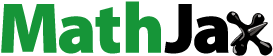
ABSTRACT
The pH, thermal, urea, and trifluoroethanol treatments were used to denature yogurt antioxidant peptides, and their antioxidant capacities and secondary structures were analysed. Results showed that yogurt peptides treated at pH 6.0 or above 50°C exhibited higher antioxidant activity than the control, whereas trifluoroethanol treatment resulted in a reduction and urea treatment held invariability. Peptide secondary configuration analyses revealed that these treated peptides significantly decreased their α-helical conformation but enhanced the β-sheet presence as well as an obvious alteration on β-turn and random coil structures. These peptide conformation alterations contributed to their antioxidant property increase.
Introduction
Milk protein is one of the most important food proteins that can provide necessary nutrients for human health.[Citation1] Protein in milk mainly consists of casein and whey protein, and these primary nutrients have been confirmed to play important roles in affecting the physiological activity of human.[Citation2] Casein and whey protein are the major antioxidants that can provide milk with antioxidant properties.[Citation3] It has been reported that yogurt, a milk fermentation product, can exhibit stronger antioxidant capacity and better ion chelation activity than milk, and such an enhancement in yogurt result mainly from the formation of antioxidant peptides in yogurt.[Citation4] Amino acids composition and amino acids sequence directly determine the antioxidant capacity of antioxidant peptides in yogurt.[Citation5] Additionally, their secondary and tertiary structures also play significant roles in affecting the antioxidant properties of these peptides.[Citation6] Recent studies have been mainly made on the separation and purification of antioxidant peptides.[Citation7,Citation8] Meanwhile, the studies on the characterization of primary sequence of antioxidant peptides have been extensively investigated.[Citation9,Citation10] However, the investigation on how the secondary structures of antioxidant peptides affect their antioxidant properties has not been well documented to our best knowledge.
Protein secondary structure refers to the dimensional interactions in local segments of proteins/peptides, and the common secondary structure is mainly comprised of α-helix, β-sheet, β-turn, and random coil.[Citation11] The stability of these secondary structures in protein is mainly determined by noncovalent interactions, including hydrogen bonds, hydrophobic interactions, and ionic interactions.[Citation12] It has been reported that thermal, pH, salt, and denaturant treatments could significantly alter the secondary structure of the protein, which could result in a significant alteration on the biological and physiological properties of proteins.[Citation13,Citation14] For instance, it has been reported that the bioactivity of milk-derived antibacterial peptides was significantly correlated with their secondary structures, and the conversion from α-helix to β-sheet in their secondary structures could result in a significant improvement on their inhibitory capacity to Gram positive and negative bacteria.[Citation15] However, the effect of the secondary structures alteration in yogurt antioxidant peptides on their antioxidant properties remained to be unveiled.
Thermal, pH, and denaturant treatments have been reported to alter inter- and intra-molecular interactions of casein, whey protein and other peptides in milk and yogurt, which could result in an alteration on the secondary and tertiary conformations in protein.[Citation16,Citation17] As a result, the antioxidant activity of these peptides could be altered. In the present study, we isolated antioxidant peptides in yogurt and denatured these peptides through pH, thermal, and denaturant treatments. Furthermore, the antioxidant activities and secondary structures of these treated peptides were measured and compared. The objective of this study was to investigate how peptides secondary structures affected the antioxidant property of peptides. The findings from this study could provide useful information on antioxidant capacity improvement of peptides through altering their secondary structures.
Materials and methods
Materials
Skim milk powder was provided from Zhejiang Yiming Food Co., Ltd. (Wenzhou, Zhejiang, China). Commercial lactic culture YF-L822 was a product of Christian Hansen (Hørsholm, Denmark). 2,2ʹ-Azino-bis(3-ethylbenzothiazoline-6-sulfonic acid) (ABTS) and 6-hydroxy-2,5,7,8-tetramethylchroman-2-carboxylic acid (Trolox) were purchased from Sigma-Aldrich (Steinheim, Germany). All other reagents and chemicals used in the present study were of analytical grade and purchased from Huadong Medicine Co., Ltd. (Hangzhou, Zhejiang, China).
Antioxidant peptides isolation in yogurt
Yogurt protein preparation was followed by a previously published method.[Citation4] In brief, milk powder (100 g) was mixed with 1,000 mL deionized water with 3.3% (w/v) protein content. The milk sample was then heated at 90°C for 5 min and then cooled to 43°C. Afterwards, the milk sample was inoculated with the lactic culture YF-L822 under a 2% (w/w) ratio, and then transferred to 200-g capacity yogurt cups. The fermentation took place at 43°C for 6 h until the pH of yogurt reached to 4.6. The yogurt sample was stored at 4°C for 24 h, and then homogenized at 1,600 rpm for 10 min. Subsequently, the homogenized yogurt sample was then centrifuged at 2,000 × g for 20 min to collect the supernatant. The supernatant was lyophilized to yield yogurt antioxidant peptides. The protein content in the yogurt antioxidant peptides was measured under the Kjeldahl nitrogen method.[Citation18] The yogurt peptides powder was stored at −20°C prior to the denaturation treatments.
