ABSTRACT
The current study was aimed to investigate the effect of glucosinolate degradation on the flavor precursors and pungent odors in raw and microwaved rapeseeds. Five isothiocyanates and six sulfur compounds were identified using comprehensive two-dimensional gas chromatography and time-of-flight mass spectrometry (GC× GC-TOF/MS). Comprehensive analysis with headspace solid-phase microextraction (HS-SPME) and aroma extract dilution analysis (AEDA) with altering split ratio showed that 1-isothiocyanato butane, allyl isothiocyanate and 4-isothiocyanato-1-butene were responsible for the sulfur, pungent and green odors in rapeseed oils, due to their high flavor dilution factor. Dimethyl sulfide, dimethyl trisulfide and dimethyl sulfoxide, might be derived from methionine or isothiocyanate rearrangement reactions imparted onion-like, cabbage-like, garlic-like, and sulfurous-pungent odors. Rapeseed samples were heated in a microwave for seven minutes, and the total glucosinolate content decreased from 28.2 ± 0.8 μmol/g to 14.5 ± 0.6 μmol/g in Brassica napus, from 101.2±3.0 μmol/g to 42.2 ± 5.4 μmol/g in Brassica campestris, and from 132.02 ± 9.31 μmol/g to 52.50 ± 6.50 μmol/g in Brassica juncea, compared to untreated ones. Differences in the concentrations of six main odorants and the glucosinolate precursors from genetic types were responsible for the intensity of the pungent odors in raw and microwaved rapeseeds. The result can assist to determine the flavor of the commercial rapeseed oils.
Introduction
Consumer acceptance of commercial rapeseed oils is often determined by the characteristic flavors and the nutrient values of the samples.[Citation1] The flavoring ingredients in the rapeseed oils are mainly generated via fatty acid degradation, Maillard or amino acid degradation and/or secondary metabolites-glucosinolate degradation.[Citation2]
Glucosinolates (GLS) are sulfur- and/or nitrogenous secondary metabolites, primarily present in the plants of Brassicaceae family.[Citation3] The pungent flavor in rapeseed oils is usually imparted by glucosinolates.[Citation4] Chemically, glucosinolates are classified as aliphatics, indoles, and aromatic glucopyranoses and oxime derivatives of amino acids (e.g., methionine, phenylalanine, and tryptophan).[Citation5] Distribution and content of glucosinolates largely depend on the genotypes of rapeseed plants. Glucosinolates may biotransform into isothiocyanates, thiocyanates, nitriles, and oxazolidine; of Myrosinase generates allyl isothiocyanate from glucosinolates, which was responsible for the pungent and sulfurous aroma of the rapeseed oils.[Citation6,Citation7] In addition, dimethyl sulfide, dimethyl disulfide, dimethyl trisulfide, and methanethiol were determined in cabbage, which contributed to onion, cabbage, and sulfur pungent odors.[Citation8] In China market, traditional rapeseed was usually contain pungent and irritating smell. The molecule source of the odor, however, is not clear enough in rapeseed oil.
Headspace solid phase microextraction (HS-SPME) coupled with gas chromatography-mass spectrometry (GC-MS) is the most popular method for analyzing the volatile aromas in rapeseed oils. Rapeseed oil contains many types of characteristic odors. Seven different isothiocyanates and sulfur compounds were discovered in the commercial rapeseed oils, and four of them were identified as 3-butenyl-, 4-pentenyl-, phenethyl isothiocyanate, and 5-vinyl-2-oxazolidinethione.[Citation9] Jeleń et al. evaluated the effects of storage time and temperature on the content and distribution of volatile compounds in the refined and cold-pressed rapeseed oils both at ambient and elevated temperature.[Citation10,Citation11]
Flavors are usually analyzed by sensory, instrumental, or a combination of both techniques. Utilizing the aroma extract dilution analysis (AEDA), Guth and Grosch evaluated the contribution of each aroma compound for developing the final odor in refined rapeseed oil and detected the following compounds, namely (Z)-1,5-octadien-3-one, 1-octene-3-one, (Z)-2-nonenal, (E)-2-nonenal, 3-methyl- 2,4-nonandione, and trans-4,5-epoxy-(E)-2-decenal with high FD factors (≥16).[Citation12] Matheis et al. detected 53 aroma compounds with FD factors ranging from 8 to 2048 in rapeseed oil, where dimethyl sulfide showed the highest FD factor of 8 among asparagus-like flavors, and methyl propanal (malty odor) had the highest FD factor of 4 for the oils with fusty/musty off-flavor.[Citation13] Then, the same group identified dimethyl trisulfide, dimethyl sulfide, pyrazines and aldehydes in a local commercial cold-pressed rapeseed oil from unpeeled seeds by solvent-assisted extraction and aroma extract dilution analysis, and characterized them using the sensory omics approach.[Citation14] In another study, a distillate of roasted (200°C, 10 min) white mustard seed was analyzed by a similar method.[Citation15] Dimethyl trisulfide was found to impart a cabbage-like flavor to roasted Brassica seeds. Raw or roasted from mustard seeds and rapeseeds were analyzed by static headspace and aroma dilution analysis. In their result, the dilution factors of the compounds showed different values depend on extraction and dilution methodology.
