ABSTRACT
The well-organized chitosan film on mica surface has drawn wide attention for its potential applications and studies on the process of self-assembly. In this article, atomic force microscopy was used to investigate the ultrastructure of the self-assembly of chitosan molecules with calcium ions in different concentrations. It was showed that calcium ions might affect the self-assembly of chitosan to a certain extent, and chitosan molecules gathered from fibrils to circular, and finally formed to film with the increasing concentration of calcium ions. Further, the formation mechanism of Ca(II)-chitosan conjugates was adapted to explain the effects of calcium ions on the self-assembly of chitosan. These results and the related analysis would be valuable for guiding the pattern of chitosan self-assembly with a potential application in food packaging science and engineering.
Introduction
In recent years, as people pay more attention to food safety, the requirements of non-toxic and biodegradable material are becoming increasingly urgent. Chitosan is a unique alkaline polysaccharide in the nature obtained by partial or total removal of acetyl groups from chitin. Chitosan is not only abundant and biodegradable but also a renewable resource. The chitosan is easy to form film which has a certain permeability of O2, CO2, and water vapour. It has many valuable properties such as biocompatibility, antibacterial property, biodegradability, low toxicity, and metal binding ability.[Citation1–Citation5] Moreover, antibacterial property makes the film has a broad prospective. Over the past two decades, the performance of chitosan film has been reported extensively. [Citation6,Citation7] Although chitosan film in food preservation has a great advantage, the film has high water vapor permeability, poor mechanical strength, narrow antibacterial range and other shortcomings, which limit its application scope. Since the chitosan molecule contains many amino groups and hydroxyl groups in each unit, it could be complexed with metal ions and has strong intramolecular hydrogen bond.[Citation8–Citation10] Therefore, metal ions act as a cross-linking agent, making the similar reticular structures formed by hydrogen bonds or salt bonds between chitosan molecules more compact.
Due to the good coordination of chitosan with metal ions, it presents many special physical and chemical properties as well as biological activity, so it has been widely applied in chemical industry, pharmaceutical industry, agriculture, bioengineering, food industry, and so forth.[Citation11] There were many studies on the adsorption of chitosan and Fe2+, Cd2+, Pb2+, Zn2+, Cu2+, Ag+ conjugates.[Citation12–Citation16] Unfortunately, very little attention has been paid to the complexation between chitosan and Ca2+. The related results showed that, chitosan mainly reacts with a calcium ion through its four amino,[Citation17] and the schematic diagram of complexation mechanism was shown in . While many studies have shown that, Ca(II)-chitosan conjugates film have a certain preservation effect on mango, fresh lettuce, pumpkin, carrot, strawberry, and so on.[Citation18–Citation22] Moreover, calcium is a better choice to apply to the food preservation as a constant element of human body. Therefore, Ca(II)-chitosan conjugates film is worthy of study.
Previous studies have shown that, chitosan aggregates in star-like structure, while those which absorb Ag+ grow disc-like structure and the Ag(I), Cu(II)-chitosan conjugates fractal dimension is smaller.[Citation23] It could be speculated that adding metal ions can regulate the morphology of the film. At the same time, the atomic force microscopy (AFM) that we chose to study self-assembly was an efficient and widely used technique in molecular and cellular biology for its nano-scale resolution and simple sample preparation methods. Many AFM studies indicated that it could acquire lots of information on the self-assembly of chitosan on mica surface.[Citation24–Citation30] Thus, atomic force microscopy (AFM) was used to investigate chitosan and Ca(II) conjugates with different concentrations in this work.
Materials and methods
Preparation of chitosan solution
Totally, 5 mg/mL chitosan was obtained by dissolving chitosan with the deacetylation degree of 80.0%~ 95.0% (Guiyang Chemical Co., Guizhou, China) using 1% acetic acid solution (Purity≥ 99.5%, Guanghua Chemical Factory Co., Guangdong, China), and then to configure the concentration of 5 mg/mL chitosan, and then diluted to 100 μg/mL, 200 μg/mL, 500 μg/mL, 1 mg/mL, 2 mg/mL, and 3 mg/mL separately. After standing for a while, 10 μL of the solutions were added onto the freshly cleaved mica sheets (Tosai, Hong Kong, China) in 1.0 × 0.5 cm2, finally were placed, covered and dried in 12-wells plates at room temperature.