Denaturation of antioxidant peptides
The antioxidant peptides isolated from yogurt were further denatured under pH, thermal, urea, or trifluoroethanol treatment. For pH treatment, the yogurt peptide powder (2.0 mg) was dissolved in 1.0 mL of 0.01 M Na2HPO4-NaH2PO4 buffer, and then its pH was adjusted to 6.0, 7.0, or 8.0. The resultant peptide samples were measured regarding their antioxidant property and structural characteristics. Regarding the thermal treatment, the yogurt peptide powder (2.0 mg) was added in 1.0 mL deionized water. The resultant mixture was incubated at 38°C, 50°C, or 65°C in a water bath for 5 min. Afterwards, the antioxidant property and secondary structure of the sample were measured. For the effect of the urea treatment, 2.0 mg yogurt peptide powder was dispersed in 1.0 mL of 1, 3, 5, 7, or 8 M urea solution. The resultant dispersion was kept in a 25°C water bath for 4 h before the antioxidant capacity analysis and secondary structure of the peptides were analysed. For the trifluoroethanol treatment, 2.0 mg yogurt peptide powder was dissolved into 1.0 mL of 10%, 30%, or 50% (v/v) trifluoroethanol solution. Subsequently, the sample was incubated at 25°C for 30 min in a water bath. The antioxidant activity and structural features of the yogurt peptide samples were analysed.
ABTS+ scavenging capacity
The ABTS+ scavenging capacity of the yogurt peptide samples was measured using ABTS radical cation de-colouration assay according to a published method.[Citation19,Citation20] Briefly, the ABTS radical cation stock solution was freshly prepared through mixing 7 mM ABTS aqueous solution and 2.45 mM K2S2O8 solution in the darkness for 16 h. The resultant solution was diluted in 0.1 M phosphate buffer at pH 7.4 to ensure its absorbance of 0.70 at 734 nm on a UNICO-2102PC UV-VIS spectrophotometer (UNICO, Dayton, NJ, USA). Afterwards, the yogurt peptide sample (0.1 mL) was incubated with 3.9 mL of the ABTS working solution at 30°C for 6 min and then the absorbance was recorded at 734 nm on the same spectrophotometer. The ABTS+ scavenging analysis was carried out in triplicate. The percentage of ABTS scavenge was calculated using the equation below,
Percentage ABTS scavenge (%) =
where Ai and Af are the initial and final absorbance of the sample, respectively. The yogurt peptide without any treatment was used as control. Trolox was used as the external standard with its concentration of 0–100 µM in water. The percentage ABTS scavenging capacity of peptides was expressed as µM Trolox equivalents antioxidant capacity (TEAC)/g,
TEAC (μmol/g) =
where CTrolox and Csample are Trolox equivalent concentration (μM) and peptides concentration (mg/mL), respectively.
Lipid peroxidation inhibition assay
Lipid peroxidation inhibitory activity of these yogurt peptide samples was assessed according to a published method with minor modifications.[Citation21] Briefly, the freeze-dried yogurt protein sample (2.0 mg) was mixed with 1.0 mL of 0.1 mM phosphate buffer (pH 7.0) containing 0.2% homogeneous lecithin. The mixture was then brought to 3.0 mL with deionized water, and further mixed with 1.5 mL of acetic acid: water (2:8, v/v) and 0.8% thiobarbituric acid (v/v) solution containing 1.0 M NaOH and 0.61 M trichloroacetic acid. Afterwards, the mixture was incubated at 95°C for 1 h and then cooled down to room temperature. Butanol (5.0 mL) was mixed with the sample and the upper phase was collected. Anhydrous sodium sulfate (1.0 g) was added to the extract to remove the residual water. Subsequently, the resultant extract was centrifuged at 1,500 × g for 10 min, and the absorbance of the supernatant was recorded at 532 nm on a UNICO-2102PC UV-VIS spectrophotometer (UNICO, Dayton, NJ, USA). Deionized water (1.0 mL) was used as the blank. The yogurt peptide without any treatment was used as the control. The lipid peroxidation inhibition of the peptides sample was carried out in triplicate and expressed as the inhibitory concentration of peptides that inhibited 50% of the substrate (IC50).
Fluorescence spectroscopy
F-4500 Fluorescence Spectrometer (Hitachi, Tokyo, Japan) was used to characterize the fluorescence features of these yogurt peptide samples according to a published method.[Citation22] In brief, 0.2 mg/mL of the sample was recorded in an emission spectrum range of 250 to 450 nm under an excitation wavelength at 280 nm. The scan rate was set at 60 nm/min and the triplicate test was carried out. Each replicate was measured five times to yield the average result.
Circular dichroism spectroscopy
The secondary structure of these yogurt peptide samples was analysed using J815 circular dichroism spectrometer (Jasco, Easton, MD, USA) regarding a published method.[Citation23] The spectrum of the yogurt peptide sample (0.2 mg/mL) was scanned at a wavelength range of 181–350 nm with a scan rate of 100 nm/min under a resolution of 0.1 nm at 25°C. Triplicate test was conducted, and each replicate was measured three times to obtain the average value.
Fourier transform infrared (FTIR) spectroscopy
The freeze-dried yogurt peptide sample (1.0 mg) was mixed with 100 mg of pure KBr. Afterwards, the mixture was ground into fine powder and then analysed on a Thermo Nicolet Nexus 470 FTIR (Thermo Nicolet, Madison, WI, USA). Pure KBr powder was used as the background. The FTIR spectra of the sample were obtained over a wave number range of 400 to 4,000 cm−1 with a resolution of 4 cm−1. Peakfit Version 4.12 (Seasolve Software Inc, San Jose, CA, USA) was used to correct the baseline of amide I band (1600–1700 cm−1). Furthermore, the deconvolution was conducted using the same software on the interpretation of amide I band (1600–1700 cm−1) components. Gaussian curve fitting was based on the second derivative spectra. All experiments were performed in triplicate.
