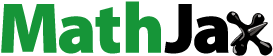
ABSTRACT
Almond fruits are subjected to various mechanical stresses throughout production, from harvest to processing, storage and packaging. Kernel properties play an important role in reducing mechanical damage such as scratches and penetration of shell pieces. Knowledge of kernel properties under various conditions of the fruit can assist in optimising post-harvest and processing lines to minimise kernel damage and thus maximise final kernel quality. Kernel moisture content is one of the main attributes affecting the kernel’s response to mechanical processing. Increasing the kernel moisture content to an optimum level through wetting fruit prior to processing can lead to a reduced percentage of damaged kernel. Water added to the structure of kernels acted as a plasticiser and helped the kernels to absorb the mechanical load instead of fracturing and breaking into pieces. In this study, tests were conducted on almond kernels with different moisture content levels from 5.52 to 14.09g/100g wet basis. Kernels from a Nonpareil variety were tested in dried and wetted conditions. Test results showed that kernels with higher moisture content were able to undergo a larger deformation at a given force value in comparison with dry kernels. Average deformation for dry samples was from 0.12 mm, which increased to an average of 0.25 mm in wetted samples. The effect of skin on the mechanical properties of the kernels (with and without skin) was studied using a mechanical tester. The test results showed a peak force value in samples tested with skin in comparison with the kernels tested without skin.
Introduction
Almonds are harvested dry with a kernel moisture content of less than 7 g/100g wet basis Micke.[Citation1] The in-hull fruit is then piled on the farm until it is sent to processing plants for cleaning, hulling and shelling. Almond processing consists of various loading stages where the fruit undergoes compression, shear and impact in order to hull and shell the fruit and release the kernel. Commercial hulling and shelling operations are done on in-hull fruit using shear rollers and/or rolls over belts Micke[Citation1] to break the hull and shell layers and release the kernel. There are also recommendations for using impact threshing systems in removing hull and shell specifically for harder shell varieties of almonds Shirmohammadi and Fielke.[Citation2] The current commercial systems release the kernel through several loading stages and usually create a high percentage of kernel damage including broken, chipped and scratched product. In order to define why and where the damage occurs during processing, it is essential to develop a detailed knowledge of kernel physical and mechanical properties. The properties mentioned also define the sensory and textural characteristics of kernels, which are very important aspects of nut quality and standard Saeleaw and Schleining.[Citation3]
The moisture content of fruit at harvest time and prior to handling and processing plays an important role in the mechanical response of the product under loading. For managing the concealed damage during the pre-processing stage, Halbrook, et al.[Citation4] lower fruit moisture content is desired, however, this can cause brittleness and lead to higher mechanical damage. Rainfalls during the harvest season can also affect the harvest outputs by increasing the moisture level of fruit and postpone the harvest operations Gradziel.[Citation5] To create an optimal post-harvest and processing quality it is crucial to maintain the level of moisture content according to the kernel mechanical properties to diminish any mechanical damage that can occur during hulling, shelling and handling.
Numerous studies have demonstrated that there is a strong correlation between moisture content and mechanical properties of nuts and grains, which is important for determining the optimum moisture content for processing and product quality Aydin,[Citation6] Davidson Jr, et al.,[Citation7] Ekinci, et al.,[Citation8] Shahbazi,[Citation9] Shirmohammadi and Fielke,[Citation2] Sims,[Citation10] WoŸniak,[Citation11] Woźniak and Styk.[Citation12] For example, studies on peanuts have shown that under impact loading, increased moisture content reduces the percentage of damaged kernels Davidson Jr, et al.[Citation7] Cracking tests conducted on apricot seeds also showed that a higher percentage of kernels are damaged when they are processed dry and determined an optimum level of moisture content for cracking Shahbazi.[Citation9] The mechanical response of sunflower seeds to loading has also shown that the moisture content plays an important role in the mechanical properties Gupta and Das.[Citation13] Studies on seeds have also demonstrated that increases in moisture content of rapeseed, wheat, and beans lead to decreased damage during impact Shahbazi, et al.,[Citation14] Szwed, and Lukaszuk.[Citation15]
In cases where the brittleness of the kernel has increased damage during post-harvest and processing, water conditioning has been used on nuts and grains to reduce kernel mechanical damage Aydin,[Citation6] Davidson Jr, et al.,[Citation7] Shahbazi,[Citation9] Shirmohammadi and Fielke,[Citation2] Sims,[Citation10] WoŸniak,[Citation11] Woźniak and Styk.[Citation12] The addition of water to the tissue structure increases the elastic properties of the kernel and reduces the possibility of mechanical damage due to loading Szwed and Lukaszuk.[Citation15] Previous studies on impact threshing of soft and hard shell almonds showed that pre-wetting and processing can reduce kernel damage during hulling and shelling Shirmohammadi and Fielke.[Citation2]
Wetting can be an option to enhance the processing effectiveness of almond kernels: water added could act as a plasticiser in kernel and skin structure, leading to an increase in kernel meat and skin resistance to mechanical damage Shirmohammadi and Fielke.[Citation2] However, while the percentage of broken kernels is reduced after wetting treatment, the percentage of scratches on the kernel skin is still high Shirmohammadi and Fielke.[Citation2] Whilst this study focused on shell characteristics after wetting treatment [2], further work is needed to address the properties of skin and kernel in reducing the percentage of scratched kernels after the mechanical hulling and shelling process. Therefore, the results presented in this paper aim to investigate how added water can help to increase the resistance of kernel and skin to damage under mechanical loads during harvest and post-harvest operations, specifically focusing on skin rupture and scratched kernel percentage.
