, K. Y.; Lee, H. G. Effect of Cross-Linking on the Physicochemical and Physiological Properties of Corn Starch. Food Hydrocoll 2010, 24, 619–625. DOI: 10.1016/j.foodhyd.2010.02.009.
- Kahraman, K.; Koksel, H.; Ng, P. K. Optimisation of the Reaction Conditions for the Production of Cross-Linked Starch with High Resistant Starch Content. Food Chemistry 2015, 174, 173–179. DOI: 10.1016/j.foodchem.2014.11.032.
- Ackar, D.; Babic, J.; Subaric, D.; Kopjar, M.; Milicevic, B. Isolation of Starch from Two Wheat Varieties and Their Modification with Epichlorohydrin. Carbohydrate Polymers 2010, 81, 76–82. DOI: 10.1016/j.carbpol.2010.01.058.
- Yoneya, T.; Ishibashi, K.; Hironaka, K.; Yamamoto, K. Influence of Cross-Linked Potato Starch Treated with POCl3 on DSC, Rheological Properties and Granule Size. Carbohydrate Polymers 2003, 53, 447–457. DOI: 10.1016/S0144-8617(03)00143-7.
- Zhao, J.; Schols, H. A.; Chen, Z.; Jin, Z.; Buwalda, P.; Gruppen, H. Substituent Distribution within Cross-Linked and Hydroxypropylated Sweet Potato Starch and Potato Starch. Food Chemistry 2012, 133, 1333–1340. DOI: 10.1016/j.foodchem.2012.02.021.
- Mirmoghtadaie, L.; Kadivar, M.; Shahedi, M. Effects of Cross-Linking and Acetylation on Oat Starch Properties. Food Chemistry 2009, 116, 709–713. DOI: 10.1016/j.foodchem.2009.03.019.
- Shin, M.; Song, J.; Seib, P. A. In Vitro Digestibility of Cross‐Linked starches–RS4. Starch/Stärke 2004, 56, 478–483. DOI: 10.1002/star.200400301.
- Sandhu, K. S.; Singh, N. Relationships between Selected Properties of Starches from Different Corn Lines. International Journal of Food Properties 2005, 8, 481–491. DOI: 10.1080/10942910500267711.
- Wurtzburg, O. B. 1960. Preparation of Starch Derivatives. US Patent No. 2,935,510.
- Chatakanonda, P.; Varavinit, S.; Chinachoti, P. Relationship of Gelatinization and Recrystallization of Cross-Linked Rice to Glass Transition Temperature. Cereal Chemistry 2000, 77, 315–319. DOI: 10.1094/CCHEM.2000.77.3.315.
- Williams, P. C.; Kuzina, F. D.; Hlynka, L. A. Rapid Calorimetric Procedure for Estimating the Amylose Content of Starches and Flour. Cereal Chemistry 1970, 47, 411–421.
- Leach, H. W.; McCowen, L. D.; Schoch, T. J. Structure of the Starch Granule I. Swelling and Solubility Patterns of Various Starches. Cereal Chemistry 1959, 36, 534–544.
- Thory, R.; Sandhu, K. S. A. Comparison of Mango Kernel Starch with a Novel Starch from Litchi (Litchi chinensis) Kernel: Physicochemical, Morphological, Pasting, and Rheological Properties. International Journal of Food Properties 2016, 20, 911–921. DOI: 10.1080/10942912.2016.1188403.
- Kaur, M.; Singh, S. Physicochemical, Morphological, Pasting and Rheological Properties of Tamarind (Tamarindus indica L.) Kernel Starch. International. International Journal of Food Properties 2016, 19, 2432–2442. DOI: 10.1080/10942912.2015.1121495.
- Park, S.; Chung, M. G.; Yoo, B. Effect of Octenylsuccinylation on Rheological Properties of Corn Starch Pastes. Starch/Stärke 2004, 56, 399–406. DOI: 10.1002/star.200300274.
- Englyst, H. N.; Kingman, S. M.; Cummings, J. H. Classification and Measurement of Nutritionally Important Starch Fractions. European Journal of Clinical Nutrition 1992, 46, S33–S50.
- Chung, H. J.; Lim, H. S.; Lim, S. T. Effect of Partial Gelatinization and Retrogradation on the Enzymatic Digestion of Waxy Rice Starch. Journal of Cereal Science 2006, 43, 353–359. DOI: 10.1016/j.jcs.2005.12.001.
- Xiao, H.; Lin, Q.; Liu, G. Q.; Wu, Y.; Tian, W.; Wu, W.; Fu, X. Physicochemical Properties of Chemically Modified Starches from Different Botanical Origin. Science Research Essays. 2011, 6, 4517–4525. DOI: 10.5897/SRE11.618.
- Sandhu, K. S.; Siroha, A. K. Relationships between Physicochemical, Thermal, Rheological and in Vitro Digestibility Properties of Starches from Pearl Millet Cultivars. LWT Food Science and Technology 2017, 83, 213–224.
