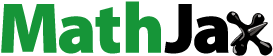
ABSTRACT
Changes in composition and structure of orange cuticle during storage at 4°C or 25°C for 40 days were investigated. The total epicuticular wax content of fruits increased during storage at 4°C for 30 days and then decreased as storage time prolonged to 40 days, while it increased continuously at 25°C for 40 days. The total intracuticular wax content of fruits increased to 9.70 μg cm−2 stored at 4°C for 10 days and then decreased to 6.74 μg cm−2 for 40 days. The total intracuticular wax content of fruits was decreased to 5.17 μg cm−2 stored at 25°C for 10 days and then increased to 10.06 μg cm−2 for 30 days. Fatty acids were the most abundant component of the epicuticular wax and terpenoids were restricted to the intracuticular wax. Terpenoids were restricted to the intracuticular wax, and their amounts in the fruit stored at 4°C increased continuously during the first 20 days of storage at 4°C and then decreased as storage time increased up to 40 days. Although significant changes were found in the contents of the cutin monomer, their proportions did not change significantly during storage at 4°C or 25°C. Size of wax platelets crystals wax increased during storage of fruits at 4°C up to 30 days; however, the crystals were degraded and decreased as storage prolonged to 40 days. Furthermore, the shape of the fruit cuticle surface wax crystals changed from flattened platelets to small granulelike after storage at 25°C for 20–40 days. The obtained results provide detailed information about the changes in orange cuticle occur during storage at varying temperatures, which may help in preserving the quality of citrus fruits during storage.
Introduction
Plant cuticle synthesized by the epidermis provides a hydrophobic barrier between plants and the environment.[Citation1] Despite their variable chemical compositions, plant cuticles consist of both cuticular waxes and cutin.[Citation2,Citation3] The primary functions of the plant cuticle are to minimize water loss, protect against physical, chemical, and biological attacks, as well as provide mechanical support to maintain organ integrity.[Citation4] Plant cuticular wax is mainly composed of very-long-chain fatty acids and their derivatives.[Citation5,Citation6] Plant cuticular waxes can be divided into two wax layers: the intracuticular wax, embedded in the cutin polymer matrix, and the epicuticular wax on the outer surface of the cutin polymer matrix.[Citation7,Citation8] Cutin is a polyester matrix composed mainly of hydroxylated and epoxy-hydroxylated C16 and C18 fatty acid monomers.[Citation4] Self-assembling of plant epicuticular wax during its development introduces diverse crystal structures, and the most predominant structures are platelet and plate-shaped crystals.[Citation9] Composition and structure of fruit cuticle influence postharvest storage significantly.[Citation4,Citation8] However, plant cuticles are affected by environmental factors, such as relative humidity, sunlight, and temperature.[Citation1,Citation10]
Citrus fruits are economically important, and their annual production in China has increased to 37.64 million tons in 2016.[Citation11] Fresh citrus fruits are perishable and susceptible to be infected by a pathogen if improperly handled, leading to a great loss of commercial value. Previous studies mainly focused on the evolution of the chemical composition of citrus cuticle during on-tree development. Liu et al.[Citation12] found that aldehydes are the most predominant compounds, and several kinds of terpenoids were identified in ‘Newhall’ orange during on-tree development. Wang et al.[Citation13] found that cutin accumulation is synchronous with fruit expansion, while wax synthesis is synchronized with fruit maturation. The formation of epicuticular wax crystals on the navel orange surface was found to depend on a high accumulation of aliphatic wax components and a trace amount of terpenoids.[Citation14] Cajuste et al.[Citation15] found that exogenous ethylene caused structural and compositional changes in surface waxes of ‘Navelate’ oranges during storage and suggested that the biosynthesis of cuticular waxes is controlled by the ethylene production. However, available information about the changes in composition and structure of mature citrus fruits cuticle during postharvest storage is limited. Meanwhile, the cuticle components and crystal structure of many other fruits have been well studied. Changes in the wax composition of ‘Elstar’ apple were found to be minor compared with those in ‘Jonagold’ and ‘Jonagored’ apples, which could be attributed to the lower diffusion resistance and stable structure of ‘Elstar’ wax compared with those of the latter two.[Citation16] 1-Methylcyclopropene inhibits the production of ethylene and delays the development of certain wax constituents, which are responsible for the occurrence of fruit surface greasiness during cold storage.[Citation17] In spite of being composed of similar chemical constituents, cultivar-related differences were found in cuticle composition during cold storage.[Citation18] Therefore, it is necessary to determine the changes in composition and crystal structure of citrus cuticle during postharvest storage to understand their influence on the fruit quality.