Methods
Geometric properties
Samples of raw Nonpareil almond kernels harvested in the 2016–2017 harvest season were used in this study. To capture the effects of skin on mechanical properties, kernels were tested with and without skin. The skin was removed using a sharp cutter prior to testing, and the average size of the samples was recorded by measuring the length (L), width (W), thickness (T), and mass of a minimum of 20 kernels prior to testing. The calculated properties including arithmetic diameter (mm), geometric diameter (mm) and sphericity (%) were calculated using Equations 1–3 Ludger Figura:[Citation16]
Colour readings
An UltraScan VIS Spectrophotometer (Hunterlab) using an integrating sphere and an illuminant A/2 was used to record the colour of kernels. This device measures the colour specifications of food particles that most closely matches the way that the human eye sees the colour. Values of L*, a* and b* were recorded for each sample. The L* value indicates the light and dark spectrum with a range of 0 to 100 for black and whiteness. The a* value shows the green to red spectrum with a range of −60 (green) to 60 (red). The b* value shows the blue to yellow colours for −60 (blue) to 60 (yellow) values. Values of a*, b* and L* were determined using the Hue angle and Chroma values according to Equations 4 and 5:
Sample hydration
To investigate the effects of moisture content on mechanical properties, kernel samples were soaked in water (15–20 ⁰C) for different periods of time. The samples were prepared in two categories of dry and wetted (soaked 10, 20 and 30 min), and the details of moisture content changes are presented in . The wet basis moisture content of samples was determined gravimetrically by placing samples in an oven at 103 ± 3⁰C for 72 h and weighing the samples before and after drying.
Table 1. Changes in moisture content of almond kernel sample with skin tested.
To study the effects of resting after soaking and changes in kernel texture, samples that had been soaked in water for 10 min were subsequently allowed to rest for 0, 1, and 2 h before mechanical testing. During resting, samples were kept in sealed plastic containers at standard laboratory conditions in order to stop loss of moisture.
Skin thickness
To determine the thickness of the skin and the effect of wetting on the skin’s thickness and structure, environmental scanning electron microscopy (ESEM) was performed using an FEI Quanta 450 FEG ESEM. Samples of dry and wetted kernels were cut in half and placed on the ESEM platform for imaging. The relative humidity (RH) of the ESEM chamber was set to 43–47% (low RH) or 100% (high RH) during the imaging at a constant temperature of 2°C. To prevent the reduction of moisture content during imaging, the images of wetted samples were taken at 100% RH, while the imaging for dry samples was conducted at low RH.
Mechanical testing
Macroscale compression test
To study the effects of skin on the mechanical properties of the kernels, indentation tests were performed using a Shimadzu EX-LZ with a 50 N load cell. Samples were positioned on the tester’s stage on their flat side. The testing was conducted at a loading speed of 1 mm/s using a sharp end cone indenter with 6 mm diameter and 4 mm height. Each test was stopped when the compressive load reached 50 N, while results showed the response of kernel skin under load, the kernel itself did not reach the fracture point.