100 mMCaAc2(Chengdu Kelong Chemical Reagent Factory Chengdu, China) was prepared with1% acetic acid solution, and it was diluted to prepare CaAc2 solution that was twice the concentration of the desired solution at concentrations of 0.2 mM, 0.4 mM, 1 mM, 2 mM, 4 mM, 10 mM, 20 mM, 40 mM, 100 mM. Then 100 μg/mL chitosan and CaAc2 solution were mixed up in equal volume to get Ca(II)-chitosan conjugates, then was placed, covered and dried in 12-wells plates at room temperature.
AFM imaging of the self-assembly of chitosan
A Multimode-8 type AFM (Bruker Co., Santa Barbara, USA) was used in ScanAsyst mode with ScanAsyst-AIR probes to image the naturally dried chitosan at 0.977 Hz.AFM was used for visualization of chitosan and Ca(II)-chitosan conjugates deposited on mica sheet. First placed the sample on the stage, adjusted the light mirror to find a clean location under the light microscope, then placed the needle holder and fixed it so that the needle was just touching the sample. First selected the 500 nm scan range, align the X-axis and Y-axis to the origin position. Finally chose typical region to gain images when the range was extended from 500 nm, 1 µm, 2 µm, 5 µm, to 10 µm. All images are typically obtained from at least five macroscopically separated regions on each sample at room temperature with the related humidity of 55%.
Data analysis
Typical height images, roughness, and surface area difference were statistical analyzed using the offline software Nanoscope Analysis V1.5 (Bruker Co., Santa Barbara, USA) after using 1st order flatten and noise-line eraser to smooth the image. Then, the “section” function could output the section profiles. And root mean square roughness (Rq) and average roughness (Ra) were collected by the “roughness” function to describe the topological change, and the surface area difference was gathered to indicate the degree of surface coverage. All data were expressed as mean ± standard deviations. Analyses were performed using Excel 2010 (Microsoft Co., Redmond, USA) and SPSS22.0 (IBM Co., Armonk, USA).
Results and discussion
Self-assembly of chitosan in different chitosan concentration
Firstly, the effect of chitosan concentration on the structure of the assembly on mica surface was investigated by AFM and the typical height images and section profiles curves were collected and shown in . The values of height, roughness (Ra, Rq) and surface area difference were shown in . When the concentration of chitosan is low, chitosan was uniformly dispersed on the mica sheet (-c). At 0.01 mg/mL, the height was 1.07 ± 0.21 nm, Ra was 0.153 nm, Rq was 0.251 nm, surface area difference was 0.114%. While the height of 0.01 mg/mL chitosan was increased to 4 ± 2, and Ra was 0.214 nm, Rq was 0.430 nm, surface area difference was 0.115%. With the concentration increased slowly to 0.05 mg/mL (), the height was decreased to 0.90 ± 0.26 nm, Ra was 0.271 nm, Rq was 0.343 nm, surface area difference was 0.111%, the chitosan particles became more intensive and began to form a little short, thick fibers.
As shown in -f, when the concentration of chitosan increased from 0.1 mM to 0.5 mM (-f), the height changed from 8.59 ± 0.11 nm, 11.43 ± 0.26 nm, to 8.11 ± 0.19 nm in turn, Ra was 2.02 nm, 1.94 nm, and 2.24 nm, successively, Rq was 2.52 nm, 2.47 nm and 2.73 nm in turn, and surface area difference varied from1.07%, 1.41%, to 1.70% one by one. Chitosan particles were evenly and densely distributed on the surface of mica at 0.1 mg/mL, as shown in . With the increase of concentration, partial chitosan fibers firstly gathered due to the interaction among chitosan molecules. At 0.2 mg/mL (see ), the average vertical height of chitosan particles increased to 11.43 ± 0.26 nm, compared with the data of chitosan at 0.1 mg/mL. However, the Ra and Rq did not change significantly. While the chitosan concentration further increased, chitosan fibrils were largely formed and began to overspread in the whole picture for the enhanced interaction among chitosan molecules. Thus, the height of 0.5 mg/mL chitosan () was reduced to 8.11 ± 0.19 nm, but Ra, Rq and surface area difference were increased simultaneously. Chitosan fibrils became dense obviously and distributed evenly at 1 mg/mL ().The height of the 1 mg/mL chitosan was reduced more than a half to 3.31 ± 0.10 nm, Ra was 1.09 nm, Rq was 1.33 nm, and the surface area difference was considerably reduced to 0.593%.When the chitosan concentration increased to2 mg/mL (), rough chitosan film was formed with the height of 12.20 ± 0.75 nm, Ra and Rq increased to 3.43 nm, and 4.35 nm individually, and the surface area difference was 1.91%.After the concentration of chitosan increased to3 mg/mL (), the chitosan film got thicker with the chitosan particles height of 16.84 ± 0.61 nm, Ra of 3.40 nm, Rq of 4.24 nm, and surface area difference of 1.14% separately. Although the height increased, the Ra and Rq changed little compared to the data of chitosan at 2 mg/mL.