Sweet orange (Citrus sinensiscv ‘Bingtang’) fruit is a common local orange variety in Hunan Province, China. It is fleshly consumed and also a good raw material for juice production for its high content of vitamin C and bioactive compounds such as phenolic acids, flavonoids, limonoids, carotenoids, and fibers.[Citation19,Citation20] Also, the sweet orange by-products are used for animal and poultry feeding and also as alternative gelling agent in the food industry, as well as they are a good source for the extraction of and functional compounds.[Citation21–Citation25] Therefore, sweet orange cuticle was chosen as the experimental materials in the present study to determine the changes in fruit cuticle during storage at varying temperatures and to investigate the role of the cuticle in the maintenance of fruit quality. The objectives of this study are to (1) analyze the changes in chemical composition of mature sweet orange ‘Bingtang’ fruit cuticle during storage and (2) to characterize the changes in the crystal structure of the epicuticular wax of mature sweet orange ‘Bingtang’ fruit during storage.
Materials and methods
Experimental materials and storage conditions
Mature sweet orange ‘Bingtang’ fruits were harvested from Yongzhou, Hunan Province, China, on November 2016. After harvest, the fruits were delivered to the laboratory immediately. Fruits with uniformity of size and color, free from visible blemishes, disease, and/or physical damage were selected. A total of 450 fruits were divided to two groups, each containing the same number of fruits, and stored at 4°C or 25°C with a relative humidity of 85% for 40 days. A sample of 30 fruits was withdrawn every 10 days for cuticle analysis. The decayed samples in each replicate of 120 fruits were examined visually, counted every 10 days, and expressed as the percentage of rotting fruits. The fruit weight in replicates of 20 fruits each was recorded every 10 days. Weight loss (%) was determined by comparing the weight of the samples on the sampling day with their initial weight determined on day 0.
Determination of pericarp surface area
Fruit surface area was measured as described by Parsons et al.[Citation26] with slight modifications. Citrus fruit peel was weighed, and 40 disks (1.5 cm in diameter) were punched out. The surface area was calculated using the following formula:
where X is the weight of the fruit peel (g), D is the area of the 40 disks (cm), and Y is the weight of the 40 disks (g). Three replicates of 10 fruits each were used for the determination of pericarp surface area.
Cuticle extraction and analysis
Epicuticular and intracuticular wax extraction
Cuticular wax was extracted as described by Liu et al.[Citation14] Briefly, commercial gum arabic powder was used with chloroform to remove any soluble lipids and residues. Once dissolved in distilled water (1 g mL−1), the glue solution for epicuticular wax extraction was applied to the entire fruit surface using a small paintbrush and air-dried for 3–5 h until a dry and stable polymer film formed. Then, the polymer films were collected, dissolved in 30 mL of distilled water, and extracted with chloroform at room temperature, and the process was repeated twice. After the adhesive treatments, the intracuticular wax was extracted by immersing the same fruit twice in CHCl3 for 30 s. A volume of 200 µL (1 µg µL−1) n-tetracosane was added as an internal standard and dried completely under nitrogen gas (N2). The dried waxes were stored at −20°C before gas chromatography–mass spectrometry (GC-MS) analysis. Three replicates of 10 fruits each were used for epicuticular and intracuticular wax extraction.