The recorded force-displacement curves were used to determine the yield point, stiffness (N/mm), toughness (N.mm) and the slope of the force versus deformation curve. The stiffness and toughness were calculated according to Equations 6 and 7 Aguilera and Lillford:[Citation17]
Mechanical testing was conducted on dry and wetted kernel samples with and without skin. Wetted samples were prepared as described above and tested immediately after soaking. The samples that were soaked for 10 min were also tested after resting for 1 and 2 h in order to determine the time-dependent effects of water absorbed on the kernel mechanical properties.
Nanoindentation of the almond kernel
The mechanical properties of the almond kernels were determined via nanoindentation using a UMIS2000 system (CSIRO, Australia). Due to the irregularities and large roughness of both the skin and the outer part of the almond, tests were only performed on peeled and cut samples (10 mm length from the central part of the kernel) to exhibit a flat and smooth surface. Samples were then placed on the indentation sample holder using super glue. A Berkovich diamond indenter (Semelab, Ltd.) was used for testing at a loading speed of 0.23 mN/s. The kernel samples were loaded in the direction perpendicular to the cross section, and the load applied varied between 2 and 10 mN. To assess the creep and viscoelasticity of the almond sample, the maximum load applied was held 20 s at 10 mN before unloading ().
Figure 1. Typical force versus displacement curve during nanoindentation for loading and unloading, are the plastic deformation, elastic deformation, and maximum height of indentation, respectively.
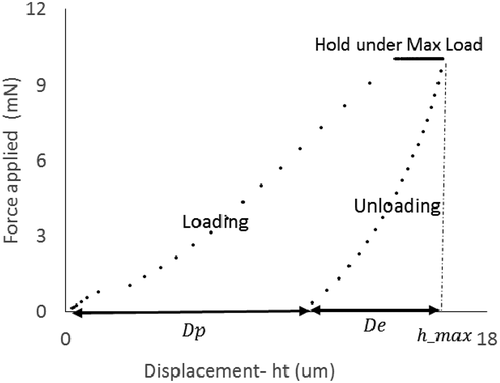
Each test was repeated 8 times and the average force values, deformation depth and energy absorbed were determined. A typical experimental curve is shown in and , loading and unloading details were determined by releasing the load where some elastic deformation recovered. The difference between the loading and unloading curve defined the permanent deformation of the material by defining the depth of penetration (defined as in ). Noting that testing a totally elastic material presents exactly the same loading and unloading curves. The slope of the unloading curve allows the contact stiffness (S) to be determined Duprat, et al.,[Citation18] Hernández-Sánchez and Gutiérrez-López.[Citation19]
Figure 2. Typical force versus deformation curve at different moisture content on raw almond kernel tested (a) with skin and (b) without skin.
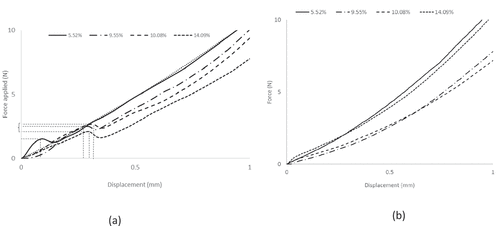
The hardness (H) and elastic modulus (E) values were calculated for each sample. Values of elasticity and degree of elasticity were determined using the following equations Considine:[Citation20]
Results and discussion
Physical analysis of the dry kernels (with skin) with a moisture content of 5.52 g/100g wet basis provided average arithmetic diameter, geometric diameter, mass, and sphericity values of 14.7 mm, 13.7 mm, 1.31 g, and 38.5%, respectively (). The sphericity values determined for the Nonpareil variety were lower than that reported previously for hard-shell varieties Arslan and Vursavus,[Citation21] Aydin.[Citation6] Colour measurements revealed that the Nonpareil variety (54.24) was lighter in colour in comparison with the hard-shell varieties Ferragnes (39.03), Ferradual (35.28) and Guara (38.71) reported in literature Arslan and Vursavus.[Citation21]
Table 2. Size specification and colour measurement of samples used for testing.