Microwave-assisted heating method is employed for improving the comprehensive qualities, like the oil extraction rate, oxidation stability, and nutritional quality in rapeseed oils.[Citation16–Citation18] Some of the previous studies have reported the relationship between glucosinolate degradation and the pungent odor in cold pressed rapeseed oil.[Citation14,Citation19] The sulfur compounds from rapeseed oils possess low threshold and strong odor. Very few studies have reported the distribution of glucosinolates, volatile isothiocyanates and sulfur compounds, and pungent odor difference in different genetic types of rapeseed. In this study, we investigated the presence of pungent aroma compounds in raw and microwave heated Brassica napus, Brassica campestris, and Brassica juncea rapeseed samples. The main aim is to explain the relationship between the samples and pungent odors.
Materials and methods
Materials and chemicals
Brassica napus samples (about ~30 µmol/g glucosinolate of fat free, 41.7% fat content; 6.9% moisture content) were procured from Zhongyou Sunshine Seeds Co., Ltd. (Wuhan, P. R. China), Brassica campestris samples (about ~102 µmol/g glucosinolate of fat free, 47.7% fat content, 6.4% moisture content) were procured from the Oil Crops Research Institute, Chinese Academy of Agricultural Sciences, Wuhan City, P. R. China, and Brassica juncea samples (about ~ 130 µmol/g glucosinolate of fat free, 36.9% fat content, 6.9%, moisture content) were procured from the Institute of Economic Crops, Xinjiang Academy of Agricultural Sciences, Xinjiang Province, P. R. China.
Reagents
Analytical reagents were purchased from the National Drug Reagent Company (Shanghai, P.R. China); acetonitrile and methyl alcohol of chromatographic grade were purchased from Merck & Co., Ltd. (Darmstadt, Germany); 2-methyl-3- heptanone, sinigrin, and DEAE Sephadex were obtained from Sigma-Aldrich (USA); Glucotropaeolin was purchased from ChromaDex Co., Ltd. (USA).
Sample preparation
Two hundred grams of each rapeseed variety was heated in a microwave oven (MarsX, CEM, Matthews, NC, USA) at a frequency of 2450 MHz at a medium power of 400 W for 7 min, and the heating cycle was repeated for 5 times. The microwave-treated rapeseeds were cooled to room temperature, and then both raw and microwave-heated rapeseeds were pressed with an expeller (KOMET CA59G, IBG Monforts Oekotec GmbH & Co. KG, Germany) less than 90 ºC. The centrifuged oils were stored at 4°C for analysis. R-oil was raw rapeseed oil. M-oil was rapeseed oil from microwaved seed. Seed 1, seed 2 and seed 3 were, respectively, on behalf of Brassica napus, Brassica campestris and Brassica juncea.
Identification of targeted compounds
Two grams of oil was placed in a 10-mL headspace glass vial and kept on the auto-heating block at 40°C for 20 min to equilibrate. The volatiles were extracted with the fibers (CAR/DVB/PDMS, 2 cm) (Supelco, Bellefonte, PA, USA) at 40°C for 30 min. The SPME fibers were desorbed in injection port for 5 min, and the desorbed sample was injected by automatic sample injector (Gerstel, Germany) into the GC at 250°C. Comprehensive two-dimensional gas chromatography coupled with time-of-flight mass spectrometry (GC× GC-TOF/MS) was employed for analyzing the volatile samples (Pegasus 4 LECO, St. Joseph, MI, USA). The extracted compounds were chromatographed using two column system: the first column was DB-5MS (30 m × 250 μm × 0.25 μm), and the second column was DB-17HT (1.9 m × 100 μm × 0.15 μm). The flow rate of the carrier gas (helium) was maintained at 1.0 mL/min. Column 1 was maintained at 40°C (2 min), 5°C/min to 175°C (0 min), and 25°C/min to 245°C (5 min), and column 2 at 60°C (1 min), 6°C/min to 190°C (0 min), and 25°C/min to 260°C (5 min). The GC/MS transfer line was maintained at 280°C, the modulation time was 4 s, and the time-of-flight mass spectrometer was operated at the mass range of 38 to 388 m/z at 150 spectra/s. Data were collected and processed using LECO® Chroma TOF® v.4.40.2.3. All the samples were analyzed in triplicates. The result was expressed as the peak areas of volatile compounds
GC-O for AEDA analysis
Volatile compounds of the rapeseed oil were identified and characterized by headspace solid phase microextraction and gas chromatography-olfactometry-mass spectrometry. Serial dilutions were employed by changing the split ratio (1:2n, n = 1, 2, 3, 4, 5…) of each aroma extract from SPME until analysis. Spilt volumes were analyzed by GC-O-MS using a 7890GC/5975MS system (Agilent Technologies Inc., Palo Alto, CA) equipped with an olfactory detection port (Gerstel, Germany). DB-5 and DB-Wax column (30 m × 0.25 mm i.d. × 0.25 μm; J&W Scientific) was employed to separate samples with split 1:1 (by column length set) into a sniffing port, while the temperature of the transfer line was maintained at 280°C. The temperature was programmed from 40°C (2 min) to 220°C at a rate of 5°C/min. Helium was used as the carrier gas at a constant flow rate of 2.0 mL/min. The volatile aromas need be sniffed in time. The aroma odor of every split sample was sniffed by three experienced sensory members in order to avoid omission. FD factor was the dilution ratio when the volatile odor is no longer felt.
