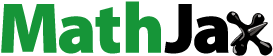
ABSTRACT
The effectiveness of two drying agents, namely whey protein isolate (WPI) and maltodextrin (MD), was evaluated during spray-drying of strawberry puree. With the increase of WPI substitution in the feed material, the surface tension of strawberry puree decreased, and powder recovery increased. Powder recovery (Rp) increased from 39.2 ± 2.3% (S:MD:WPI = 60:40:0) to 56.5 ± 2.8% when MD was replaced by WPI (S:MD:WPI = 60:39:1). Surface morphology of powders showed that the addition of WPI resulted in shrunken particle surface, which gave rise to smaller DB and particle size. The particles were not spherical, and even with the addition of 0.5% WPI, the particle morphology was altered. The surface shrinkage of strawberry powder increased with increase in WPI from 0.5% to 10%. The production efficiency of strawberry powder could be greatly improved when MD was replaced by 1% WPI.
Introduction
Spray drying is a well-established and widely used method for the transformation of a wide range of liquid food products into powder form. The advantages include cost-effectiveness, flexibility, and continuous process facilitating the production of good quality powder. [Citation1] However, stickiness easily occurs during the production of materials with a high proportion of low-molecular-weight sugars, such as fructose, glucose, and sucrose. The stickiness of sugars is mainly caused by low glass transition temperature (Tg). For example, the Tg of fructose, glucose, and sucrose is 16°C, 31°C, and 62°C, respectively. [Citation2,Citation3] Stickiness leads to considerable logistic challenges and economic loss during drying, which limits the application of spray drying for food and pharmaceutical materials. [Citation4,Citation5]
Some available ways to reduce stickiness problem include using low humidity air, mechanical scraping of the drying chamber wall, adding of drying aids such as maltodextrins, starch, which the most feasible method is adding maltodextrins. The high-molecular-weight maltodextrin (MD) increases the Tg of the product, preventing stickiness and reducing hygroscopicity. [Citation6] However, large quantities of drying additives such as MD (>40%) are required to convert the concentrated fruit juices.[Citation7] Addition of high levels of drying additives may alter flavour, taste, and colour of the original product. Another novel approach to minimize stickiness is to modify the surface properties of the droplets/particles with small amounts of protein. [Citation8] The preferential migration of proteins combined with dry film formation overcomes the stickiness of sugar-protein solutions. [Citation8] Generally, dairy proteins were more effective than plant proteins as they resulted in higher powder recoveries. [Citation9]
Strawberry (Fragaria x ananassa Duch.) fruits are one of the most popular and valuable fruits in the Chinese market because of their excellent flavour and taste, and high antioxidant activity of its polyphenols.[Citation10,Citation11] As strawberry fruits are a highly perishable product, it spoils quickly, which leads to substantial economic losses. Polyphenols are a group of phytochemicals sensitive to heat processing, spray drying might be a good option for drying of polyphenol-rich strawberry. Fang et al.[Citation12] reported that the retention of the total phenolic content and total anthocyanins during the spray drying process were about 96% and 94%.
Strawberry fruits contain a high proportion of low-molecular-weight sugars, such as fructose, glucose and sucrose, which can cause stickiness during spray drying. Studies investigating spray drying of strawberry puree using a combination of protein and maltodextrin as drying carrier agents may overcome this problem. Therefore, the aim of this study was to investigate the efficacy of MD combined with WPI to overcome the stickiness of strawberry puree during spray drying. We also analysed the role of WPI in spray drying of strawberry based on the surface tension of feed solution, physicochemical properties, Tg and microstructure of strawberry powder. Secondly, to study if a very small amount of protein addition is sufficient to reduce MD addition during spray drying strawberry powder. Physicochemical properties of spray-dried strawberry powder (surface tension of feed solution, powder recovery, moisture content, aw, Tg and microstructure) were analysed.
Materials and methods
Materials
Frozen strawberry (Cultivar Mei 13) was purchased from Laiyang Hai Te Er Food Co. Ltd. (Jinan, China). Whey protein isolate (WPI 90, protein content 90%) and MD of dextrose equivalent (DE) 10 were purchased from Hangzhou Puxiu Biological Technology Co., Ltd. (Hangzhou, China) and Hilmar Cheese Co. (California, USA).