Compression testing
Mechanical compression tests were performed using a cone-shaped probe on dry and wetted kernels with and without skin in order to study the effects of skin on damage resistance of kernels. The force versus displacement curves obtained for kernels with skin layer at each moisture content level provided peak forces (yielding points) at average loads of 1.64, 1.88, 1.89, and 1.91 N for kernels with moisture contents of 5.52, 9.55, 10.08, and 14.09 g/100g wet basis, respectively. This yielding point can be related to the skin rupture prior to the kernel rupture that happens at much higher force values. The deformation at this yielding point increased from 0.12 mm to ~0.25 mm for dry and wetted samples, respectively. The values of skin rupture force and the deformation at those points can be used in defining the threshold load values to reduce the damage due to rupturing skin. In setting up the hulling and shelling equipment the rupture force values can be applied to reduce the possibility of scratches on kernels during processing (). These data are essential in determining the optimum moisture content input of fruit for re-designing post-harvest technologies and operations Shirmohammadi and Fielke.[Citation2]
Table 3. Degree of elasticity and residual deformation for wet and dry samples after soaking in water (samples were tested right after removed from water).
Although the existing data on the properties of the kernel are limited, previous studies on the mechanical properties of in-shell almond samples showed that the mechanical properties are a function of moisture content of the fruit Aydin.[Citation6] Furthermore, mechanical testing of Nonpareil almond kernels after 10 min soaking in water using a platen to compress the samples also reported a kernel rupture forces increase from 27.5 N at 4.6% moisture content to 52.7 N at 7.7% moisture content Shirmohammadi and Fielke,[Citation2] which was accompanied by a decrease in the stiffness values.
The changes observed in force and deformation values for dry and wetted samples with skin are presented in . The deformation values at similar force ranges were also determined for samples tested without skin (), which showed a similar increase in values from dry and wetted samples. The results indicate that kernels with higher moisture levels have improved mechanical properties that would help to reduce the brittleness of the kernels under load. The peak force requires to rupture the skin varied from 1.6 N to 1.9 N as moisture content increased. This would be expected to lead to reduced mechanical damage during processing of nuts. However, as previously shown, there is an optimum moisture content level (10, 12, 10, and 14 g/100g wet basis for Nonpareil, Vyro, Tarraco, and Marcona, respectively) in order to produce maximum undamaged kernel [2].
Figure 3. (a) Force and deformation values for kernels at yielding point, and stiffness and toughness values for samples tested (b) with and (c) without skin.
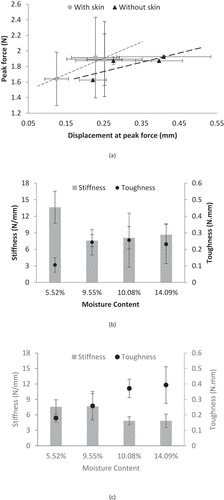
A clear difference in the mechanical properties of the kernels was observed when the moisture content increased. The stiffness values reduced from 13.63 N/mm at 5.52 g/100g wet basis moisture content to 8.65 N/mm at 14.01 g/100g wet basis moisture content, and toughness values increased from 0.11 N.mm to 0.23 N.mm for dry and wetted samples. The ANOVA results on effects of skin and moisture content showed significant differences in values of stiffness and toughness (). ANOVA results indicated the importance of optimum moisture content of kernel in order to minimise the kernel mechanical damage due to brittle behaviour. Studies on roasting and reducing the moisture content of kernels have also shown that the brittleness increases as kernels lose moisture due to heat treatment Kader, et al.,[Citation22] Varela, et al.[Citation23] The stiffness values for the kernels with the skin at the peak force where the skin was ruptured () displayed a similar decreasing trend from 293 to 67.2 N/mm for raw and 99.3 to 49.7 N/mm for roasted almonds after soaking in water Kong and Singh.[Citation24]
The stiffness values determined as the ratio of force over deformation at the yield point are presented in . The slope of the force-deformation curve decreased as moisture content increased. The toughness values showed that greater energy is required to create the damage on kernels when the moisture content is higher. Similar trends were observed when shelled corn kernels at different moisture content were mechanically loaded Shelef and Mohsenin.[Citation25] To investigate the effects of absorbed water on the kernel mechanical properties (with skin) following wetting and then resting, kernels were soaked for 10 min and then rested at 20–25°C in a sealed container for 1 or 2 h. The peak force and deformation at the yielding point were found to increase after wetting and resting (), indicating that on resting, the absorbed water has time to permeate through the kernel structure. Determination of the stiffness and toughness values revealed that after soaking and resting, there was a significant increase in toughness and a slight decrease in stiffness ().
Figure 4. Force versus displacement for samples soaked for 10 min and then rested for various times.