Flavor evaluation
A panel of four male and six female aroma experience staffs evaluated and described the intensity of the selected odors in raw and microwaved seeds using a seven-point linear scale from 0 (not smell) to 3 (strongly smell). The panelists were trained to detect and describe the odor qualities using different samples and standard compounds. Sensory evaluation was performed at room temperature in a sensory room equipped with single booths. The panelists received the samples (10 g) in glass vessels (adiabatically maintained at 40°C), and were asked to rank the intensities of the different aroma-attributes on a seven-point scale (0, 0.5, 1.0, 1.5, 2.0, 2.5, 3.0; where 0 indicates ‘not perceivable’ and 3 indicates ‘strongly perceivable’).
Individual glucosinolate determination
Rapeseed samples were chilled by liquid nitrogen and immediately crushed by a micro-pulverizer. The crushed rapeseed (200 mg) was placed into a 15-mL centrifuge tube containing 2 mL of 70% hot methanol and 200 μL of 5 mmol/L of internal standard solution. Sinigrin was used as an internal standard for Brassica napus and Brassica campestris, whereas glucotropaeolin was used for Brassica juncea mustard. The samples were kept on an ultrasonic bath at 55°C for 10 min, and then centrifuged at 5000 g for 5 min. The supernatant was collected. The precipitation was extracted with 2 mL of 70% methanol, and the process was repeated twice. The supernatants were combined and diluted to 5 mL with water. The extracted glucosinolates were purified with a 1.5 cm DEAE Sephadex A-25 anion exchange column. The column was washed twice with 6 mol/L of imidazole formate, followed by 1 mL of millipore water. Extracted glucosinolates were washed twice with 1 mL of 0.02 mmol/L sodium acetate. Sulfatase was added to the column, and the reaction was continued for 16 h at 35°C. Desulfoglucosinolates were eluted with 4 mL of millipore water. The elute factor was diluted to 5 mL with millipore water and filtered through a 0.22 μm filter before analysis.
High-performance liquid chromatography 1100 (Agilent, USA) with ultraviolet detector was used to analyze the individual glucosinolate. HPLC program was as follows: phase A was 100% water and phase B was 100% acetonitrile; 100% A run for 15 min, 75% A for next 4 min, and 100% A for next 4.5 min and re-equilibrated. The eluent was monitored at 229 nm (at 2 nm intervals), and the flow rate was 1.0 mL/min. Desulfoglucosinolates were identified by a comparison of their retention times.
Statistical analysis
All the graphs were drawn with Original 9.0.
Results and discussion
Key odors identification of isothiocyanate and sulfur compounds
Comprehensive two-dimensional gas chromatography and time-of-flight mass spectrometry (GC× GC-TOF/MS) identified different volatile compounds including five isothiocyanates and six sulfur compounds with low molecular weight. Two-dimensional GC analysis has extremely advantageous for analysis of volatiles and the off-odor from heated rapeseed oil compared to using different polar columns.[Citation20]
In this study, five volatiles isothiocyanates were identified in oils from seed 1 (Brassica napus) and seed 2 (Brassica campestris), including isopropyl isothiocyanate (IITC), allyl isothiocyanate (AITC), 1-isothiocyanato butane (1-ITC), 4-isothiocyanato-1-butene (4-BITC), and cyclopentyl isothiocyanate (CITC); However, IITC and CITC were not detected in Brassica juncea oil (from seed 3). Genetic differences among different Brassica species might be responsible for the variation in isothiocyanate profiles. Daun et al. reported the presence of 3-butenyl-, 4-pentenyl-, phenethyl isothiocyanate in commercial rapeseed oils.[Citation9] Analysis of identified six samples through gas chromatography-olfactometry suggested that the panelists could not sniff isopropyl-isothiocyanate (new) and cyclopentyl-isothiocyanate (new) due to their high thresholds or without odor report. Those two substances contribute little to pungent flavor in rapeseed oil.
The relationships between glucosinolate content and aroma profiles have been studied in broccoli, brussels sprouts and cauliflower.[Citation21–Citation23] The isothiocyanate derivatives of glucoiberin, sinigrin, and gluconapin were identified by comparing the mass spectra and retention times of those compounds with the previously reported values.[Citation24] Except isothiocyanate, sulfur compounds with low molecular weight also possess strong odors. Methanethiol, a highly volatile compound smells like gasoline; the compound imparts garlic odor to rapeseed oils and “rotten cabbage-like” odor to cabbages.[Citation25] In different studies, dimethyl sulfide (DMS) was reported to smell as “decayed cabbage”; DMS was sniffed identified as “onion” in their study. Dimethyl trisulfide (DMTS), an aroma compound derived from S-methylcysteine, imparts a spoiled and cooked cabbage-like odor to cabbages in the present study. We got the similar conclusion in our smell results, DMS, DMDS, and DMTS impart cabbage and onion which attribute to pungent flavor. In addition, we found that dimethyl sulfone (MS) and dimethyl sulfoxide (DMSO) both have contribution to the pungent odor (). However, the contribution of each substance is inconsistent in different samples.
Figure 1. The difference between isothiocyanate and sulfide-containing compounds in raw and microwaved seed oils.
1. Rapeseed oil from raw Brassica napus,2. rapeseed oil from microwaved Brassica napus3. rapeseed oil from raw Brassica campestris4. rapeseed oil from microwaved campestris5. rapeseed oil from raw Brassica juncea.6. rapeseed oil from microwaved Brassica juncea.