Statistical analysis
Data were expressed as mean ± standard deviation of triplicate tests. One-way analysis of variance (ANOVA) was carried out to judge the significant differences among the means under Tukey test at a 0.05 significant level under OriginPro7.5 (OriginlabCorp., Wellesley Hills, MA, USA).
Results and discussion
Antioxidant activity of different treated yogurt peptides
displays the antioxidant properties of the yogurt peptides in different pH conditions. The peptides at pH 6.0 and 8.0 exhibited lower ability on inhibiting the ABTS+ radicals compared to the control. It should be aware that the sample at pH 7.0 did not show the significant difference in the TEAC value compared to the control or the peptide sample at pH 6.0 and 8.0. However, its lipid peroxidation inhibitory ability was significantly reduced in comparison with those samples. It was observed that the highest lipid peroxidation inhibition was found in the peptide sample at pH 6.0. These indicated that weak acid condition might enhance the antioxidant property of yogurt peptides than the neutral and basic pH conditions. shows the estimated secondary structures of yogurt antioxidant peptides. Under weak acid conditions, α-Helix conformation percentage was slightly lower than others and α-Helix/β-Structure ratio exhibited the similar trend. So, we suggested that proper low α-Helix and high β-Structure (β-sheet and β-turn) might be favourable to yogurt peptide exerting antioxidant activity.
Table 1. Estimated secondary structures of yogurt antioxidant peptides.
Figure 1. Antioxidant capacity of yogurt antioxidant peptides under the following treatments: (A) pH6, pH7, and pH8; (B) heat treat with 38°C, 50 oC, and 65 oC; (C) urea on the concentration of 1mol/L, 3mol/L,5mol/L,7mol/L, and 8mol/L; (D) trifluoroethanol on the concentration of 10%, 30%, and 50% (v/v). Different label letters indicate significant differences among samples at a significant level of 0.05, and the same label alphabet means insignificant.
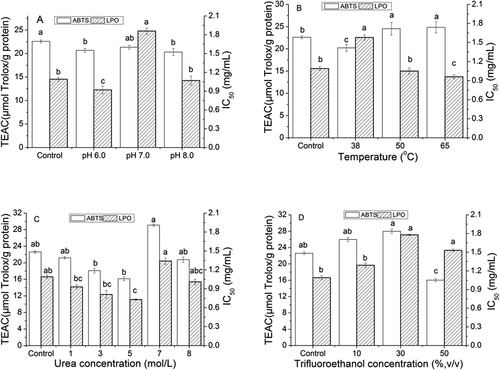
An increase in the ABTS+ scavenging ability of the yogurt peptides was observed with an increase of the incubation temperature (). Similarly, the thermal treatment with above 50 oC also resulted in an increase of the lipid peroxidation inhibitory capacity of these samples. It should be noted that the yogurt peptide sample incubated at 50°C and 65 oC exhibited higher activity on the ABTS+ scavenge and lipid peroxidation inhibition than the control. These indicated that the thermal treatment increased the antioxidant properties of these peptides by enhancing their proton and electron donations. Meanwhile, in , β-Turn conformation at 50°C and 65 oC has a higher percentage than the control. Especially, yogurt peptide sample treated at 65°C was found the lowest α-Helix and α-Helix/β- structure ratio. So, we suggested that the thermal treatment above 50 oC could also loosen the conformation of yogurt peptide molecules by altering the inter- and intra-molecular noncovalent interactions, such as hydrogen bonds and hydrophobic interactions.[Citation13,Citation24] These conformation alterations might expose hydrophobic groups of the peptides on the surface, leading to the enhancement of the peptides antioxidant property. It is similar to pH adjustment and more β-structure is beneficial to the increase of yogurt peptide antioxidation.
The yogurt peptide samples treated with different concentrations of urea exhibited the similar activity on inhibiting the ABTS+ radicals in the present study compared to the control (). However, the increase in the urea concentration from 1 to 5 mol/L in the sample increased the inhibition of their lipid peroxidation. The yogurt peptide sample treated with the 5 mol/L urea showed significantly higher inhibitory ability on the lipid peroxidation compared to the control. shows that α-Helix conformation on yogurt peptides dramatically decreased with the existence of urea (1–8 mol/L) compared to the control. Among all urea treated peptides, β-Structure (β-sheet and β-turn) percentages were significantly higher than the control without concentration-dependent. It seemed to be different from pH adjustment and thermal treatment. Yogurt peptides with the lowest α-Helix/β-Structure ratio could not exhibit the highest antioxidant capability. To the contrary, ABTS+ radicals scavenging ability was almost lower than the control. Then, we suggested that organic molecule like urea could destroy hydrogen bond inside the protein or peptide molecule and compete to be as the proton donor or receptor. So, yogurt peptide interacting with urea molecule displayed high β-structure and inconsistent activity increase or decrease according to different antioxidation analysis indices. In addition, it should be aware that the 8 mol/L urea addition did not significantly change the antioxidant activity of the sample. It has been reported that the effective urea concentration that could cause the denaturation of protein ranged of 4–6 mol/L.[Citation25,Citation26] In the present study, no precipitant was found in the urea treated samples, indicating that their antioxidant property alteration did not result from the aggregation of the peptide molecules in the present study.