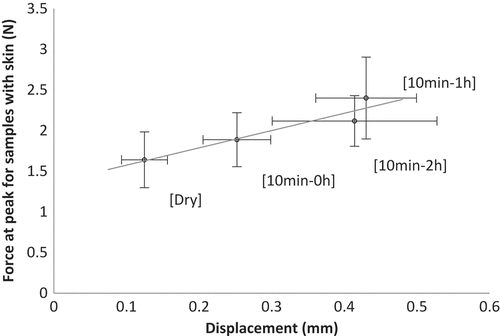
Figure 5. Stiffness and toughness values determined for soaked and rested samples, the x-axis shows the soaking time (in min)-the resting time (in hour).
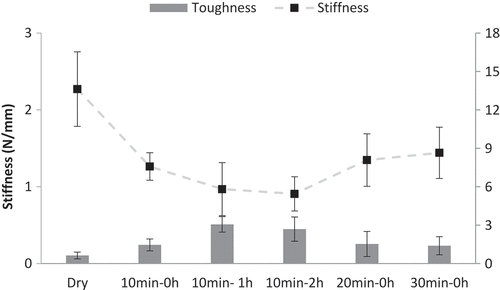
The resting time (the time between when samples are removed from soaking in water until testing time) can allow for the use of less water for wetting and longer time for the kernels to absorb the moisture. The moisture increase also changes with the variation of relative humidity (RH) of the environment, which needs to be investigated in the future. This can mean that the hot and humid harvest seasons and rainfalls can actually lead to an increase in fruit moisture content to optimum levels and reduce mechanical damage due to the brittle behaviour of the kernel.
Our previous studies on the mechanical damage on almond kernels during shelling and hulling indicate the advantages of wetting fruit prior to processing Shirmohammadi and Fielke.[Citation2] Determining the optimum conditions for the wetting process, including the soaking and resting time, is required in order to minimise the use of water and time required for the treatment and provide benefits in mechanical properties. The test results showed that resting is also important in changing the mechanical properties of the kernels and reducing potential damage to the kernels. These results could also be applied to determine the best re-shaking time during a wet harvest season. The current commercial harvest operation leaves the wet fruit after re-shaking on the ground to dry while the additional moisture can lead to microbial growth Uesugi and Harris.[Citation26] The results of this study indicate that processing should be conducted when the kernel moisture content is at an optimum level to prevent kernel damage. The results of this study indicate that the wetter product (moisture content higher than 7%) can be processed if the processing equipment is set for wet fruit, taking advantage of the plasticity water adds to the kernel and skin structure. Previous studies also showed that impact threshing can be applied to wetted almond in-hull fruit with high kernel moisture content and still create a high percentage of undamaged kernel recovery of 91%, 75%, and 67% for Nonpareil, Marcona and Vyro varieties, respectively.
Study of changes in skin thickness
To study the effects of moisture on the structure of the kernel and skin, ESEM imaging was conducted on dry and wetted samples. The images were taken from different sections of one sample in order to investigate the layers and thickness of the skin. For a dry sample, there was a complex skin structure consisting of two main sections with an overall thickness of approximately 50 um (51.9 ± 3.1) ( and ). Whereas the outer (top) section consisted of a layered structure parallel to the surface of the nut, the inner (bottom) section consisted of a cell-like structure with walls organised roughly perpendicular to the surface.
The images of the kernel with skin taken after different wetting periods (analysis conducted at RH of 100% at 2 ⁰C) show that the two sections (top and bottom layers of skin) become thicker as moisture content increased (). The water added to the skin could act as a support in absorbing the mechanical damage and reducing the possibility of cell damage in comparison with the brittle condition of the dry kernel.
Nano-mechanical properties of kernels
Nanoindentation was performed on dry and wet almond kernels (after removing the skin and cutting the kernel) by loading the samples at a rate of 0.23 mN/s, holding the load and then unloading. Representative force-deformation results for nanoindentation tests on samples with three different moisture contents are presented in . The force-depth curves from the nanoindentation tests indicate that wetting the kernel increases compliance (lowers the elastic modulus). Based on the decrease in hardness, water added to the kernel samples increased the ductility of wet samples in comparison with dry samples, making them less likely to exhibit brittle fracture. Also, adding water increased the viscoelastic behaviour of the almond, which enables a larger non-plastic energy dissipation, as observed by the large relaxation creep plateau under maximum load. The energy dissipated due to loading and unloading tests shows the sensitivity of kernel structure to loading and suggests some type of failure occurs Figueroa, et al.[Citation27]
Figure 8. Deformation versus the moisture content obtained for kernels with 14.09% (wet), 9.55% (wet) and 5.52% (dry) moisture contents. The deformation was determined as the depth of penetration () to the samples.