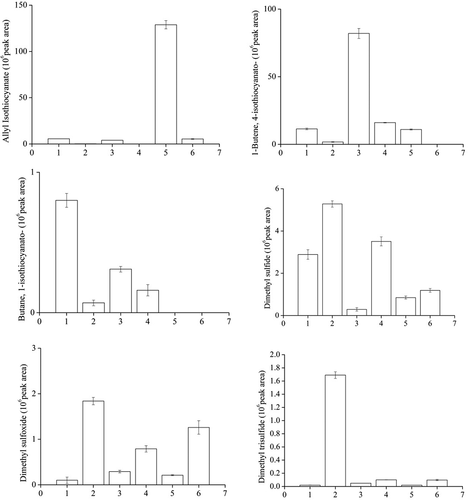
AEDA analysis and sensory evaluation
AEDA can detect and estimate the contribution of a volatile compound to the pungent aroma in oils. In raw rapeseed oil (Brassica napus), present that FD factors were estimated as 64 for allyl isothiocyanate (sulfur, pungent, green), 16 for1-isothiocyanato- butane (sulfur, pungent, garlic) and 128 for 4-isothiocyanato-1-butene (spicy, pungent), respectively. The aroma compound 4-isothiocyanate-1-butene significantly contributed to the pungent odor of the oils, as verified by the AEDA analysis.[Citation20] As previously reported, dimethyl sulphide, methallyl cyanide, dimethyl disulphide, 4-isothiocyanate-1-butene could help differentiate the whole-seed oils from peeled-seeds.[Citation26]
Table 1. The FD factor in different rapeseed oils based on genetic type and technology.
In Brassica napus oil, FD factors of dimethyl sulfide (DMS), dimethyl disulfide (DMDS) and dimethyl trisulfide (DMTS) were calculated as 32, 8 and 64, respectively, in microwaved oils, and these values were significantly higher than cold pressed oils as heating can significantly catalyze the formation of these compounds. Dimethyl sulfide is derived from methionine. FD factors of isothiocyanates were not reported in previous studies due to low glucosinolates content in rapeseed oils. In Brassica campestris oils, FD factors of 4-isothiocyanato-1-butene were highest in pungent odor, up to 256. In Brassica juncea oil, DMS, DMDS and DMTS can reach to 4, 32 and 4 in raw and microwaved oils. In addition, allyl isothiocyanate was, respectively, 128 and showed intense pungent oil which was observed different from the other two genotypes.
The FD factors of methanethiol in cold pressed and microwaved oils were estimated as 2 and 8, 2 and 4, 2 and 4, respectively, in three seed, hence it has the contribution is limit. FD factor of dimethyl sulfoxide (DMSO) was increased from 8 to 64 after heating in Brassica napus oil. Higher FD factor of dimethyl sulfide (DMS) in cold-pressed rapeseed oil was reported in various studies. For example, Dimethyl sulfide (FD = 128) was identified in cold-pressed rapeseed oil from peeled seeds using polar and non-polar columns. Eight odorants with FD factors between 4 and 2048 were identified, of which FD in Dimethyl trisulfide was 16. Dimethyl disulfide is derived from S-methylcysteine sulfoxide by the action of cysteine sulfoxide lyase, and the compound was not sniffed when the dilution is equal to 8 as Matheis’s report.[Citation14]
Trained experienced sensory members compared and ranked the aroma profiles of different rapeseed oils by their aromatic scores from 0 to 3 (). The grassy, green, cabbage-like, malty odor attributes were similar in all samples. All oil samples were sniffed as ‘oily’ and ‘herbaceous’, but the intensities of ‘roasted’ and ‘almond’ aroma odors differed significantly in various samples. This phenomenon agreed with the result of FD factors analysis. In the sensory evaluation, there are two big differences between three oils. For one, pungent and sulfur odor in seed 3 was strongest. For another, cabbage-like was most obvious in seed 1 than seed 2 and seed 3. After 7 min microwave treatment, the cabbage-like in three oils were stronger than raw oils, but the pungent like odor was weaker than before.
Change of glucosinolates as volatile flavor precusor
The total GLS levels in seed 1 (Brassica napus), seed 2 (Brassica campestris) and seed 3 (Brassica juncea) were 28.22 ± 0.78 μmol/g, 101.15 ± 2.95 μmol/g and 132.02 ± 9.31 μmol/g, respectively (). Thirteen different glucosinolates (progoitrin, epi-progoitrin, glucoraphanin, gluconapoleiferin, gluconapin, hydroxyglucobrassicin, glucobrassicanapin, glucotropaeolin, glucobrassicin, gluconasturtiin, 4-methoxygluconasturtiin, neoglucobrassicin, and sinigrin) were identified in the three ecotypes using high-performance liquid chromatography-mass spectrometry technology. Glucosinolates can be grouped into three chemical classes depending on their amino acid precursors: methionine, tryptophan, or aromatic amino acid (tyrosine or phenylalanine).[Citation27,Citation28] Methionine-derived glucosinolates are found in Brassica species.[Citation29] The Brassica samples contained 8 aliphatic, 3 indolyl, and 2 aromatic glucosinolates, which was agreed with Elbeltagi’s study [Citation19]; though, the total glucosinolate content was lower (4.40 to 5.90 µmol/g) in their study. The contents and odor intensities of degradation products generally depend upon the precursors.
Table 2. The change of individual glucosinolate in rapeseeds after microwaved treatment.