Strawberry puree preparation
The frozen strawberry was thawed, and mashed with DS-1 high-speed tissue blender (Shanghai Biaoben Instrument Co., Shanghai, China). The strawberry purees were pulverized using a colloid mill (JM-FB60, Wenzhou Qixing Dairy Equipment, Zhejiang, China). The strawberry puree contained a total solid content (TSS) of 8.35 ± 0.12°Brix, pH 3.51 ± 0.03, moisture content 89.3 ± 0.3%, sugar content (by HPLC method) comprising fructose 2.9 ± 0.2%, glucose 2.7 ± 0.3%, and sucrose 1.2 ± 0.1%, and 1.13 ± 0.13% organic acids, along with 0.98 ± 0.09% fibre content .
Spray drying of strawberry puree with WPI and MD
The ratios of strawberry puree total solids, WPI, and MD were set at 70:30:0, 60:40:0, 50:50:0, 80:0:20, 70:0:30, respectively, based on dry mass. Then, the strawberry content was fixed at 60%, and the S:MD:WPI ratio was varied as follows: 60:40:0, 60:39.5:0.5, 60:39:1, 60:35:5, 60:30:10, 60:20:20, 60:10:30, and 60:0:40, respectively, based on dry solid mass. WPI and MD were dissolved in strawberry purees using a magnetic stirrer.
One hundred grams of solution was spray-dried for each run. The viscosity of feed solutions ranged from 603.8 to 4494.2 mPa s using NDJ-5S Digital Rotational Viscometer (Shanghai HengPing Scientific Instrument Co., LTD, spindle 2, speed 6 rpm/min). The experiments were carried out in a Büchi B-290 mini spray dryer (Büchi Labortechnik AG, Switzerland) with an aspirator rate of 100% (35 m3/h), atomization air rotameter of 30 mm (439 L/h) using a co-current flow, dry air inlet and outlet temperatures of 165 ± 1°C and 85 ± 1°C, respectively. The feed rate was 3 mL/min. After the completion of the experiment, the samples were collected from the product collection vessel. The powders were immediately sealed and stored in a desiccator. The spray-drying processes were performed in triplicate.
Surface tension (ST)
The surface tension of strawberry puree before spray drying was determined by QBZY-2 surface tension meter (Shanghai FangRui Instrument Co., China). Sample solutions were filled in a test vessel, and a platinum Wilhelmy plate was immersed into and raised out of the solutions slowly. The surface tension was recorded using the measuring software. The analysis was repeated five times with each sample.
Powder recovery
The powder recovery was calculated as the ratio of the mass of solids obtained after spray drying to the mass in the feed solution based on dry mass. DM denotes dry matter.
Moisture content and water activity (aw)
Moisture content was determined by gravimetric analysis (GB/T5009.3–2003). The samples of strawberry powder (1–2 g) were weighed in triplicate and dried in an air oven at 105°C for 12 h until a constant weight was attained. Water activity was measured using a Water Activity Meter (Model CR-400, Konika Minolta Sensing, Japan). The samples were analysed in triplicate, and the mean was recorded.
Bulk density (DB) and particle size
After transferring 2 g of powder to a 50-mL graduated cylinder, the bulk density was calculated by dividing the mass of the powder by its volume in the cylinder. The analysis was repeated five times for each sample. The particle size was measured using a laser particle size analyser (Dandong Bettersize Instruments Ltd., Liaoning, China) to determine the volume and mean diameter. A small amount of the powder sample was subjected to sonication for better dispersion of the powders. The dispersion media was the clean compressed air. The particle size and distribution was monitored during each measurement until successive readings were constant. The particle size was expressed as D[4,3], which represents the mean diameter of a sphere with the same volume and is commonly used to determine the particle size.
Glass transition temperature (tg)
The Tg of strawberry, MD, and WPI powders was determined using a TA Q20 differential scanning calorimeter (DSC) (TA Instruments, New Castle, Delaware, USA), equipped with a refrigerated cooling system. Powders (10 mg) were placed in the pre-weighed DSC aluminium pan, and hermetically sealed, using an empty pan as the reference. The liquid nitrogen was used for sample cooling before the runs. The powders were heated at the rate of 20°C/min between −20°C and 100°C in an inert atmosphere of nitrogen. Tg was determined as a change in heat flow in the DSC thermograms. The values of Tg were obtained from the Universal Analysis software (TA Instruments, New Castle, USA).