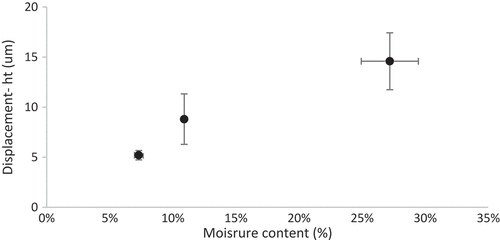
The changes due to added water to the structure increase kernel resistance to the mechanical damages during processing stages. The data indicate the desirable effect that increasing moisture content has on reducing the damage to the kernel product, which could be applied during mechanical operations that fruit undergo after harvest. This supports our previous work on impact hulling and shelling of almonds, which showed that wetting of fruit results in lower kernel damage Shirmohammadi and Fielke.[Citation2] Determination of the degree of elasticity () – as defined by the ratio of recoverable deformation to total deformation – revealed that wetted kernels had a higher degree of elasticity, and the degree of plasticity decreased as moisture content increased.
Conclusion
Damaged kernel (broken and chipped) and scratched kernel skin (missed parts of skin) are some undesirable changes on final kernel product considered as the quality loss by the industry. In this study, the mechanical properties of almond kernels were investigated at different moisture levels. To study the effects of skin on mechanical damages such as scratches, samples were tested with and without skin. The results indicated that an increase in magnitude of force required to break the skin layer from 1.64 N to 1.91 N when samples were tested dry (5.52 g/100g wet basis moisture content) and wetted (14.09 g/100g wet basis moisture content), respectively. The deformation at the skin breakage point increased as moisture content increased from 0.12 mm to 0.25 mm. The tests results showed higher toughness values for both samples tests with and without skin. The results of force and deformation values for kernel products showed that an optimum moisture level could be determined before processing of fruit. Adding moisture could be applied if the kernel initial moisture content is low in order to reduce the damages due to brittle characteristics of the kernel. This knowledge can be used in setting the equipment to create the lowest damage to the kernel. Resting wetted samples showed that longer times increase both values of force and displacement for skin breakage from 0.12 mm and 1.64 N when samples were dry to 0.41 mm and 2.12 N when samples were soaked for 10 min and rested for 2 h. The increase in deformation from 0.25 mm to 0.41 mm caused by resting samples was also considerable, which indicates absorption of moisture into the kernel structure can decrease the chances of kernels being damaged during mechanical operations such as hulling and shelling. Further testing of the nanoproperties of kernels also showed that the degree of elasticity increases from 43% to 61% as the kernel moisture content increased from 5.52 to 14.09 g/100g wet basis. The nanoindentation loading and unloading curves also showed a difference between how kernel samples respond to loading and unloading tests. These results indicate the importance of determining properties of fruit prior to the harvest and processing which could lead to better harvest timing and conditioning of fruit with emphasis on reduction of mechanical damage on kernel products.
Acknowledgments
This study was not funded by any company or organisation. Conflict of Interest: There is no conflict of interest. No animal samples were used in this study. No human was involved in this study. The study was conducted on nut kernel purely experimental and theoretical and no individual participant was involved in the study.
References
- Micke, W. Almond Production Manual; UCANR Publications: Oakland, California, 1996, Vol. 3364.
- Shirmohammadi, M.; Fielke, J. Conditioning Reduces Kernel Damage When Impact Shelling Almonds. International Journal of Food Engineering 2017, 13(8), 1556–3758.
- Saeleaw, M.; Schleining, G. A Review: Crispness in Dry Foods and Quality Measurements Based on Acoustic–Mechanical Destructive Techniques. Journal of Food Engineering 2011, 105(3), 387–399.
- Halbrook, W.; Fuller, G.; Whitehand, L. Almond Nutmeat Moisture and Water Activity and Its Influence on Fungal Flora and Seed Composition. Journal of Food Science 1983, 48(2), 615–617.
- Gradziel, T.M. Almonds: Botany, Production and Uses; CABI: Oxfordshire, 2017; pp 1–494.