In the three genetic type of rapeseed, progoitrin (aliphatic glucosinolate), gluconapin (aliphatic glucosinolate), 4-hydroxyglucobrassicin (indolyl glucosinolate), and sinigrin (aliphatic glucosinolate) were the most abundant glucosinolates. Sinigrin is the characteristic glucosinolate in Brassica juncea. The individual components of Brassica napus and Brassica campestris were significantly different. The major glucosinolates in raw Brassica napus L. were progoitrin (9.29 ± 0.23 μmol/g), gluconapin (5.64 ± 0.11 μmol/g), and 4-hydroxyglucobrassicin (5.39 ± 0.04 μmol/g), which accounted for 72.00% of the total content. In raw Brassica campestris, progoitrin (6.00 ± 0.12 μmol/g), gluconapin (74.23 ± 2.11 μmol/g), and 4-hydroxyglucobrassicin (3.83 ± 0.12 μmol/g), and glucobrassicanapin (9.77 ± 0.21) constituted 89.85% of the total glucosinolate content (). Sinigrin (126.93 ± 8.21 μmol/g), gluconapin (3.14 ± 0.09 μmol/g) and 4-hydroxyglucobrassicin (9.77 ± 0.21 μmol/g) were the major glucosinolates in Brassica juncea.
The glucosinolates in rapeseed oils were degraded depending on enzymatic action and the thermal effects during the microwave process. The total glucosinolate content, respectively, decreased to 48.65%, 58.32%, and 60.25% in the three samples after 7 min of microwave heating (200 g, 400 W). Ebrahimi et al. reported that the content of total glucosinolate was decreased by 42%, 55%, and 59%, after 2, 4, and 6 min of microwave heating, respectively, which generally agreed with the outcome of the present study.[Citation30] Differences in the drying temperature and moisture content may be responsible for the difference. The enzymatic degradation depends on the temperature. During the heating process of rapeseed, the myrosinase activity in seeds started at 23°C and achieved the maximum value at 60°C.
The progoitrin, gluconapin and 4-hydroxyglucobrassicin in Brassica napus was, respectively, decreased from 9.29 ± 0.23 to 6.42 ± 0.23 μmol/g, 5.64 ± 0.11 to 4.08 ± 0.10 μmol/g and 5.39 ± 0.04 to 2.01 ± 0.05 μmol/g. After pressing, the peak area (106) of 4-isothiocyanato-1-butene (4-BITC) was 11.4 ± 0.56 to 1.74 ± 0.21. The peak area (106) of allyl isothiocyanate (AITC) in oil was from 5.63 ± 0.01 to 0.11 ± 0.00, meanwhile 1-isothiocyanato butane (1-ITC) from 0.8 ± 0.05 to 0.07 ± 0.02. The content of main individual glucosinolate in seed 2 was ranged from 6.00 ± 0.12 to 2.11 ± 0.12 μmol/g, 74.23 ± 2.11 to 35.67 ± 4.90 μmol/g, 9.77 ± 0.21 to 2.33 ± 0.02 μmol/g and 3.83 ± 0.12 to 2.33 ± 0.02 μmol/g, respectively, in progoitrin, gluconapin, glucobrassicanapin, and 4-hydroxyglucobrassicin. The outstanding contributors-4-BITC, AITC, and 1-ITC all have a great decrease in oils. One of progoitrin degradation products was confirmed as 3-hydroxy 4,5-epithio pentanenitrile.[Citation24] However, gluconapin is converted to 4-isothiocyanato-1-butene. The peak area decrease of 4-isothiocyanato-1-butene may be caused by the content of gluconapin in seeds. In addition, 4-hydroxyglucobrassicin is belong to indolyglucosinolates which are often quantitatively dominating in vegetative parts of cruciferous plants as rape and cabbage, however, relate degradation products were not be identified.
Sinigrin is hydrolyzed by myrosinase enzyme to allyl cyanide and AITC. Sinigrin content in microwaved Brassica juncea was decreased from 126.93 ± 8.21 μmol/g to 51.45 ± 6.41 μmol/g, but the variation of progoitrin and gluconapin content was limit. The peak area (106) of AITC in oils was from 128.86 ± 4.51 to 5.35 ± 0.39. 1-ITC were not be detected and no odors were smelled in seed 1 because of the lack of corresponding percusor. In addition, the source of isopropyl isothiocyanate (IITC) and cyclopentyl isothiocyanate (CITC) is not yet clear until now.
Effect of the genetic types and microwave treatment on pungent odors
From , there was significant difference on the genetic rapeseed and before and after microwave treatment. The peak area (× 106) of dimethyl sulfide was creased from 2.89 ± 0.23 to 5.28 ± 0.15, 0.29 ± 0.08 to 0.79 ± 0.07 and 0.21 ± 0.01 to 1.26 ± 0.15 in seed 1, seed 2 and seed 3. The increasing of dimethyl trisulfide from raw rapeseed oil to microwaved ones was 85 folds, 2 folds and 4 folds, respectively, in seed 1, seed 2 and seed 3. The amplitude of variation was close to the report in Katrin Matheis’s study.[Citation14] In their conclusion, dimethyl trisulfide was the most important odors in native cold-pressed rapeseed oil. In addition, it could elicit the fusty/musty off-flavor when its content increased.
In addition, dimethyl sulfone and dimethyl sulfoxide were increased from raw oil to microwaved oil (). The possible reason was methionine or isothiocyanate rearrangement. The trend of isothiocyanates was opposite to the small molecule sulfur compounds that allyl isothiocyanate, butane, 1-isothiocyanato- and 1-butene, 4-isothiocyanato- were decreased sharply compare microwaved seed oil to raw rapeseed oil, mainly relate to the degradation to the seed glucosinolates.