It was found that chitosan was gathered from short and thick fibers () to long and fine fibrous structure (), and the number of chitosan fibrils obviously increased with the increase of chitosan concentration (-g). The intermolecular and intramolecular hydrogen bond played a key role in chain gathering. Finally, rough and dense chitosan film was formed (-i).
Table 1. Height, roughness (Ra, Rq) and surface area difference of the self-assembly of chitosan molecules in different chitosan concentrations.
Self-assembly of ca(ii)-chitosan conjugates in different caac2 concentration
Because 50 ug/mL chitosan fibers were evenly distributed, and its shape was clear, to farther gain the clear pattern and mechanism of interaction between calcium ions and chitosan molecules in details, the chitosan concentration was reduced to 50 μg/mL in the further study. To investigate the effect of calcium ions concentration on the self-assembly of chitosan molecules, calcium acetate was added to the anion interference. Chitosan molecules assembled on mica surfaces with increasing concentration of calcium ions and the AFM height images were shown in .The Values of height, roughness (Ra, Rq) and surface area difference derived from the AFM height images were shown in . The line chart of height, Ra, Rq change with concentration were shown in .
Table 2. Height, roughness (Ra, Rq) and surface area difference of the self-assembly of 50 μg/mL chitosan molecules in different CaAc2 concentrations.
Figure 3. Typical AFM height images of 50 μg/mL chitosan assembled on mica surface in different concentration of CaAc2. (a) 0.1 mM, (b) 0.2 mM, (c) 0.5 mM, (d) 1 mM, (e) 2 mM, (f) 5 mM, (g) 10 mM, (h) 20 mM, and (i) 50 mM.
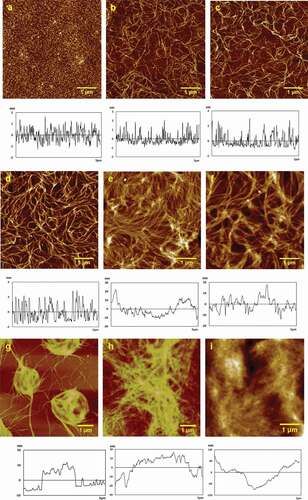
It can be seen from the AFM height images that the morphology of self-assembled Ca(II)-chitosan conjugates was largely different in the presence of different CaAc2 concentrations. In the presence of 0.1 mM CaAc2, particles gathered closely with height of 2 ± 1 nm, and Ra of 0.549 nm, Rq of 0.432 nm, and surface area difference of 0.130%, as shown in . With the increase in calcium ions concentration, chitosan particles gathered into fibers and mutually crosslinked on the mica sheet. In the presence of 0.2 mM CaAc2, fibril-like structure of assembled chitosan was observed in . At 0.5 mM CaAc2, chitosan fibrils were much thicker than that in 0.2 mM CaAc2, as shown in . While the CaAc2 concentration further increased to 1 mM (), the fibers became more rough and denser. Then the numbers of chitosan fibers were significantly increased, and fibers became largely thick. At 2 mM CaAc2 (), the fibers distributed very close, and the height tended to be evenly. While at 5 mM CaAc2 (), the fibers start to further crosslink based on the multilayered fibers furthermore. The concentration of calcium ions increased from 0.2 mM to 5 mM (-f) and the height changed from1.95 ± 0.98 nm, 3 ± 2 nm, 3.80 ± 0.92 nm, 5 ± 1 nm, to 7 ± 3 nm in turn, the Ra values grew from 0.478 nm, 0.721 nm, 0.993 nm, 2.84 nm to 4.79 nm, the Rq values increased form0.606 nm, 0.898 nm, 1.13 nm, 3.76 nm to 5.93 nm,and the surface area difference in turn was 0.172%, 0.312%, 0.228%, 1.32%, and 1.40%. And the data shows an increase in regularity. At 10 mM CaAc2, fibers formed into the ring-like structure due to fibers continuously crosslinked and gathered with the height of 10 ± 2 nm, and Ra of 3.01 nm, Rq of 3.81 nm, and surface area difference was reduced to 0.427%, as shown in .The white part of the figure showed where fibers tended to gather. And the size of the ring