Particle morphology
The surface morphologies were observed using an FEI Nanosem 430 Scanning Electron Microscopy (SEM, FEI Co., Oregon State, USA). The images were obtained at a magnification of 5000× at room temperature.
Statistical analysis
One-way analysis of variance (ANOVA) and Duncan’s test (SPSS 18.0 statistics software) were used for the determination of significant differences between different powders. The results were expressed as mean ± standard error (SE) and considered significantly different when p < 0.05.
Results and discussion
Surface tension of feed solution
shows the surface tension values of feed solutions with different Strawberry: MD: WPI (S:MD:WPI) ratios during spray drying. The ST value of the solutions with an S:MD:WPI ratio of 60:40:0 was 33.6 ± 0.4 mM m−1. The ST values of feed solution were significantly (p < 0.05) decreased with the WPI addition compared with solutions without WPI (S:MD:WPI, 60:40:0). The ST values of feed solution were significantly decreased with increasing WPI to 1%. However, no significant differences were observed in the ST values when the proportion of WPI increased from 1% to 10%. The results indicated that the addition of maltodextrin at any ratio showed no effect on the surface tension (33.2–33.8 mM m−1) of the strawberry puree. In contrast, WPI effectively reduced the ST of strawberry purees. WPI contains surface-active ingredients, which preferentially migrate to the air/water interface, and lower the ST of the solution. The results also indicated that about 1% of WPI might be saturated on the air/water interface. Other studies[Citation13] reported similar results of ST for honey following the addition of WPI.
The whey protein is mainly composed of β-lactoglobulin, α-lactalbumin, bovine serum albumin, and immunoglobulins, which have amphophilic structure and good emulsifying property. WPI contains surface-active ingredients, which preferentially migrate to the air/water interface, and lower the ST of the solution.[Citation8] However, sugars are not surface-active, the addition of MD at any ratio showed no influence on the ST of the feed solutions. About 1% of WPI might be saturated on the air/water interface because the ST remained almost constant when the WPI ratio was raised further. This implied that the droplet surface has already reached saturation and that no more protein molecules were able to occupy the surface even if the protein concentration in the bulk is increased. In fact, as low as 0.125% nominal protein composition in the feed appears to be sufficient to attain close to maximal protein coverage at the particle surface.[Citation8]
It has been suggested that a lower ST value of a solution indicates a higher surface activity of a solute in water.[Citation8] The ability of adding protein molecules to lower the ST value of solutions is attributed to their amphophilic properties, which cause them to diffuse and adsorb onto the newly created water/air or water/oil interfaces as rapidly as they formed.[Citation14] The adsorbed protein molecules partially unfold to expose a high proportion of their hydrophobic amino acid residues to the non-aqueous medium. The tendency of the protein molecules to partition between the aqueous and non-aqueous phases permits them to remain adsorbed on the interface, thus lowering interfacial tension.[Citation8,Citation15]
Powder recovery
The spray-dried strawberry powder was collected only from the product collection vessels, which were used to calculate powder recovery (Rp). Before spray drying solutions with different S:MD:WPI ratios, individual solutions of strawberry, MD, or WPI solutions containing the same feed concentration (11g/100g) were spray-dried for reference. However, all the strawberry solids were stuck onto the dryer wall and no powder was recovered from the collection vessel after spray drying of strawberry puree alone (). Thus, pure WPI and MD were only spray-dried as controls, and their Rp were 89 ± 2.1% and 86 ± 1.7%, respectively. Rp was significantly improved by the addition of MD or WPI into strawberry puree. When 30% and 50% of the strawberry TSS (total solid content) was replaced by MD, the Rp increased to 15 ± 1.1% and 42 ± 1.8%, respectively. However, the strawberry powder was difficult to collect when more than 50% of the strawberry TSS was replaced by MD. When WPI replaced 20% and 30% of the strawberry TSS, the Rp increased to 38 ± 2.5% and 48 ± 2.3%, respectively. In our preliminary experiments, the Rp was lower than 50%. The Rp was lower than 50% when our preliminary experiments were carried out to determine the suitable ratio of strawberry TSS to MD and WPI alone as a carrier. However, successful spray drying criteria should be higher than 50% proposed by Bhandari & Howes[Citation16] because Rp of lower than 50% was thought too low efficiency and high energy consumption.