Isothiocyanates impart a characteristic pungent and sulfurous aroma in Brassica oils.[Citation31] Significant amounts of 4-BITC (pungent odor) was detected in Brassica napus and Brassica campestris, while AITC was detected in Brassica juncea. AITC is a degradation product of sinigrin. AITC in the canola oil might be generated from an unknown source, because canola seeds do not contain sinigrin.
AITC possesses a strong mustard odor and spicy smell.[Citation8] In rapeseed, sinigrin is hydrolyzed by myrosinase enzyme to pungent odor AITC, and gluconapin is converted to 44-BITC. In damaged rapeseed cells, the endogenous myrosinase hydrolyzes the precursor molecules, like progoitrin, gluconapin, hydroxyglucobrassicin, and sinigrin, into corresponding volatile compounds. The contents of the hydrolysates (aroma odor) depend upon the content of their type of precursor molecules, which may affect the intensity of the pungent flavor in rapeseed.
Besides volatile isothiocyanates, mono, di, and tri sulfides were found in the three varieties of rapeseed oils including raw seeds and microwaved seed. The glucose moiety of glucosinolate was hydrolyzed to form an unstable molecule.[Citation32] Hydrogen sulfide ions can be easily separated and broken down into isothiocyanates or cyanides, which may further convert into thiols, mono-sulfides, disulfides, and trisulfides in the three Brassica species. The isothiocyanates react with nucleophiles, and the reaction products of isothiocyanates and indol-3-ylmethylglucosinolates are rapidly transformed into indolyl thiocyanates.
In addition, some reports showed the bioactive sulfur compounds were existed in broccoli and other Brassicaceae plants.[Citation33] The glucosinolates and s-methylcysteine sulfoxide are presumed as the precursors of methylthiocyanate and dimethylsulfoxide.[Citation33] The formation of DMSO was possible involvement of glucosinolate products, non-protein bound amino acid and thiol-methyltransferase.
Previous studies have shown that thermal treatment can inactivate the myrosinase enzyme.[Citation34] Sinigrin, progoitrin, and gluconapin are aliphatic glucosinolate, while 4-hydroxyglucobrassicin is indolyl glucosinolate. Aliphatic glucosinolates (but, not indolyl glucosinolates) produce isothiocyanate and oxazolidine after enzymatic degradation. Significant research has been conducted to elucidate the degradation characteristics of the indole oligomers of GLS, which were generated in damaged cells due to temperature fluctuations during the microwave process. Many studies have reported that the glucosinolate continued to degrade because of the thermal effect, even after the myrosinase activity was subsided.[Citation9,Citation19] Indole glucosinolate −4-hydroxyglucobrassicin was significantly decomposed after 4, 5, and 6 min of heating.[Citation34] During the microwave process, the glucosinolate degradation may range from the effect of myrosinase activity.[Citation35] The residual glucosinolates were hydrolyzed rapidly during the brief exposure of microwaves, and AITC was generated during long exposure of microwaves until the myrosinase activity was subsided. In microwaved seed oils, the cabbage-like in three oils were stronger than raw oils, but the pungent like odor was weaker than before.
Conclusion
The total glucosinolate content decreased to 48.65%, 58.32%, and 60.25% in the three rapeseed samples after 7 min of microwave heating, respectively in Brassica napus seeds, Brassica campestris and Brassica juncea. Their main degradation products, 1-isothiocyanato butane, allyl isothiocyanate and 4-isothiocyanato-1-butene are responsible for the sulfur, pungent and green odors in rapeseed oils, contribute to the high flavor dilution factor. The contribution of isopropyl-isothiocyanate and cyclopentyl-isothiocyanate were due to their high thresholds. Those two substances contribute little to pungent flavor in rapeseed oil. Dimethyl sulfide, dimethyl trisulfide and dimethyl sulfoxide, imparted onion-like, cabbage-like, garlic-like, and sulfurous-pungent odors were smelled. Their possible source was may be derived from methionine or isothiocyanate rearrangement reactions. Differences in the concentrations of six main odorants and the glucosinolate precursors were responsible for the variation in the intensity of the pungent odors in raw and microwaved rapeseeds. The concentrations of glucosinolate and sulfur compounds can be correlated with the intensity of the pungent odor in different genotypes of rapeseed. Isothiocyanate from glucosinolates and sulfur compounds were the main source of pungent odors in rapeseed oils. Pungent odor was obvious in rapeseed oil, which may alter the acceptant of customs. The result can assist to the evaluation of pungent odors in the commercial rapeseed oils and provide a guide to rapeseed oil flavor quality.
Supplemental Material
Download ()Acknowledgments
Financial support was provided by the NSFC (31501528), The National Key Research and Development Program of China (2016YFD0401401), Agricultural Science and Technology Innovation Project of Chinese Academy of Agricultural Sciences (CAAS-ASTIP-2013-OCRI) and Earmarked Fund for China Agriculture Research System (CARS-13).
Supplementary Material
Supplemental data for this article can be accessed here.
Additional information
Funding
References
- Chambers, E.; Koppel, K. Associations of Volatile Compounds with Sensory Aroma and Flavor: The Complex Nature of Flavor. Molecules 2013, 18, 4887–4905. DOI: 10.3390/molecules18054887.