depends on how many crosslinked fibers gathered locally. It was shown that in , the fibers gathered and resulted in a long-range orderly effect. The main agent of the formation of structure originated from the surface tension and anisotropy. The ring-like structure of chitosan assembly was formed into film at 20 mM CaAc2with the height of 20 ± 6 nm, Ra of 3.20 nm, Rq of4.08 nm, and the surface area difference of 0.915%. Finally, at 50 mM CaAc2, the film’s height increased by three times to 62 ± 5 nm, Ra increased to 4.27 nm, Rq increased to 3.98 nm, and surface area differencealso increased to 0.482% simultaneously. It was obvious that, the Ra and Rq did not change much ().
With the increase inCaAc2 concentration, due to hydrogen bonding and intermolecular forces, chitosan firstly assembled on mica surfaces from the point to the fibrils (-b), and then chitosan fibrils grew to more intensive fibers (-d). Then the fibers gathered further thicker (-f), then gathered into circular (), and finally the ring-like structure of chitosan assembly gathered into the film (-i). The height was close to linear growth, the Ra, Rq, and surface area difference increased slowly with it. The fitting function was showed in . It was shown that calcium ions will affect the self-assembly of chitosan to a certain extent, and the results indicated that Ca(II)-chitosan conjugates are easier to form fibrils and film.
Conclusion
In summary, it was found that the self-assembly of chitosan and Ca(II)-chitosan conjugates is a dynamic process. During this process, calcium ions improve chitosan ability to enhanced interaction. Compared to single chitosan self-assembly, chitosan assembled on mica surface in the presence of CaAc2 easily tends to crosslink and gather into film. With the increase inCaAc2 concentration, chitosan firstly assembled on mica surfaces from the particles to the fibrils, then chitosan fibrils became more intensive fibers. Then the fibers gathered further thicker, then gathered into circular, and finally gathered into the film. The film derived from 50 μg/mL chitosan in the presence of 20 mM CaAc2 become obvious thick and smooth. When the concentration of calcium ion was increased to 50 μg/mL, the Ca(II)-chitosan film can be very thick. In contrast, single component chitosan film is not smooth enough. Based on the theoretical basis of chitosan complexation with calcium ion, the change of chitosan self-assembly in the presence of different calcium ion concentration can be controlled according to the demand. It may provide theoretical guidance and data supports for the research of the structural stability of Ca(II)-chitosan conjugates and its physiological and nutritional effects such as calcium supplementation.
Declaration of interest
The authors declare that they have no conflicts of interest.
Additional information
Funding
References
- Kumar, M.; Muzzarelli, R.; Muzzarelli, C.; Sashiwa, H.; Domb, A. J. Chitosan Chemistry and Pharmaceutical Perspectives. Chemical Reviews 2004, 104, 6017–6084.
- Langer, R.; Tirrell, D. A. Designing Materials for Biology and Medicine. Nature 2004, 428, 487–492. DOI: 10.1038/nature02388.
- Prashanth, K. V. H.; Tharanathan, R. N. Chitin/Chitosan: Modifications and Their Unlimited Application Potential - an Overview. Trends in Food Science & Technology 2007, 18, 117–131. DOI: 10.1016/j.tifs.2006.10.022.
- Huang, G.; Yin, Y.; Pan, Z.; Chen, M.; Zhang, L.; Liu, Y.; Zhang, Y.; Gao, J. Fabrication of 3D Photonic Crystals from Chitosan that are Responsive to Organic Solvents. Biomacromolecules 2014, 15, 4396–4402. DOI: 10.1021/bm501374t.