- Sukhija, S.; Singh, S.; Riar, C. S. Physicochemical, Crystalline, Morphological, Pasting and Thermal Properties of Modified Lotus Rhizome (Nelumbo nucifera) Starch. Food Hydrocoll 2016, 60, 50–58. DOI: 10.1016/j.foodhyd.2016.03.013.
- Singh, A. V.; Nath, L. K. Synthesis and Evaluation of Physicochemical Properties of Cross-Linked Sago Starch. International Journal of Biological Macromolecules 2012, 50, 14–18. DOI: 10.1016/j.ijbiomac.2011.09.003.
- Kurakake, M.; Akiyama, Y.; Hagiwara, H.; Komak, T. Effects of Cross-Linking and Low Molecular Amylose on Pasting Characteristics of Waxy Corn Starch. Food Chemistry 2009, 116, 66–70. DOI: 10.1016/j.foodchem.2009.02.006.
- Jan, R.; Saxena, D. C.; Singh, S. Pasting, Thermal,Morphological, Rheological and Structural Characteristics of Chenopodium (Chenopodium album) Starch. LWT Food Science and Technology 2016, 66, 267–274. DOI: 10.1016/j.lwt.2015.10.040.
- Rodriguez‐Marín, M. L.; Nunez‐Santiago, C.; Wang, Y. J.; Bello‐Perez, L. A. Physicochemical and Structural Characteristics of Cross‐Linked Banana Starch Using Three Cross‐Linking Reagents. Starch/Stärke 2010, 62, 530–537. DOI: 10.1002/star.201000025.
- Kaur, L.; Singh, J.; Singh, N. Effect of Cross-Linking on Some Properties of Potato (Solanum tuberosum L.) Starches. Journal of the Science of Food and Agriculture 2006, 86, 1945–1954. DOI: 10.1002/(ISSN)1097-0010.
- Lee, Y.; Chang, Y. H. Effects of Galactomannan Addition on Rheological, Pasting and Physical Properties of Water Chestnut Starch. Journal of Texture Studies 2015, 46, 58–66. DOI: 10.1111/jtxs.2015.46.issue-1.
- Kim, B. Y.; Yoo, B. Effects of Cross‐Linking on the Rheological and Thermal Properties of Sweet Potato Starch. Starch/Stärke. 2010, 62, 577–583. DOI: 10.1002/star.201000035.
- Nunez-Santiago, M. C.; Bello-Perez, L. A.; Tecante, A. Swelling-Solubility Characteristics, Granule Size Distribution and Rheological Behavior of Banana (Musa paradisiaca) Starch. Carbohydrate Polymers 2004, 56, 65–75. DOI: 10.1016/j.carbpol.2003.12.003.
- Kim, H. R.; Hermansson, A. M.; Eriksson, C. E. Structural Characteristics of Hydroxypropyl Potato Starch Granules Depending on Their Molar Substitution. Starch/Stärke 1992, 44, 111–116. DOI: 10.1002/star.19920440307.
- Carmona-Garcia, R.; Sanchez-Rivera, M. M.; Méndez-Montealvo, G.; Garza-Montoya, B.; Bello-Pérez, L. A. Effect of the Cross-Linked Reagent Type on Some Morphological, Physicochemical and Functional Characteristics of Banana Starch (Musa paradisiaca). Carbohydrate Polymers 2009, 76, 117–122. DOI: 10.1016/j.carbpol.2008.09.029.
- Kaur, M.; Sandhu, K. S.; Singh, N.; Lim, S. T. Amylose Content, Molecular Structure, Physicochemical Properties and in Vitro Digestibility of Starches from Different Mung Bean (Vignaradiata L.) Cultivars. Starch/Stärke 2011, 63, 709–716. DOI: 10.1002/star.201100053.
- Chung, H. J.; Shin, D. H.; Lim, S. T. In Vitro Starch Digestibility and Estimated Glycemic Index of Chemically Modified Corn Starches. Food Res. Int. 2008, 41, 579–585. DOI: 10.1016/j.foodres.2008.04.006.
- Woo, K. S.; Seib, P. A. Cross-Linked Resistant Starch: Preparation and Properties 1. Cereal Chemistry 2002, 79, 819–825. DOI: 10.1094/CCHEM.2002.79.6.819.
- Shukri, R.; Shi, Y. C. Physiochemical Properties of Highly Cross-Linked Maize Starches and Their Enzymatic Digestibilities by Three Analytical Methods. Journal of Cereal Science 2015, 63, 72–80. DOI: 10.1016/j.jcs.2015.03.001.
- Sajilata, M. G.; Singhal, R. S.; Kulkarni, P. R. Resistant Starch–A Review. Comprehensive Reviews in Food Science and Food Safety 2006, 5, 1–17. DOI: 10.1111/j.1541-4337.2006.tb00076.x.