Compared with the control, increasing the concentration of trifluoroethanol resulted in a decrease of the antioxidant activity of these yogurt peptide samples (). Particularly, the significant reduction on both ABTS+ scavenging and lipid peroxidation inhibitory capacity was observed in the yogurt peptide sample treated with 50% (v/v) trifluoroethanol, whereas the other trifluoroethanol treated peptide samples exhibited the similar ability as the control on scavenging ABTS+ radicals and inhibiting the lipid peroxidation. Meanwhile, secondary structure analysis in showed that peptide with the existence of trifluoroethanol exhibited significantly higher β-sheet and lower β-turn conformation than the control. Yogurt peptides α-Helix/β-Structure ratio kept almost identical at trifluoroethanol concentration range 10%-50% (v/v). Obviously, trifluoroethanol treatment played an adverse role in yogurt peptide antioxidation exerting even if the loosen secondary structures (β-sheet and β-turn) slightly increased. We suggested that β-turn conformation might perform a crucial factor. Trifluoroethanol reduced significantly the percentage of β-turn and resulted in the decrease of yogurt peptide antioxidant ability. The similar alteration was found for thermal treatment peptide sample above 50 oC. It has been known that trifluoroethanol is a critical denaturant that could disrupt the secondary structure of proteins, especially α-helix structure.[Citation14] Meanwhile, trifluoroethanol has been reported to stabilize hydrogen bonds in protein structure, which could prevent hydrophobic groups in protein from the exposure. As a result, the antioxidant property of protein could be weakened.[Citation17]
It was observed that these peptide samples after denaturation possessed different antioxidant properties compared to the control. Within peptide samples, antioxidant peptides have been isolated and identified in our pervious published work.[Citation27]In present work, yogurt peptides samples are mixtures of various molecular weight peptides. Obviously, the antioxidant activity of yogurt peptides was impacted by physicochemical conditions and other co-components, such as pH, thermal, organic solvent or denaturant and other peptides or proteins. Since these denaturants have been also reported to affect the structure of proteins/peptides, we hypothesized that it might be the alteration on the peptide secondary conformations that cause the variation on the peptides antioxidant capacity.[Citation14] To elucidate such a correlation, we further investigated the secondary structure features of these peptide samples.
Fluorescence spectrum of different treated yogurt peptides
Aromatic amino acids, such as tryptophan, tyrosine and phenylalanine, have been confirmed to play an essential role in the determination of the fluorescent feature of proteins/peptides.[Citation22] It has been known that milk protein has an isoelectronic point of 4.5–5.2.[Citation1] Inorganic ions incorporation in protein could enhance the hydration, which could enhance the polarity of the aromatic amino acids in protein structure.[Citation24] This could lead to a structural change in protein and thus alter the fluorescent feature of protein.[Citation28] In the present study, adjusting the yogurt peptides to pH 6.0, 7.0, and 8.0 indeed shifted the maximal fluorescent wavelength from 331 nm (the control) to 335 nm, 336 nm, and 338 nm, respectively ().
Figure 2. Fluorescence spectrum of yogurt antioxidant peptides under the following treatments: (A) pH6, pH7, and pH8; (B) heat treat with 38°C, 50 oC, and 65 oC; (C) urea on the concentration of 1mol/L, 3mol/L,5mol/L,7mol/L, and 8mol/L; (D) trifluoroethanol on the concentration of 10%, 30%, and 50% (v/v). Different label letters indicate significant differences among samples at a significant level of 0.05, and the same label alphabet means insignificant.
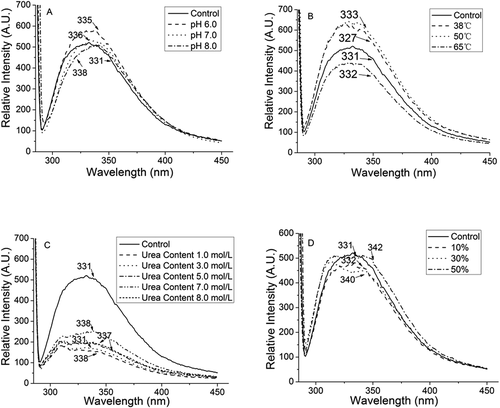
Temperature did not significantly cause the spectrum shift of these yogurt peptide samples (). However, the yogurt peptide sample treated at 65°C lost its fluorescent feature. That might be because high temperature could significantly reduce the α-helical configurations in the secondary structure of peptides, and β-sheet and random coil structures might be enhanced.[Citation29] This could help to loosen peptide secondary structure and expose their hydrophobic groups to elevate the antioxidant ability of peptides.[Citation24] However, such high temperature could trigger the destruction of these aromatic amino acids residues in the peptides, which cause the disappearance of the fluorescent property in the peptide heated at 65°C.
The addition of urea in the yogurt peptide samples was found to quench the fluorescent ability of the yogurt peptide samples (). It has been reported that urea could form a layer on the surface of protein structure, which could reduce the contact between peptides and water molecules but enhance the contact between peptides and hydrophobic compounds.[Citation25] As a result, the lipid peroxidation inhibitory and radical scavenging activity of these peptides could be expected to be enhanced. However, the increase of urea concentration in the peptide samples might embed aromatic amino acid residuals in the core of peptides, which decreased the fluorescent property of the peptides.[Citation22]
The increase of the trifluoroethanol concentration at the range of 10%-50% (v/v) in the yogurt peptide samples significantly shifted the maximal fluorescent spectrum of the peptides, and the maximal wavelength was observed at 342 nm when the 50% (v/v) trifluoroethanol concentration was applied to the sample (). In , α-helix and β-Turn conformation were discovered to decrease while β-Sheet significantly increase. This might be because trifluoroethanol molecules could be assembled on the surface of peptide molecules, which could inhibit the hydration of peptide and changed the driving force to form secondary structure. So, secondary structure profile was altered.