- Haque, T.; Chen, H.; Ouyang, W.; Martoni, C.; Lawuyi, B.; Urbanska, A. M.; Prakash, S. Investigation of a New Microcapsule Membrane Combining Alginate, Chitosan, Polyethylene Glycol and poly-L-lysine for Cell Transplantation Applications. International Journal of Artificial Organs 2015, 38, 291.
- Gong, J.; Hu, X.; Wong, K.; Zheng, Z.; Yang, L.; Lau, W.; Du, R. Chitosan Nanostructures with Controllable Morphology Produced by a Nonaqueous Electrochemical Approach. Advanced Materials 2008, 20, 2111. DOI: 10.1002/adma.200701840.
- Caridade, S. G.; Monge, C.; Gilde, F.; Boudou, T.; Mano, J. F.; Picart, C. Free-Standing Polyelectrolyte Membranes Made of Chitosan and Alginate. Biomacromolecules 2013, 14, 1653–1660. DOI: 10.1021/bm400314s.
- Fang, N.; Chan, V. Chitosan-Induced Restructuration of a Mica-Supported Phospholipid Bilayer: An Atomic Force Microscopy Study. Biomacromolecules 2003, 4, 1596–1604. DOI: 10.1021/bm034259w.
- Lee, D. W.; Lim, C.; Israelachvili, J. N.; Hwang, D. S. Strong Adhesion and Cohesion of Chitosan in Aqueous Solutions. Langmuir 2013, 29, 14222–14229. DOI: 10.1021/la403124u.
- Varma, A. J.; Deshpande, S. V.; Kennedy, J. F. Metal Complexation by Chitosan and Its Derivatives: A Review. Carbohydrate Polymers 2004, 55, 77–93. DOI: 10.1016/j.carbpol.2003.08.005.
- Rinaudo, M.;. Chitin and Chitosan: Properties and Applications. Progress in Polymer Science 2006, 31, 603–632. DOI: 10.1016/j.progpolymsci.2006.06.001.
- Ngah, W.; Ab Ghani, S.; Kamari, A. Adsorption Behaviour of Fe(II) and Fe(III) Ions in Aqueous Solution on Chitosan and Cross-Linked Chitosan Beads. Bioresource Technology 2005, 96, 443–450. DOI: 10.1016/j.biortech.2004.05.022.
- Guibal, E.;. Interactions of Metal Ions with Chitosan-Based Sorbents: A Review. Separation and Purification Technology 2004, 38, 43–74. DOI: 10.1016/j.seppur.2003.10.004.
- Webster, A.; Halling, M. D.; Grant, D. M. Metal Complexation of Chitosan and Its Glutaraldehyde Cross-Linked Derivative. Carbohydrate Research 2007, 342, 1189–1201. DOI: 10.1016/j.carres.2007.03.008.
- Qi, L. F.; Xu, Z. R.; Jiang, X.; Hu, C. H.; Zou, X. F. Preparation and Antibacterial Activity of Chitosan Nanoparticles. Carbohydrate Research 2004, 339, 2693–2700. DOI: 10.1016/j.carres.2004.09.007.
- Potara, M.; Jakab, E.; Damert, A.; Popescu, O.; Canpean, V.; Astilean, S. Synergistic Antibacterial Activity of Chitosan-Silver Nanocomposites on Staphylococcus Aureus. Nanotechnology 2011, 22(13), 135101. DOI: 10.1088/0957-4484/22/13/135101.
- Rhazi, M.; Desbrieres, J.; Tolaimate, A.; Rinaudo, M.; Vottero, P.; Alagui, A.; El Meray, M. Influence of the Nature of the Metal Ions on the Complexation with Chitosan. Application to the Treatment of Liquid Waste. European Polymer Journal 2002, 38, 1523–1530. DOI: 10.1016/S0014-3057(02)00026-5.
- Hernandez-Munoz, P.; Almenar, E.; Ocio, M. J.; Gavara, R. Effect of Calcium Dips and Chitosan Coatings on Postharvest Life of Strawberries (Fragaria X Ananassa). Postharvest Biology and Technology 2006, 39, 247–253. DOI: 10.1016/j.postharvbio.2005.11.006.