Epicuticular and intracuticular wax analysis
Wax extracts were incubated in pyridine at 50°C for 30 min, followed by treatment with bis-N,N-(trimethylsilyl)-trifluoroacetamide (BSTFA, containing 1% trimethylchlorosilane; Sigma-Aldrich, Saint Louis, USA) at 60°C for 40 min. After the evaporation of excess BSTFA under N2, the samples were dissolved in chloroform for GC-MS analysis. Wax analysis was performed as described by Parsons et al.[Citation27] The derived wax mixtures were separated on a capillary GC (Agilent 7890A with an HP–5 MS column 30 m, 0.25 mm ID, 0.25 µm film) with He carrier gas inlet pressure programmed for a constant flow of 2 mL min−1 and a mass spectrometric detector (Agilent 5975C, 70 eV, m/z 50–600). GC was carried out with automatic temperature-programmed injection at 70°C and an oven temperature of 70°C. Temperature was held at 70°C for 1 min, increased to 200°C by 20°C min−1, held at 200°C for 2 min, increased to 300°C by 5°C min−1, and held at 300°C for 20 min. The individual wax components were identified by comparing their mass spectra with those of standards and literature data. Quantitative determination of wax components was performed by comparing their GC peak areas with those of standards. The amounts of cuticular wax components were normalized to these surfaces areas. Wax analysis was carried out in triplicates and expressed as mean values ± standard deviations.
Isolation and analysis of cutin
After the extraction of epicuticular and intracuticular waxes, the fruits were peeled for cutin isolation and analysis as described by Wang et al.[Citation28] with slight modifications. The enzyme solution was changed every 2 days, and the mixture was hydrolyzed until no cellular debris was released from the disks. Then, the obtained mixture was extracted with chloroform using 200 μL (1 μg μL−1) of n-tetracosane as an internal standard. The extracts were then evaporated in a stream of N2.
Free hydroxyl and carboxyl groups were converted into their trimethylsilyl ethers and esters with BSTFA in pyridine at 90°C for 40 min prior to GC-MS analysis. After the evaporation of excess BSTFA under N2, the samples were dissolved in chloroform for GC-MS analysis. The composition of cutin was determined by the same capillary GC. The oven temperature was programmed at 50°C for 2 min, increased to 150°C by 10°C min−1, held at 150°C for 1 min, increased to 290°C by 3°C min−1, and held at 290°C for 20 min. Cutin monomers were identified and quantified by the same method described above in epicuticular and intracuticular wax analysis.
Scanning electron microscopy analysis
Six replicates of one fruit each were used for scanning electron microscopy (SEM) analysis. Pericarp pieces (0.5 cm in diameter) from the equatorial section of six ‘Bingtang’ fruits stored at 4°C or 25°C were excised with a blade and then fixed in 2.5% glutaraldehyde solution. The dehydrated sample was coated with gold particles for 300 s with a 25 mA of voltage by an EDT-2000 ion sputtering apparatus. The coated samples were analyzed using a JEOL JSM-6380LV scanning electron microscope (SEM, 25KV). SEM analysis was performed every 10 days.
Statistical analysis
Statistical analyses were performed using the Origin 8.0 software. Data are presented as the mean ± SD of three replicates and compared by one-way analysis of variance. Significant differences among treatments were determined by t test at p ≤ 0.05 and indicated by different letters.
Results and discussion
Postharvest decay and weight loss
The decay rates of ‘Bingtang’ fruits stored at 4°C and 25°C for 40 days were 14.2% and 21.7%, respectively (). The fruits stored at 4°C began to rot after 20 days of storage, and the decay rate is 0.8%. However, the fruits stored at 25°C began to rot after 10 days, and the decay rate was 8.3%. These results can be attributed to that the growth and metabolism of pathogens are inhibited at 4°C, while the temperature of 25°C is convenient for pathogen growth. The cuticle provides protective purposes for covering stomata, cracks, or areas lacking wax and against pathogen penetration.[Citation29] Weight loss of the fruits significantly affected by storage temperature and time. The average daily weight loss rates of ‘Bingtang’ fruits stored at 4°C and 25°C are 0.05% and 0.2%, respectively (). After 40 days, the weight loss of the fruits stored at 4°C (2.1%) is significantly lower than that of the fruits stored at 25°C (8.3%). Similar results were found for Satsuma mandarin and Hamlin orange when stored at 10°C for 60 days.[Citation30] The weight loss rate of ‘Bingtang’ fruits stored at 25°C significantly increased during storage time from 20 to 30 days. It was reported that wax components play an important role in controlling of water movement through the cuticle compared with the wax amount.[Citation31,Citation32] Several studies concluded that fatty acids can prevent close molecular packing in the cuticle membrane and form a relatively loose network contributing to water loss.[Citation13,Citation33] In the present study, the fruits stored at 25°C exhibited a higher metabolic activity and higher weight loss than those of fruits stored at 4°C. It has been established that the cuticle composition and crystal structure of fruit are affected by environmental factors.[Citation1,Citation10] Therefore, the changes in the fruit cuticle during storage were investigated in this study.