FTIR spectra of different treated yogurt peptides
FTIR spectra have been widely used to indicate the driving force in secondary structure of protein molecules.[Citation17] exhibits the FTIR spectrum of these different treated yogurt peptide samples. A wider and stronger absorption peak around 3400 cm−1 was observed in the samples treated with different pH, thermal, and trifluoroethanol concentration conditions. These indicated that these peptide molecules contained numerous inter- and intra-molecular hydrogen bonds. For instance, the trifluoroethanol treated yogurt peptide sample, compared to the control, significantly shifted its peak to 3427–3434 cm−1. Such peak shifts in the samples could be because trifluoroethanol molecules could substitute water molecules on the surface of the protein, and the aggregation of trifluoroethanol molecules could alter the hydrogen bonds formed between water molecules and the C = O groups in peptides/proteins.[Citation14] Additionally, a significant alteration was observed on the peak with a wave number of 3600–3300 cm−1 in the urea treated yogurt peptide samples, which could be attributed to the stretching vibration of the N-H groups in urea to the peptide molecules.
Figure 3. FTIR spectrum of yogurt antioxidant peptides under the following treatments: (A) pH6, pH7, and pH8; (B) heat treat with 38°C, 50 oC, and 65 oC; (C) urea on the concentration of 1mol/L, 3mol/L,5mol/L,7mol/L, and 8mol/L; (D) trifluoroethanol on the concentration of 10%, 30%, and 50% (v/v). Different label letters indicate significant differences among samples at a significant level of 0.05, and the same label alphabet means insignificant.
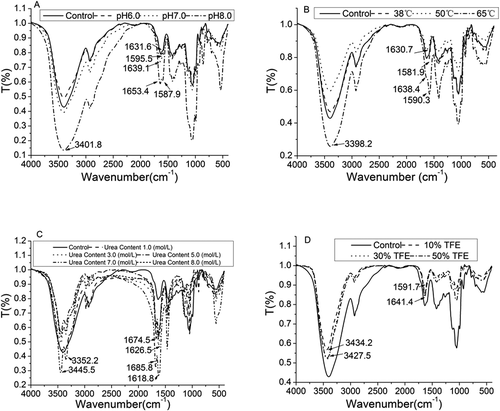
The yogurt peptides exhibited a typical amide I and II peak at 1700–1600 cm−1 and 1600–1500 cm−1, respectively. The absorption of the amide I peak resulted from the stretching vibration of the carbonyl groups, whereas the C-N and the N-H vibrations resulted in the formation of the amide II. Amide I include numerous information about peptide secondary conformation.[Citation17] So, characterization of amide I was applied to analyse peptide secondary structure. FTIR spectra profile of Amide I and Amide II indicated peptide fold behavior. It should be aware that the amide I in the control exhibited a peak at 1631.6 cm−1 (). The yogurt peptide sample treated at pH 6.0 also displayed a similar amide I peak as the control. However, the sample treated at pH 8.0 shifted the amide I peak to 1653.4 cm−1. Meanwhile, the amide II peak in this pH treated yogurt peptide sample appeared at 1587.9 cm−1 compared to that in the control (1595.5 cm−1).
The thermal treatment to the yogurt peptide sample at 38°C to 50°C did not alter the peak absorption of the amide I, and these samples exhibited the amide I peak around 1631 cm−1 (). However, the yogurt peptide sample treated at 65°C showed the amide I peak at 1638.4cm−1. In the meantime, the amide II peak was shifted to 1590.3cm−1. It has been reported that high temperature could induce the damage of hydrogen bonds in the protein, which could accelerate α-helical conformation to be converted into β-sheet, β-turn, and random coil configurations.[Citation29] The shifts of amide I and II were an indication of peptide secondary structure change.
It was clearly observed that the urea treatment significantly altered the peak absorption of the amide I and II in the yogurt peptide samples (). For instance, the amide I peak in the yogurt peptide samples was shifted to 1674–1685 cm−1 along with the increase of the urea concentration in the samples. It has been reported that urea peak can be absorbed around 1700–1600 cm−1 as the amide I in protein, which could bias the characterization of protein secondary structure.[Citation30] However, urea has been confirmed to form hydrogen bonds with water molecules, which could alter the polarity of protein molecules and improve their hydrophobic property.[Citation26] A further study needs to be carried out to elucidate these speculations.
The yogurt peptide samples treated with the different concentrations of trifluoroethanol exhibited a significant shift on the amide I and II peak (). For example, the amide I peak in these trifluoroethanol treated yogurt peptides was shifted to 1641.4 cm−1, whereas the absorption of the amide II peak was observed at 1591.7 cm−1. Obviously, we could not draw a conclusion between amide I and II shifts to higher wavenumber or lower wavenumber and peptide antioxidant activity. As we have discussed earlier in , amide I and II spectra peaks of peptides treated with pH, thermal, urea and trifluoroethanol did not show significant shift trend and explicit directions to interpret antioxidation alteration mechanism. So, peptide secondary conformation profiles were analysed.