- Chong, J. X.; Lai, S.; Yang, H. Chitosan Combined with Calcium Chloride Impacts Fresh-Cut Honeydew Melon by Stabilising Nanostructures of Sodium-Carbonate-Soluble Pectin. Food Control 2015, 53, 195–205. DOI: 10.1016/j.foodcont.2014.12.035.
- Hernandez-Munoz, P.; Almenar, E.; Del Valle, V.; Velez, D.; Gavara, R. Effect of Chitosan Coating Combined with Postharvest Calcium Treatment on Strawberry (Fragaria X Ananassa) Quality during Refrigerated Storage. Food Chemistry 2008, 110, 428–435. DOI: 10.1016/j.foodchem.2008.02.020.
- Ayon-Reyna, L. E.; Tamayo-Limon, R.; Cardenas-Torres, F.; Lopez-Lopez, M. E.; Lopez-Angulo, G.; Lopez-Moreno, H. S.; Lopez-Cervantes, J.; Lopez-Valenzuela, J. A.; Vega-Garcia, M. O. Effectiveness of Hydrothermal-Calcium Chloride Treatment and Chitosan on Quality Retention and Microbial Growth during Storage of Fresh-Cut Papaya. Journal of Food Science 2015, 80, C594–C601. DOI: 10.1111/1750-3841.12783.
- Suwannarak, J.; Phanumong, P.; Rattanapanone, N. Combined Effect of Calcium Salt Treatments and Chitosan Coating on Quality and Shelf Life of Carved Fruits and Vegetables. Chiang Mai University Journal of Natural Sciences 2015, 14, 269–284. DOI: 10.12982/CMUJNS.
- Hu, Y.; Wu, Y.; Cai, J.; Ma, Y.; Wang, B.; Xia, K.; He, X. Self-Assembly and Fractal Feature of Chitosan and Its Conjugate with Metal Ions: Cu(II)/Ag(I). International Journal of Molecular Sciences 2007, 8, 1–12. DOI: 10.3390/i8010001.
- Song, Y.; Li, Z.; Liu, Z.; Wei, G.; Wang, L.; Sun, L.; Guo, C.; Sun, Y.; Yang, T. A Novel Strategy to Construct A Flat-Lying DNA Monolayer on A Mica Surface. Journal of Physical Chemistry B 2006, 110, 10792–10798. DOI: 10.1021/jp0564344.
- Wang, L.; Jiang, J. G.; Song, Y. H.; Zhang, B. L.; Wang, E. K. Self-Assembled Monolayer Growth of Octanol on Mica: Atomic Force Microscopy and Fourier-Transform Infrared Spectroscopy Studies. Langmuir 2003, 19, 4953–4957. DOI: 10.1021/la026458r.
- Tang, J.; Ebner, A.; Ilk, N.; Lichtblau, H.; Huber, C.; Zhu, R.; Pum, D.; Leitner, M.; Pastushenko, V.; Gruber, H. J.; et al. High-Affinity Tags Fused to S-Layer Proteins Probed by Atomic Force Microscopy. Langmuir 2008, 24, 1324–1329. DOI: 10.1021/la702276k.
- Preiner, J.; Tang, J.; Pastushenko, V.; Hinterdorfer, P. Higher Harmonic Atomic Force Microscopy: Imaging of Biological Membranes in Liquid. Physical Review Letter 2007, 99(4), 046102. DOI: 10.1103/PhysRevLett.99.046102.
- Wang, H.; Hao, X.; Shan, Y.; Jiang, J.; Cai, M.; Shang, X. Preparation of Cell Membranes for High Resolution Imaging by AFM. Ultramicroscopy 2010, 110, 305–312. DOI: 10.1016/j.ultramic.2009.12.014.
- Wang, L.; Wu, J.; Guo, Y.; Gong, C.; Song, Y. Topographic Characterization of the Self-Assembled Nanostructures of Chitosan on Mica Surface by Atomic Force Microscopy. Applied Surface Science 2015, 353, 757–763. DOI: 10.1016/j.apsusc.2015.06.193.
- Nosal, W. H.; Thompson, D. W.; Yan, L.; Sarkar, S.; Subramanian, A.; Woollam, J. A. UV-vis-infrared Optical and AFM Study of Spin-Cast Chitosan Film. Colloids and Surfaces B-Biointerfaces 2005, 43, 131–137. DOI: 10.1016/j.colsurfb.2004.08.022.