The changes in the content of cuticle fractions
As shown in , the storage temperatures influenced the content of ‘Bingtang’ fruit cuticle fractions. Epicuticular wax content of fruit cuticle stored at 4°C increased from 1.63 to 4.14 µg cm−2 during 30 days of storage and decreased to 2.47 µg cm−2 during storage time between 30 and 40 days. On the other hand, the epicuticular wax content of fruit cuticle stored at 25°C increased from 1.63 to 9.79 µg cm−2 during a storage time of 40 days. Moreover, for the fruits stored for the same time or at the same temperature, amount of intracuticular wax is significantly higher than that of epicuticular wax. For the fruits at harvest time, intracuticular wax content was 5.4 times higher than that of epicuticular wax content. Except for the fruits stored at 4°C for 40 days and at 25°C for 10 days, the total wax content of the fruits increased along the storage time (). These results suggest that a redistribution of cuticle material took place during storage. The physiological mechanism for postharvest modification of cuticle modification is not well understood yet. These results are in a good agreement with the previous findings in which that total cuticle content per surface unit of sweet cherry fruit was found to be increased during cold storage.[Citation18] Also, this phenomenon was found for ‘Jesca’ peach fruits[Citation34] and suggested that the pathways involved in cuticle biosynthesis were upregulated during storage. Furthermore, the previous studies found that total wax contents remain unchanged for ‘Sturmer,’ increased for ‘Granny Smith’ and ‘Dougherty’ apples,[Citation35] or/and decreased for ‘Red Fuji’ samples during cold storage.[Citation36] The differences in patterns of wax accumulation may be attributed to the differences among cultivars or environmental conditions. Cajuste et al.[Citation15] found structural and compositional changes in surface waxes of ‘Navelate’ oranges during storage and suggested that the biosynthesis of cuticular waxes was controlled by ethylene.
Table 1. Amount of fractions in the cuticle of ‘Bingtang’ fruits during storage at different temperatures.
New wax formation plays a protective role, such as covering stomata, cracks or areas lacking wax, and against pathogen penetration.[Citation29] Raffaele et al.[Citation37] found that wax biosynthetic genes can be induced by bacterial pathogens. In this study, the sweet orange fruits stored at 25°C presented higher rotting rates and higher wax amount than those of fruits stored at 4°C (). Cutin is known as the main component of the ‘Bingtang’ fruits cuticle, and the changes in the content of cutin are consistent with those of cuticle content. The content of cutin is higher than that of wax, which is consistent with the previous finding for other fruits, such as navel oranges, tomato, and sweet cherry fruits.[Citation28,Citation38,Citation39] Cutin content decreased from 60.70 to 40.63 µg cm−2 when the fruits were stored at 4°C for 40 days. The previous studies found that content of cutin monomers tended to decrease during storage of Newhall fruit peel, which suggested that cutin might be degraded by lipase.[Citation40] In addition, most of the cutin-deficient Arabidopsis mutants showed a stronger resistance to Botrytis cinerea than the wild type.[Citation41] Also, previous research proved that the cutin-deficient Arabidopsis mutants presented higher permeability, resulting in the release of more reactive oxygen species to resist B. cinerea.[Citation42] Hence, it can be suggested that the degradation of fruit cutin during storage might induce the production of hydrogen peroxide and defense responses to improve the resistance to biological stresses.[Citation8] Cutin content of fruits stored at 25°C for 40 days increased by about 32.2%. Belge et al.[Citation34] found that cutin content of ‘Jesca’ peaches increased from 111.5 to 187.6 µg cm−2 as the samples stored at 20°C for 5 days. The similar trend of results was also found for cuticles as fruits stored at 4°C and 25°C in this study.