Secondary structure of different treated yogurt peptides
It has been known that the major secondary structure of a protein/peptide molecule consists of α-helix, β-sheet, β-turn, and random coil.[Citation17] These secondary configurations are mainly present in the amide I of protein. In detail, the featured peak of α-helix was at 1646 to 1664 cm−1, whereas the peaks at 1610–1640 cm−1 and 1682–1700 cm−1 mainly resulted from β-sheet. The β-turn typical peak has been reported to be absorbed at 1664–1681 cm−1, and the random coil configuration peak can be found in 1637–1645 cm−1.[Citation31]
and display the percentage of these secondary structures in these different treated yogurt peptide samples. The control sample contained α-helical configuration as the major secondary structure, which represented about 33% of the total secondary structures (). The yogurt peptide samples treated at pH 6.0 to 8.0 showed the similar α-helix percentage as the control. However, heating the yogurt peptide sample to 65°C dramatically reduced the percentage of α-helix structure to 16.61% (). Similarly, the α-helical configuration was found to be reduced in the yogurt peptide sample treated with urea and trifluoroethanol. It has been accepted that α-helix conformation is the most stable secondary structure in protein, and the cleavage on hydrogen bonds in the α-helical structure could be considered the major reason to damage the integrity of α-helix.[Citation17] Therefore, we speculated that thermal, urea, and trifluoroethanol treatment could accelerate the destruction of α-helix in the yogurt peptides through cleaving the hydrogen bonds in the α-helical structure. But according to , the decrease of α-helix conformation ratio did not necessarily enhance peptide antioxidant ability. β-Sheet mainly exists in the folding area of protein, and aggregation of β-lactoglobulin and α-lactalbumin has been reported to play an essential role in increasing β-sheet configuration in protein.[Citation32] It has been reported that β-sheet structure in protein can be converted into β-turn, and β-turn could further be turned into random coil structure.[Citation24] In the present study, the yogurt peptide sample at pH 6.0 contained a much higher percentage of the β-sheet structure compared to the control. In addition, the higher percentage of the β-sheet and β-turn configurations was also observed in the yogurt peptide sample treated at 65°C. It has been known that the α-helix/β-structure ratio could be used to indicate the flexibility of protein.[Citation17] In the present study, the yogurt peptide sample treated at 65°C showed a significantly lower α-helix/β-structure ratio compared with the control. This might be because high temperature could result in denaturation of protein molecules, such as β-lactoglobulin, which could induce the aggregation of these denatured protein molecules.[Citation33] Based on antioxidant activity results in , peptides composed of lower α-Helix/β-Structure ratio might exhibit higher antioxidant property under pH adjustment and thermal treatment conditions. However, this conclusion was conflicting in the situation of urea and trifluoroethanol treatment. The urea and trifluoroethanol treated samples possessed a higher percentage of the β-sheet structure compared to the control, and their flexibility value ranged of 0.24–0.68 (). And the lower flexibility value was, the higher antioxidant activity was not necessary. We suggested that urea and trifluoroethanol molecules interacted with yogurt peptides, which contributed to molecular spatial effect (such as steric hindrance) except for alteration peptide secondary conformation. Regarding the random coil conformation, it accounted for 15.88% of the whole secondary structures in the control. The similar random coil structure percentage was found in the yogurt peptide samples treated at pH 6.0 to 8.0. Heating the yogurt peptide sample at 50°C did not alter its random coil percentage compared to the control. However, the sample treated at 65°C exhibited a much higher random coil percentage. Similarly, the trifluoroethanol treated yogurt peptide samples also showed a significantly higher percentage of the random coil structure in their secondary structure. These indicated that high temperature and trifluoroethanol treatments dramatically turned the α-helical conformation into the random coil. It seemed that random coil structure was not beneficial to peptide antioxidation improvement.
Figure 4. Spectrum of amide I (1700–1600 cm−1) in of yogurt antioxidant peptides: (A) Control, (B) pH 6.0 yogurt peptide, (C) pH 7.0 yogurt peptide, (D) pH 8.0 yogurt peptide, (E) 38°C treated yogurt peptide, (F) 50°C treated yogurt peptide, (G)65°C treated yogurt peptide, (H) 1.0 mol/L urea treated yogurt peptide, (I) 3.0 mol/L urea treated yogurt peptide, (J) 5.0 mol/L urea treated yogurt peptide, (K) 7.0 mol/L urea treated yogurt peptide, (L) 8.0 mol/L urea treated yogurt peptide, (M) 10% (v/v) trifluoroethanol treated yogurt peptide, (N) 30% (v/v) trifluoroethanol treated yogurt peptide, and (O) 50% (v/v) trifluoroethanol treated yogurt peptide. Different label letters indicate significant differences among samples at a significant level of 0.05, and the same label alphabet means insignificant.
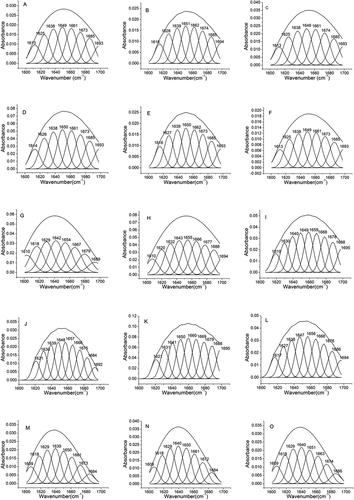
exhibits the circular dichroism spectrum of these yogurt peptide samples. It should be noted that urea significantly increased the detection voltage of the circular dichroism spectrometer, which made the detected secondary structure of the samples not reliable.[Citation12] In the control sample, two negative peaks were found at 208 and 222 nm, which resulted from the α-helical conformation in the peptides. Similarly, the β-sheet structure in the peptides resulted in the presence of a positive peak at 194 nm and a negative peak at 216 nm. The random coil structure showed a featured peak at 220 nm, whereas the peak at 206 nm represented the β-turn structure in the peptides. Temperature, pH, and trifluoroethanol treatments obviously altered the absorption wavelength, shape, and intensity of these peaks in the peptides, demonstrating that these treatments affected the secondary structures of the yogurt peptide samples. These results were consistent with the results in . These illustrated that peptide secondary structure alteration affected the antioxidant capacity of yogurt peptide samples.