- Wei, F.; Yang, M.; Zhou, Q.; Zheng, C.; Peng, J.H.; Liu, C.S.; Huang, F.H.; Chen, H. Varietal and Processing Effects on the Volatile Profile of Rapeseed Oils. LWT-Food Science and Technology 2012, 48, 323–329. DOI: 10.1016/j.lwt.2012.04.007.
- Kushad, M.M.; Brown, A.F.; Kurilich, A.C.; Juvik, J.A.; Klein, B.P.; Wallig, M.A.; Jeffery, E.H. Variation of Glucosinolates in Vegetable Crops of Brassica Oleracea. Journal of Agricultural and Food Chemistry 1999, 47, 1541–1548. DOI: 10.1021/jf980985s.
- Fahey, J.W.; Zalcmann, A.T.; Talalay, P. The Chemical Diversity and Distribution of Glucosinolates and Isothiocyanantes among Plants. Phytochemistry 2001, 32, 5–51. DOI: 10.1016/S0031-9422(00)00316-2.
- Mithen, R.; Faulkner, K.; Magrath, R.; Rose, P.; Williamson, G.; Marquez, J. Development of Isothiocyanate-Enriched Broccoli, and Its Enhanced Ability to Induce Phase 2 Detoxification Enzymes in Mammalian Cells. Theoretical and Applied Genetics 2003, 106, 727–734. DOI: 10.1007/s00122-002-1123-x.
- Thiyam-Holländer, U.; Eskin, N.A.M.; Matthäus, B. Canola and Rapeseed: Production, Processing, Food Quality, and Nutrition; Crc Press: Boca Raton, FL, USA 2012; pp 45–67.
- Morra, M.J.; Borek, V. Glucosinolate Preservation in Stored Brassicaceae Seed Meals. Journal of Stored Products Research 2010, 46, 98–102. DOI: 10.1016/j.jspr.2009.12.001.
- Banerjee, A.; Variyar, P.S.; Chatterjee, S.; Sharma, A. Effect of Post Harvest Radiation Processing and Storage on the Volatile Oil Composition and Glucosinolate Profile of Cabbage. Food Chemistry 2014, 151, 22–30. DOI: 10.1016/j.foodchem.2013.11.055.
- Daun, J.K.; Hougen, F.W. Identification of Sulfur Compounds in Rapeseed Oil. Journal of the American Oil Chemists’ Society 1977, 54, 351–354. DOI: 10.1007/BF02802034.
- Jeleń, H.H.; Obuchowska, M.; Zawirska-Wojtasiak, R.; Wasowicz, E. Headspace Solid-Phase Microextraction Use for the Characterization of Volatile Compounds in Vegetable Oils of Different Sensory Quality. Journal of Agricultural and Food Chemistry 2000, 48, 2360–2367. DOI: 10.1021/jf991095v.
- Jeleń, H.H.; Mildnerszkudlarz, S.; JasińSka, I.; Wasowicz, E. A Headspace-SPME-MS Method for Monitoring Rapeseed Oil Autoxidation. Journal of the American Oil Chemists Society 2007, 84, 509–517. DOI: 10.1007/s11746-007-1072-2.
- Guth, H.; Grosch, W. Comparison of Stored Soya-Bean and Rapeseed Oils by Aroma Extract Dilution Analysis. LWT- Food Science and Technology 1990, 23, 59–65.
- Matheis, K.; Granvogl, M. Characterisation of the Key Aroma Compounds in Commercial Native Cold-Pressed Rapeseed Oil by Means of the Sensomics Approach. European Food Research and Technology 2016, 242, 1565–1575. DOI: 10.1007/s00217-016-2657-5.
- Matheis, K.; Granvogl, M. Characterization of Key Odorants Causing a Fusty/Musty Off-Flavor in Native Cold-Pressed Rapeseed Oil by Means of the Sensomics Approach. Journal of Agricultural and Food Chemistry 2016, 64, 8168–8178. DOI: 10.1021/acs.jafc.6b03527.
- Ortner, E.; Granvogl, M.; Schieberle, P. Elucidation of Thermally Induced Changes in Key Odorants of White Mustard Seeds (Sinapis Alba L.) And Rapeseeds (Brassica Napus L.) Using Molecular Sensory Science. Journal of Agricultural and Food Chemistry 2016, 64, 8179–8190. DOI: 10.1021/acs.jafc.6b03625.
- Koubaa, M.; Mhemdi, H.; Barba, F.J.; Roohinejad, S.; Greiner, R.; Vorobiev, E. Oilseed Treatment by Ultrasounds and Microwaves to Improve Oil Yield and Quality: An Overview. Food Research International 2016, 85, 59–66. DOI: 10.1016/j.foodres.2016.04.007.
- Uitterhaegen, E.; Evon, P. Twin-Screw Extrusion Technology for Vegetable Oil Extraction: A Review. Journal of Food Engineering 2017, 212, 190–200. DOI: 10.1016/j.jfoodeng.2017.06.006.
- Rękas, A.; Ścibisz, I.; Siger, A.; Wroniak, M. The Effect of Microwave Pretreatment of Seeds on the Stability and Degradation Kinetics of Phenolic Compounds in Rapeseed Oil during Long-Term Storage. Food Chemistry 2017, 222, 43–52. DOI: 10.1016/j.foodchem.2016.12.003.
- Elbeltagi, H.E.S.; Mohamed, A.A. Variations in Fatty Acid Composition, Glucosinolate Profile and Some Phytochemical Contents in Selected Oil Seed Rape Cultivars. Grasas Y Aceites 2010, 61, 143–150. DOI: 10.3989/gya.087009.