The changes in the composition of epicuticular wax
Epicuticular wax constituents of ‘Bingtang’ fruits stored at 4°C and 25°C for 40 days are presented in . Totally, 16 different components, including seven fatty acids, seven alkanes, one alcohol, and one terpenoid, were identified in the epicuticular wax of fruits at harvest time. Epicuticular wax mainly composed of fatty acids (63%), followed by alkanes (18%), alcohols (4%), and terpenoids (3%) (). Liu et al.[Citation14] found that epicuticular wax of the mature wild Newhall fruits was composed of fatty acids, n-alkanes, alcohols, and terpenoids. However, Wang et al.[Citation13] found that the epicuticular wax of Newhall fruits was mostly composed of aldehydes (58%), followed by fatty acids (21%) and alkanes (16%). The inconsistency among this study and these studies can be ascribed to the differences between fruit types and cultivars. Apart from the 16 components mentioned above, pentadecanoic acid, eicosanoic acid, docosanoic acid, octadecane, and squalene were identified in the stored samples, especially in the latter time of storage. Long-chain fatty acids presented the highest content in the epicuticular wax. Among them, hexadecanoic acid and octadecanoic acid are the predominant components. The total content of fatty acids in the epicuticular wax of fruits stored at 4°C increased from 1.22 to 2.04 μg cm−2 during the first 20 days of storage time and then decreased to 1.42 μg cm−2 during the later time of storage between 20 and 40 days. However, the total content of fatty acids increased significantly during the first 20 days of storage time and then remained stable during the later time of storage up to 40 days for fruits stored at 25°C. Moreover, significant higher long-chain fatty acid contents were found for fruits stored at 25°C than those of fruits stored at 4°C for the same time of storage. Previous studies established that environmental temperature affects the epicuticular wax composition; particularly, more fatty acids and products of the reductive pathways are produced at a higher temperature and more decarbonylated products at a lower temperature of storage.[Citation10,Citation43] In this work, both fatty acids and decarbonylated products of the epicuticular wax are higher for fruits stored at 25°C than those of fruits stored at 4°C. It was suggested that different cultivars may have different sensitivity to environment temperature.[Citation10] Contents of the two main long-chain fatty acids, hexadecanoic acid, and octadecanoic acid, also showed a similar trend with that of total contents of fatty acids during storage at 4°C and 25°C. For the fatty acid proportions to the epicuticular wax, it remained stable when the fruits were stored at 4°C, while decreased significantly from 63% (0 day) to 29% (40 days) at 25°C (). An increase in alkanes was found for fruits stored at 25°C with chain lengths of C23–C31; however, the alkane compositions in the fruits stored at 4°C remained unchanged. Octadecane was undetected during the first 20 days of storage. With further storage time up to 40 days, nonacosane content was increased to 0.83 µg cm−2 and dominated the alkane fractions at 25°C. The alkane proportion to the epicuticular wax of the fruits stored at 4°C decreased from 18% (0 day) to 4% (40 days); however, it increased from 18% to 24% as the fruit stored at 25°C for 40 days (). 13-Docosen-ol is the unique alcohol of the epicuticular wax and continuously accumulated in the fruits stored at 25 °C, while its accumulation in the fruits stored at 4°C initially increased and subsequently decreased. The alcohol proportion to the epicuticular wax showed little variation when the fruits were stored at 4°C, while increased significantly from 4% (0 day) to 13% (40 days) at 25°C. Yang et al.[Citation44] found that alkanes, such as nonacosane, and fatty alcohols, such as nonacosan-10-ol, contributed to the formation of the greasy three-dimensional structure of waxes as ‘Jonagold’ apples stored at 20°C for 20 days. The terpenoid proportion to the epicuticular wax increased 9.3 times at 4°C and 4.0 times at 25°C during storage for 40 days. Friedelan-3-one and squalene were detected in trace amounts in the epicuticular wax. With prolonged storage time, the amounts of squalene and friedelan-3-one increased significantly in the fruit stored at 25°C and 4°C.
Table 2. Epicuticular wax constituents of ‘Bingtang’ fruit cuticle during storage at 4◦C and 25◦C for 40 days.