Figure 5. Circular dichromatic spectrogram of yogurt antioxidant peptides under the following treatments: (A) pH6, pH7, and pH8; (B) heat treat with 38°C, 50 oC, and 65 oC; (C) urea on the concentration of 1mol/L, 3mol/L,5mol/L,7mol/L, and 8mol/L; (D) trifluoroethanol on the concentration of 10%, 30%, and 50% (v/v). Different label letters indicate significant differences among samples at a significant level of 0.05, and the same label alphabet means insignificant.
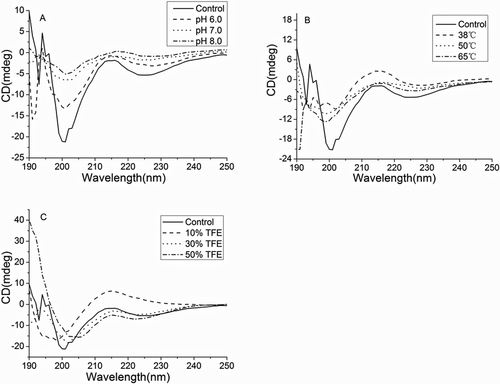
Conclusion
In conclusion, pH, thermal, urea, and trifluoroethanol treatments altered the antioxidant activity of yogurt peptides, which mainly resulted from the changes of peptide secondary structures. These treatments decreased the percentage of α-helix structure but increased β-sheet and random coil configuration. Yogurt peptide secondary conformation contributed to its antioxidation effect. As it has been well known that peptide primary structure plays a critical role in its secondary conformation. So, the results could be helpful to targeted hydrolysis of milk protein and obtain efficient antioxidant activity peptide. In addition, physicochemical elements during yogurt production could be optimized to improve the milk peptide functional effect.
Additional information
Funding
References
- Power, O.; Jakeman, P.; FitzGerald, R. J. Antioxidative Peptides: Enzymatic Production, in Vitro and in Vivo Antioxidant Activity and Potential Applications of Milk-Derived Antioxidative Peptides. Amino Acids 2013, 44, 797–820. DOI: 10.1007/s00726-012-1393-9.
- Murray, B. A.; FitzGerald, R. J. Angiotensin Converting Enzyme Inhibitory Peptides Derived from Food Proteins: Biochemistry, Bioactivity and Production. Current Pharmaceutical Design 2007, 13, 773–791.
- Irshad, I.; Kanekanian, A.; Peters, A.; Masud, T. Antioxidant Activity of Bioactive Peptides Derived from Bovine Casein Hydrolysate Fractions. Journal of Food Science and Technology 2013, 1, 1–9.
- SabeenaFarvin, K. H.; Baron, C. P.; Nielsen, N. S.; Jacobsen, C. Antioxidant Activity of Yoghurt Peptides: Part 1-In Vitro Assays and Evaluation in ω-3 Enriched Milk. Food Chemistry 2010, 123, 1081–1089.
- SabeenaFarvin, K. H.; Baron, C. P.; Nielsen, N. S.; Otte, J.; Jacobsen, C. Antioxidant Activity of Yoghurt Peptides: Part 2-Characterisation of Peptide Fractions. Food Chemistry 2010, 123, 1090–1097.
- Hernández-Ledesma, B.; Davalos, A.; Bartolomé, B.; Amigo, L. Preparation of Antioxidant Enzymatic Hydrolysates from α-lactalbumin and β-lactoglobulin. Identification of Active Peptides by HPLC-MS/MS. Journal of Agricultural and Food Chemistry 2005, 53, 588–593.
- Gómez-Ruiz, J. A.; López-Expósito, I.; Pihlanto, A.; Ramos, M.; Recio, I. Antioxidant Activity of Ovine Casein Hydrolysates: Identification of Active Peptides by HPLC-MS/MS. European Food Research and Technology 2008, 227, 1061–1067.
- Beermann, C.; Euler, M.; Herzberg, J.; Stahl, B. Anti-Oxidative Capacity of Enzymatically Released Peptides from Soybean Protein Isolate. European Food Research and Technology 2009, 229, 637–644.
- Chi, C.; Hu, F.; Wang, B.; Ren, X. J.; Deng, S. G. Purification and Characterization of Three Antioxidant Peptides from Protein Hydrolyzate of Croceine Croaker (Pseudosciaena Crocea) Muscle. Food Chemistry 2015, 168, 662–667.
- Wang, C.; Li, B.; Wang, B.; Xie, N. Degradation and Antioxidant Activities of Peptides and Zinc-Peptide Complexes during in Vitro Gastrointestinal Digestion. Food Chemistry 2015, 173, 733–740.
- Lin, S. Y.; Liang, R.; Xue, P. Y.; Zhang, S. Y.; Liu, Z. Y.; Dong, X. P. Antioxidant Activity Improvement of Identified Pine Nut Peptides by Pulsed Electric Field (PEF) and the Mechanism Exploration. LWT - Food Science and Technology 2017, 75, 366–372.
- Han, Y.; Wang, J.; Li, Y.; Hang, Y.; Yin, X.; Li, Q. Circular Dichroism and Infrared Spectroscopic Characterization of Secondary Structure Components of Protein Z during Mashing and Boiling Processes. Food Chemistry 2015, 188, 201–209.