When 40% of strawberry TSS was replaced by MD only, the Rp was 39.2 ± 2.3%. Interestingly, when 0.5% and 1% of MD were replaced by WPI, the Rp significantly increased to 45 ± 3.8% and 56.5 ± 2.8%, respectively (). When WPI ratio was increased from 1% to 40%, the Rp showed no significant differences with any of the samples (p > 0.05). At a WPI ratio of 1%, the Rp increased by 17.3%. The results were consistent with Muzaffar et al. [Citation17] who found that addition of SPI resulted in significant increase in powder recovery from 9.35% to 70.04%, thereby reducing the requirement of higher levels of maltodextrin for the production of quality tamarind pulp powder. However, increasing the WPI content higher did not have a significant effect on the yield. Different results were reported by Shi et al. [Citation13] who reported that increasing the honey TSS from 0.1% to 40% of MD and WPI, varied the Rp from 0% to 75.8% due to differences in sugar composition of the feed materials.
Figure 3. Effect of MD and WPI addition on the recovery of strawberry powder (with strawberry solid ratios in the feed solution fixed at 60%).
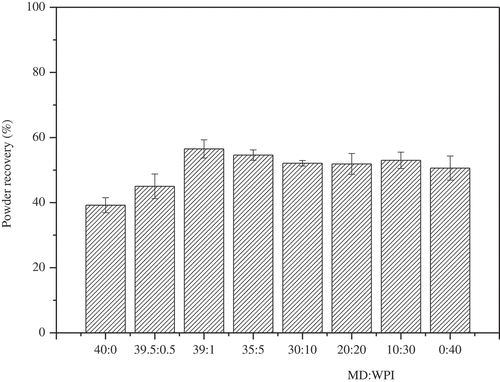
The decreased surface tension of WPI following the addition of strawberry puree may partly explain its higher powder recovery. Proteins preferentially migrate to the air-water interface of feed solutions and form a protein-rich film. When the film is subjected to hot and dry treatment, it is converted into a non-sticky glassy state upon drying. [Citation8] Hence, the enhanced powder recovery following the addition of 1% WPI in strawberry puree led to improved resistance to droplet coalescence as well as sticky interactions with the dryer wall.
Moisture content and water activity
The moisture content (MC) of the spray-dried strawberry powder are shown in . The MC ranged from 4.52 ± 0.06% to 4.92 ± 0.11%. The MC values of all the powder samples were also less than 5%. The water activity (aw) of the powders ranged from 0.208 ± 0.003 to 0.218 ± 0.006 suggesting that the spray-dried powders were relatively stable microbiologically. However, the package materials and storage conditions also play an important role in aw. Strawberry powder is highly hygroscopic and should be stored in air-tight containers in a cool dry place.
Table 1. Moisture content (MC), water activity (aw), bulk density (DB), and particle size (D[4,3]) of spray-dried strawberry powder.
Bulk density (DB) and particle size
DB and particle size were two important quality indicators in dried fruits and vegetable powders during processing, storage, packaging, and distribution. The DB of spray-dried strawberry powder was gradually decreased (p < 0.05) from 0.55 to 0.45 g mL−1 when the WPI total solid ratio in the feed solutions increased from 0% to 10%, and MD total solid ratio in the feed solutions decreased from 40% to 30%. Our results were similar to Muzaffar et al.[Citation17] involving spray drying of tamarind pulp using soya protein isolate and MD as wall materials. The DB decreased with increasing WPI ratios in the feed solutions, which might be related to surface shrinkage of strawberry powder by the SEM micrographs and would be discussed next. The DB of powders is influenced by the size, shape of powders and agglomeration. [Citation18]
The volumetric mean diameter D[3,4] of the spray-dried strawberry powder was significantly (p < 0.05) affected by the composition of feed solutions. Particle size decreased from 8.87 ± 0.01 to 7.12 ± 0.01 μm When the WPI total solid ratio from 0% to 10% and MD from 40% to 30% in the feed solution. The WPI total solid ratio in the feed solution increased from 0% to 10%, and the decrease in MD total solid ratio in the feed solutions from 40% to 30%, led to gradual decrease in powder particle size from 8.87 ± 0.01 to 7.12 ± 0.01 μm. The particle size of the spray-dried powder was affected by the total solid ratio of MD or WPI in feed solutions [Citation19], feed speed, and materials.