- Sghaier, L.; Cordella, C.B.Y.; Rutledge, D.N.; Watiez, M.; Breton, S.; Kopczuk, A.; Sassiat, P.; Thiebaut, D.; Vial, J. Comprehensive Two-Dimensional Gas Chromatography for Analysis of the Volatile Compounds and Fishy Odor Off-Flavors from Heated Rapeseed Oil. Chromatographia 2015, 78, 805–817. DOI: 10.1007/s10337-015-2897-8.
- Baik, H.Y.; Juvik, J.A.; Jeffery, E.H.; Wallig, M.A.; Kushad, M.; Klein, B.P. Relating Glucosinolate Content and Flavor of Broccoli Cultivars. Journal of Food Science 2003, 68, 1043–1050. DOI: 10.1111/j.1365-2621.2003.tb08285.x.
- Hansen, M.; Laustsen, A.M.; Olsen, C.E.; Poll, L.; Sorensen, H. Chemical and Sensory Quality of Broccoli (Brassica Oleracea L. Var Italica). Journal of Food Quality 2007, 20, 441–459. DOI: 10.1111/j.1745-4557.1997.tb00486.x.
- Schonhof, I.; Krumbein, A.; Brückner, B. Genotypic Effects on Glucosinolates and Sensory Properties of Broccoli and Cauliflower. Molecular Nutrition and Food Research 2004, 48, 25–33. DOI: 10.1002/food.200300329.
- Algendy, A.A.; Elgindi, O.D.; Hafez, A.S.; Ateya, A.M. Glucosinolates, Volatile Constituents and Biological Activities of Erysimum Corinthium Boiss. (Brassicaceae). Food Chemistry 2010, 118, 519–524. DOI: 10.1016/j.foodchem.2009.05.009.
- Chin, H.W.; Lindsay, R.C. Volatile Sulfur Compounds Formed in Disrupted Tissues of Different Cabbage Cultivars. Journal of Food Science 1993, 1993(58), 835–839. DOI: 10.1111/j.1365-2621.1993.tb09370.x.
- Gracka, A.; Raczyk, M.; Hradecký, J.; Hajslova, J.; Jeziorski, S.; Karlovits, G.; Michalak, B.; Bak, N.; Jelen, H.H. Volatile Compounds and Other Indicators of Quality for Cold-Pressed Rapeseed Oils Obtained from Peeled, Whole, Flaked and Roasted Seeds. European Journal of Lipid Science and Technology 2017, 119, 1–9. DOI: 10.1002/ejlt.201600328.
- Mithen, R.F. Glucosinolates and Their Degradation Products. Advances in Botanical Research 2001, 35, 213–232. DOI: 10.1016/S0065-2296(01)35008-5.
- Bjergegaard, C.; Møller, P.; Sørensen, H.; Sørensen, J.C.; Sørensen, S. Micellar Electrokinetic Capillary Chromatography of Thiocarbamoyl Derivatives Produced in Reactions between Isothiocyanates and Amino Acids. Journal of Chromatography A 1999, 836, 115–127. DOI: 10.1016/S0021-9673(99)00109-0.
- Mithen, R.; Bennett, R.; Marquez, J. Glucosinolate Biochemical Diversity and Innovation in the Brassicales. Phytochemistry 2010, 71, 2074–2086. DOI: 10.1016/j.phytochem.2010.09.017.
- Ebrahimi, S.R.; Nikkhah, A.; Sadeghi, A.A. Changes in Nutritive Value and Digestion Kinetics of Canola Seed Due to Microwave Irradiation. Asian Australasian Journal of Animal Sciences 2010, 23, 347–354. DOI: 10.5713/ajas.2010.80574.
- Fenwick’s, G.R.; Griffiths, N.M.; Heaney, R.K. Bitterness in Brussels Sprouts (Brassica Oleracea L. Var. Gemmifera): The Role of Glucosinolates and Their Breakdown Products. Journal of the Science of Food and Agriculture 1983, 34, 73–80. DOI: 10.1002/jsfa.2740340111.
- Zhang, X.M. Food Flavor Chemistry, China Light Industry Press: Beijing, China 2009; pp 36–40.
- Frandsen, H.B.; Møller, P.M.R.; Pedersen, K.W.E.; Sørensen, A.D.; Sørensen, J.C.; Sørensen, H. Bioactive Sulfur Compounds in Broccoli and Other Brassicaceae Plants; the Glucosinolates and S-Methylcysteine Sulfoxide as Precursors of Methylthiocyanate and Dimethylsulfoxide. Oncogene 2014, 25, 4332–4340.
- Niu, Y.X.; Rogiewicz, B.A.; Wan, C.Y.; Guo, P.M.; Huang, F.H.; Slominski, B.A. The Effect of Microwave Treatment on the Efficacy of Expeller Pressing of Brassica Napus Rapeseed and Brassica Juncea Mustard Seeds. Journal of Agricultural and Food Chemistry 2015, 63, 3078–3084. DOI: 10.1021/jf504872x.
- Eylen, D.V.; Indrawati,; Hendrickx, M.; Loey, A.V. Temperature and Pressure Stability of Mustard Seed (Sinapis Alba, L.) Myrosinase. Food Chemistry 2016, 97, 263–271. DOI: 10.1016/j.foodchem.2005.03.046.