- Aydin, C. Physical Properties of Almond Nut and Kernel. Journal of Food Engineering 2003, 60(3), 315–320.
- Davidson, J.I., Jr; Whitaker, T.B.; Dickens, J.W. Grading, Cleaning, Storage, Shelling, and Marketing of Peanuts in the United States. Peanut Science and Technology 1982, 571–623.
- Ekinci, K.; Yılmaz, D.; Ertekin, C. Effects of Moisture Content and Compression Positions on Mechanical Properties of Carob Pod (Ceratonia Siliqua L.). African Journal of Agricultural Research 2010, 5(10), 1015–1021.
- Shahbazi, F. Effects of Moisture Content, Impact Direction and Impact Energy on the Cracking Characteristics of Apricot Pit. World Applied Sciences Journal 2012, 20(11), 1520–1528.
- Sims, K.A. Mechanization of Post-Harvest Pecan Processing. In Charls R. Santerre, editor, Pecan Technology; Springer, 1994; pp 68–86.
- Woÿniak, W. Mechanical Properties of Wheat Grain in Relation to Internal Cracks. International Agrophysics 2001, 15, 59–64.
- Woźniak, W.; Styk, W. Internal Damage to Wheat Grain as a Result of Wetting and Drying. Drying Technology 1996, 14(2), 349–365.
- Gupta, R.; Das, S. Fracture Resistance of Sunflower Seed and Kernel to Compressive Loading. Journal of Food Engineering 2000, 46(1), 1–8.
- Shahbazi, F.; Analooei, M.; Saffar, A. Mechanical Damage to Pinto Bean Seeds as Affected by Moisture Content, Impact Velocity and Seed Orientation. International Journal of Food Engineering 2011, 7(6), 1556–3758.
- Szwed, G.; Lukaszuk, J. Effect of Rapeseed and Wheat Kernel Moisture on Impact Damage. International Agrophysics 2007, 21(3), 299–304.
- Ludger Figura, A.A.T. Food Physics: Physical Properties - Measurement and Applications; Springer Berlin Heidelberg: Leipzig, Germany, 2007.
- Aguilera, J.M.; Lillford, P.J. Food Materials Science: Principles and Practice; Springer Science & Business Media: Santiago, Chile, 2007.
- Duprat, F.; Grotte, M.-G.; Pietri, E.; Studman, C. A Multi-Purpose Firmness Tester for Fruits and Vegetables. Computers and Electronics in Agriculture 1995, 12(3), 211–223.
- Hernández-Sánchez, H.; Gutiérrez-López, G.F. Food Nanoscience and Nanotechnology; Springer: Mexico City, Mexico, 2015.
- Considine, D.M. Foods and Food Production Encyclopedia; Springer Science & Business Media: New York, 2012.
- Arslan, S.; Vursavus, K.K. Physico-Mechanical Properties of Almond Nut and Its Kernel as a Function of Variety and Moisture Content. The Philippine Agricultural Scientist 2008, 91(2), 171–179.
- Kader, A.A.; Heintz, C.; Labavitch, J.; Rae, H. Studies Related to the Description and Evaluation of Pistachio Nut Quality. Journal Amer Social Hort Sciences 1982, 107(5), 812–816.
- Varela, P.; Chen, J.; Fiszman, S.; Povey, M. Crispness Assessment of Roasted Almonds by an Integrated Approach to Texture Description: Texture, Acoustics, Sensory and Structure. Journal of Chemometrics 2006, 20(6‐7), 311–320.
- Kong, F.; Singh, R.P. Digestion of Raw and Roasted Almonds in Simulated Gastric Environment. Food Biophysics 2009, 4(4), 365–377.
- Shelef, L.; Mohsenin, N.N. Effect of Moisture Content on Mechanical Properties of Shelled Corn. Cereal Chemistry 1969, 46(3), 242–253.
- Uesugi, A.R.; Harris, L.J. Growth of Salmonella Enteritidis Phage Type 30 in Almond Hull and Shell Slurries and Survival in Drying Almond Hulls. Journal of Food Protection 2006, 69(4), 712–718.
- Figueroa, J.; Hernández, Z.; Véles, M.; Rayas-Duarte, P.; Martínez-Flores, H.; Ponce-García, N. Evaluation of Degree of Elasticity and Other Mechanical Properties of Wheat Kernels. Cereal Chemistry 2011, 88(1), 12–18.