Glass transition temperature
Glass transition is the gradual and reversible transition in amorphous materials (or in amorphous regions within semicrystalline materials), from a hard and relatively brittle “glassy” state into a viscous or rubbery state as the temperature is increased.[Citation20] The Tg of spray-dried powder is an important indicator of the sticky behaviour of any sugar-rich foods. It is generally accepted that the droplet or particle temperature is 10–20°C above its Tg, especially for low molecular weight sugars and carbohydrates. [Citation21] The Tg of MD and WPI alone was 141.14 ºC and 128.6 °C, respectively, which is a little lower than that reported by Shi et al. [Citation13] . The slight discrepancy is possibly due to the variation with the water content.
The Tg of spray-dried strawberry powder was significantly (p < 0.05) affected by the composition of the ingredients. A decrease in MD total solid ratio in feed concentration from 40% to 30%, and an increase in WPI total solid ratio in feed concentration from 0% to 10%, led to a gradual increase in powder Tg from 32.60 ± 1.16°C to 38.39 ± 1.83°C (). Significant differences (p < 0.05) were observed between the powders produced with S:MD:WPI ratios of 60:40:0, 60:39.5:0.5, and 60:39:1. However, no significant differences (p > 0.05) were found between the powders with S:MD:WPI ratios of 60:39:1, 60:35:5, and 60:30:10. Fang and Bhandari[Citation22] reported that the Tg of WPI-bayberry juice powders showed no significant differences when WPI increased from 0.5% to 10%. However, the Tg of spray-dried honey:MD:WPI powders increased with a rise in MD total solid ratio in the feed concentration from 0% to 39.5%.[Citation13] The differences may be related to the composition of materials. Our results partly explain the higher powder recovery at S:MD:WPI ratios of 60:39:1, 60:35:5, and 60:30:10 compared with the ratios of 60:40:0, 60:39.5:0.5.
Table 2. Glass transition temperature of spray-dried strawberry powders.
Haque and Roos[Citation23] observed that the sugar-protein systems are not compatible and proteins increased the Tg of lactose in the anhydrous state in both spray-dried lactose/protein (5:1 and 3: 1) systems. Although the DSC method in this work is unable to determine the Tg of surface component, it was reasonable to deduce that the Tg of the powder surface (with large fraction of particles covered by protein) is much higher 20–40°C than their corresponding bulk Tg value[Citation8], which can keep a safe glassy state and resist heat stress during the drying process. Meanwhile, MD is not a surface-active material in solutions, and MD forms a compatible matrix at the molecular level with the strawberry solid materials to increase the overall Tg of the strawberry powders.
Surface morphology
SEM micrographs of spray-dried strawberry powder produced with different formulations of drying aids are illustrated in . The particles of spray-dried strawberry powder with an MD:WPI ratio of 40:0 were spherical with smooth surfaces ()). The surfaces of the bigger particles contained smaller particles, indicating particle agglomeration. As shown in -), the addition of WPI resulted in shrunken particle surface. The particles were not spherical, and even with the addition of 0.5% WPI, the particle morphology was altered. The surface shrinkage of strawberry powder increased with increase in WPI from 0.5% to 10%, which resulted in smaller particle size.
Figure 4. SEM micrographs of spray-dried strawberry powder produced with different formulations of drying aids: (a) 40% MD (b) 39.5% MD + 0.5% WPI (c) 39% MD + 1% WPI (d) 35% MD + 5% WPI (e) 30% MD + 10% WPI (at × 5000 magnification).