- Ma, Y. R.; Zhou, M.; Huang, H. H. Changes of Heat‑Treated Soymilks in Bioactive Compounds and Their Antioxidant Activities under in Vitro Gastrointestinal Digestion. European Food Research and Technology 2014, 239, 637–652.
- Wall, J. S.; Williams, A.; Wooliver, C.; Martin, E. B.; Cheng, X. L.; Eric Heidel, R.; Kennel, S. J. Secondary Structure Propensity and Chirality of the Amyloidophilic Peptide P5 and Its Analogues Impacts Ligand Binding- in Vitro Characterization. Biochemistry and Biophysics Reports 2016, 8, 89–99.
- Zhou, N.; Tieleman, D. P.; Hans, J. V. Molecular Dynamics Simulations of Bovine Lactoferricin Turning a Helix. BioMetals 2004, 17, 217–223.
- Liu, T. X.; Zhao, M. M. Physical and Chemical Modification of SPI as a Potential Means to Enhance Small Peptide Contents and Antioxidant Activity Found in Hydrolysates. Innovative Food Science and Emerging Technologies 2010, 11, 677–683.
- Cian, R. E.; Vioque, J.; Drago, S. R. Structure–Mechanism Relationship of Antioxidant and ACE I Inhibitory Peptides from Wheat Gluten Hydrolysate Fractionated by pH. Food Research International 2015, 69, 216–223.
- Guebe, D. V.; Nudel, B. C.; Giulietti, A. M. A Simple and Rapid micro-Kjeldahl Method for Total Nitrogen Analysis. Biotechnology Techniques 1991, 5, 427–430.
- Zulueta, A.; Esteve, M. J.; Frı´Gola, A. ORAC and TEAC Assays Comparison to Measure the Antioxidant Capacity of Food Products. Food Chemistry 2009, 114, 310–316.
- Cian, R. E.; Martínez-Augustin, O.; Drago, S. Bioactive Properties of Peptides Obtained by Enzymatic Hydrolysis from Protein Byproducts of Porphyra Columbina. Food Research International 2012, 49, 364–372.
- Seo, H. S.; Kwak, S.; Lee, Y. S. Antioxidative Activities of Histidine Containing Caffeic Acid-Dipeptides. Bioorganic & Medicinal Chemistry 2010, 20, 4266–4272.
- Vivian, J. T.; Callis, P. R. Mechanisms of Tryptophan Fluorescence Shifts in Proteins. Biophysical Journal 2001, 80, 2093–2109.
- Lin, S. Y.; Liang, R.; Li, X. F.; Xing, J.; Yuan, Y. Effect of Pulsed Electric Field (PEF) on Structures and Antioxidant Activity of Soybean Source peptides-SHCMN. Food Chemistry 2016, 213, 588–594.
- Jandaruang, J.; Siritapetawee, J.; Thumanu, K.; Songsiriritthigul, C.; Krittanai, C.; Daduang, S.; Dhiravisit, A.; Thammasirirak, S. The Effects of Temperature and pH on Secondary Structure and Antioxidant Activity of Crocodylus Siamensis Hemoglobin. Protein Journal 2012, 31, 43–50.
- Zhang, Z. Y.; Zhu, Y. J.; Shi, Y. Y. Molecular Dynamics Simulations of Urea and Thermal-Induced Denaturation of S-Peptide Analogue. Biophysical Chemistry 2001, 89, 145–162.
- Wallqvist, A.; Covell, D. G.; Thirumalai, D. Hydrophobic Interactions in Aqueous Urea Solutions with Implications for the Mechanism of Protein Denaturation. Journal of the American Chemical Society 1998, 120, 427–428.
- Haina, Y.; Jianmin, L.; Jinyan, G.; Hailong, X.; Guangsheng, Z.; Gongnian, X.; Hui, X.; Wenchao, W. Microbial Transglutaminase Enhances Antioxidant Activity Ofyogurt through Altering Pattern of Water-Soluble Peptides Andincreasing Release of Amino Acids. International Journal of Food Science and Technology 2018, 53, 1030–1044.
- Bonomi, F.; Mora, G.; Pagani, M. A.; Iametti, S. Probing Structural Features of Water-Insoluble Proteins by Front-Face Fluorescence. Analytical Biochemistry 2004, 329, 104–111.
- Wang, Z. J.; Li, Y.; Jiang, L. Z.; Qi, B.; Zhou, L. Relationship between Secondary Structure and Surface Hydrophobicity of Soybean Protein Isolate Subjected to Heat Treatment. Journal of Chemistry 2014, 47, 127–132.
- Zhang, Z. H.; Hua, Y. F. Urea-Modified Soy Globulin Proteins (7S and11S): Effect of Wett Ability and Secondary Structure on Adhesion. Journal of the American Oil Chemists’ Society 2007, 84, 853–857.
- Long, G.; Ji, Y.; Pan, H.; Sun, Z.; Li, Y. Characterization of Thermal Denaturation Structure and Morphology of Soy Glycinin by FTIR and SEM. International Journal of Food Properties 2015, 18, 763–774.
- Heni, B. W.; Nidhi, B.; Hilton, C. D. Stability of Whey Proteins during Thermal Processing: A Review. Food Science and Food Safety 2014, 13, 1235–1251.
- Dickinson, E.; Parkinson, E. L. Heat-Induced Aggregation of Milk Protein-Stabilized Emulsions: Sensitivity to Processing and Composition. International Dairy Journal 2004, 14, 635–645.