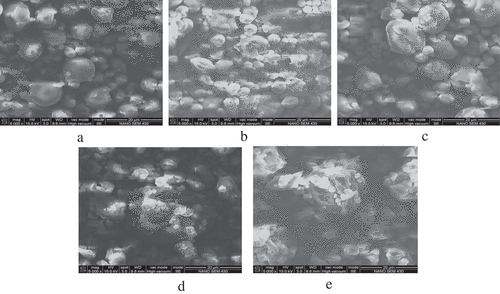
Studies have shown the surface of spray dried protein/carbohydrate powders to a large extent is covered by protein.[Citation8] For example, the surface of spray dried WPI/bayberry juice powders were covered by protein from 58.4% to 63.19% when WPI concentration was from 0.5% to 10%.[Citation22] Protein accumulated at the air/water interface is responsible for overcoming the stickiness of protein droplets during spray drying. The presence of surface indentations in the powders is attributed to uneven shrinkage during drying and/or cooling.[Citation24,Citation25]
Conclusion
Conversion of strawberry puree into powder form from spray drying is difficult because of the problems of stickiness and high hygroscopicity of a high proportion of low molecular weight sugars and organic acids in strawberry. Powder recovery was lower than 50% when MD and WPI alone replaced part of the strawberry TSS. Our results showed that Rp increased from 39.2 ± 2.3% (S:MD:WPI = 60:40:0) to 56.5 ± 2.8% when MD was replaced by WPI (S:MD:WPI = 60:39:1). The addition of WPI resulted in shrunken particle surface, which gave rise to smaller DB and particle size. The production efficiency of spray-drying strawberry powder was greatly improved when 1% MD was replaced by 1% WPI. This is attributed to amphophilic properties of WPI, which preferentially migrate to the air/water interface, form a protein-rich film, and decrease stickiness.
Acknowledgments
This work was supported by National Natural Science Foundation of China (31301531).
References
- Huntington, D. H.;. The Influence of The Spray Drying Process on Product Properties. Drying Technology 2004, 22(6), 1261–1287. DOI: 10.1081/DRT-120038730.
- Roos, Y.;. Melting and Glass Transitions of Low Molecular Weight Carbohydrates. Carbohydrate Research 1993, 238, 39–48. DOI: 10.1016/0008-6215(93)87004-C.
- Bhandari, B. R.; Datta, N.; Howes, T. Problems Associated with Spray Drying of Sugar-Rich Foods. Drying Technology 1997, 15(2), 671–684. DOI: 10.1080/07373939708917253.
- Bhandari, B. R.; Howes, T. Relating the Stickiness Property of Foods Undergoing Drying and Dried Products to Their Surface Energetics. Drying Technology 2005, 23(4), 781–797. DOI: 10.1081/DRT-200054194.
- Verdurmen, R. E. M.; Houwelingen, G. V.; Gunsing, M.; Verschueren, M.; Straatsma, J. Agglomeration in Spray Drying Installations (The EDECAD Project): Stickiness Measurements and Simulation Results. Drying Technology 2006, 24(6), 721–726. DOI: 10.1080/07373930600684973.
- Adhikari, B.; Howes, T.; Bhandari, B. R.; Troung, V. Effect of Addition of Maltodextrin on Drying Kinetics and Stickiness of Sugar and Acid-Rich Foods during Convective Drying: Experiments and Modelling. Journal of Food Engineering 2004, 62, 53–68. DOI: 10.1016/S0260-8774(03)00171-7.
- Bhandari, B. R. A.; Senoussi, E.; Dumoulin, D.; Lebert, A. Spray Drying of Concentrated Fruit Juice. Drying Technology 1993, 11, 1081–1092. DOI: 10.1080/07373939308916884.
- Adhikari, B.; Howes, T.; Bhandari, B. R.; Langrish, T. A. G. Effect of Addition of Proteins on the Production of Amorphous Sucrose Powder through Spray Drying. Journal of Food Engineering 2009, 94, 144–153. DOI: 10.1016/j.jfoodeng.2009.01.029.
- Fang, Z.; Wang, R.; Bhandari, B. Effect of Type and Concentration and Proteins on the Recovery of Spray-Dried Powder. Drying Technology 2013, 31, 1643–1652. DOI: 10.1080/07373937.2013.770011.
- Álvarez-Fernández, M. A.; Hornedo-Ortega, R.; Cerezo, A. B.; Troncoso, A. M.; García-Parrilla, M. C. Effects of the Strawberry (Fragaria Ananassa) Purée Elaboration Process on Non-Anthocyanin Phenolic Composition and Antioxidant Activity. Food Chemistry 2014, 164, 104–112. DOI: 10.1016/j.foodchem.2014.04.116.
- Oszmiajski, J.; Wojdyio, A. Comparative Study of Phenolic Content and Antioxidant Activity of Strawberry Puree, Clear, and Cloudy Juices. European Food Research Technology 2009, 228, 623–631. DOI: 10.1007/s00217-008-0971-2.
- Fang, Z.; Bhandari, B. Effect of Spray Drying and Storage on the Stability of Bayberry Polyphenols. Food Chemistry 2011, 129, 1139–1147. DOI: 10.1016/j.foodchem.2011.05.093.
- Shi, Q.; Fang, Z.; Bhandari, B. Effect of Addition of Whey Protein Isolate on Spray-Drying Behavior of Honey with Maltodextrin as a Carrier Material. Drying Technology 2013, 31, 1681–1692. DOI: 10.1080/07373937.2013.783593.
- Morr, C. V.; Ha, E. Y. W. Whey Protein Concentrates and Isolates: Processing and Functional Properties. Critical Reviews in Food Science and Nutrition 1993, 33, 431–476. DOI: 10.1080/10408399309527643.
- Fang, Z.; Wang, W. B.; Bhandari, B. Effects of Type and Concentration of Proteins on the Recovery of Spray-Dried Sucrose Powder. Drying Technology 2013, 31, 1643–1652. DOI: 10.1080/07373937.2013.770011.
- Bhandari, B. R.; Howes, T. Relating the Stickiness Property of Foods Undergoing Drying and Dried Products to Their Surface Energetics. Drying Technology 2005, 23(4), 781–797. DOI: 10.1081/DRT-200054194.
- Muzaffar, K.; Kumar, P. Effect of Soya Protein Isolate as a Complementary Drying Aid of Maltodextrin on Spray Drying of Tamarind Pulp. Drying Technology 2016, 1, 142–148. DOI: 10.1080/07373937.2015.1042586.
- Širola, I.; Lukšić, J.; Simunić, B.; Kujundžić, N. Effect of Crystal Size and Shape on Bulk Density of Pharmaceutical Powders. Journal of Crystal Growth 1997, 181(4), 403–409. DOI: 10.1016/S0022-0248(97)00299-6.
- Wu, W.; Liu, W.; Gengenbach, T.; Woo, M. W.; Selomulya, C.; Chen, X. D.; Weeks, M. Towards Spray Drying of High Solids Dairy Liquid: Effects of Feed Solid Content on Particle Structure and Functionality. Journal of Food Engineering 2014, 123, 130–135. DOI: 10.1016/j.jfoodeng.2013.05.013.
- ISO11357-7: Plastics – Differential scanning calorimetry – Part 2: Determination of glass transition temperature 2015.
- Bhandari, B. R.; Senoussi, A.; Dumoulin, E. D.; Lebert, A. Spray Drying of Concentrated Fruit Juice. Drying Technology 2007, 11(5), 1081–1092. DOI: 10.1080/07373939308916884.
- Fang, Z.; Bhandari, B. Comparing the Efficiency of Protein and Maltodextrin on Spray Drying of Bayberry Juice. Food Research International 2012, 48, 478–483. DOI: 10.1016/j.foodres.2012.05.025.
- Haque, M. K.; Roos, Y. H. Differences in the Physical State and Thermal Behaviour of Spray-Dried and Freeze-Dried Lactose and Lactose/Protein Mixtures. Innovative Food Science and Emerging Technologies 2006, 7(1–2), 62–73. DOI: 10.1016/j.ifset.2004.12.004.
- Hogan, S. A.; Mcnamee, B. F.; O’Riordan, E. D.; O’Sullivan, M. Emulsification Properties of Sodium Caseinate/Carbohydrate Blends. Journal of Agriculture and Food Chemistry 2001, 49, 1934–1938. DOI: 10.1021/jf000276q.
- Wang, S.; Langrish, T. The Use of Surface Active Compounds as Additives in Spray Drying. Drying Technology 2010, 28, 341–348. DOI: 10.1080/07373931003641404.