The changes in the composition of intracuticular wax
Intracuticular wax constituents of ‘Bingtang’ fruits stored at 4°C and 25°C for 40 days are presented in . A number of 19 intracuticular wax components, including seven fatty acids, five alkanes, one alcohol, and six terpenoids, were detected in ‘Bingtang’ fruits at harvest time. Fatty acids occupied the highest proportion (39%) of the total intracuticular wax at harvest time, followed by terpenoids (20%), alcohols (20%), and alkanes (15%) (). The percentage of fatty acids to the total intracuticular wax increased during storage time from 0 day to 10 days and decreased with prolonged storage time at 4°C. Moreover, the percentage of fatty acids decreased to 30% and became the second abundant intracuticular wax component in the fruit stored at 25°C for 40 days. Tetracosanoic acid, followed by hexadecanoic acid, was found to be the predominant fatty acid component in the intracuticular wax, and their contents account about 51.1% of the total fatty acids. Liu et al.[Citation14] found that n-hexadecanoic acid, followed by n-octadecanoic acid, was the predominant n-fatty acid during the time from 60 to 120 days of after blooming, while n-hexacosanoic acid, n-octacosanoic acid, and n-triacontanoic acid became the predominant n-fatty acid components during 180–210 days after blooming. In this study, cis-9-hexadecanoic acid and eicosanoic acid could not be detected in the initial storage period, while these were detected in lower amounts after storage of fruits at 4°C and 25°C for 40 days. The amounts of n-hexadecanoic acid and octadecanoic acid sharply increased by 1.9-fold and 9.1-fold in the fruit stored at 4°C for 10 days but decreased by 5.7-fold and 1.9-fold in the fruit stored at 25°C for 10 days, respectively (). Only seven alkane components, i.e., heneicosane, tricosane, pentacosane, heptacosane, nonacosane, and hentriacontane, were detected during storage. Nonacosane was found to be the most abundant alkane in fruits, and its amount ranged from 0.21 to 0.73 µg cm−2 during storage. The total contents of alkanes in intracuticular wax of fruits stored at 25°C are significantly higher than those of fruits stored at 4°C for the same time of storage. The similar trend of results was also found for nonacosane and heptacosane. Eight terpenoids, namely fridelan-3-one, β-amyrin, lup-20(29)-en-3-one, urs-12-en-24-oic acid, farnesol, squalene, campesterol, and stigmasterol, were detected in the intracuticular wax fraction (). Terpenoids are mainly located in the internal part of the intracuticular wax of the fruits, which was previously reported for fruits of citrus,[Citation13] tomato,[Citation31] and leaves of Ligustrum vulgare.[Citation45] Terpenoids are the most abundant component in the intracuticular wax of the fruit stored at 25°C, accounted for about 39% of the intracuticular wax after 40 days of storage. In agreement with the previous studies, the current study further approved that fruit epicuticular waxes were predominantly consisted of aliphatic compounds. A similar pattern of aliphatics was found in the intracuticular wax compartment mixed with a large number of cyclics.[Citation12–Citation14] The terpenoid proportion of the total intracuticular wax increased from 20% at 0 day to 35% at 20 days of storage and then declined from 27% at 30 days to 20% at 40 days of storage at 4°C. On the other hand, the terpenoid proportion increased from 20% to 39% as the fruits stored at 25°C for 40 days (). The total terpenoid content of fruit cuticle intracuticular wax showed the highest value (2.94 μg cm−2) after 20 days of storage and then decreased to 1.32 μg cm−2 after 40 days of storage at 25°C. A similar trend was found for the farnesol and squalene. Terpenoid content of fruits stored at 25°C was higher than that of fruits stored at 4°C, which is consistent with the higher weight loss of fruits stored at 25°C (). The weight loss was found to be negatively associated with the ratio of total aliphatics to terpenoids (r = −0.60, P < 0.08) and the aliphatics to terpenoids amount in epicuticular wax (r = −0.54, P = 0.14). These results are consistent with the previous studies.[Citation26,Citation27] Aliphatic compounds are lacking the capacity of forming an impermeable region, but the terpenoid compounds with highly complex structure can disrupt molecular packing in the cuticle membrane and thus produces highly permeable cuticle. Furthermore, terpenoids are predicted to be located in the amorphous matrix, and aliphatics are predicted to be located in the crystalline regions.[Citation27,Citation32] Generally, changes in contents of lup-20(29)-en-3-one, β-amyrin, urs-12-en24-oic acid, and friedelan-3-one in the intracuticular wax of the fruits showed a gradual decline during storage at 25°C. However, the total content of terpenoids increased significantly from 2.28 to 3.63 μg cm−2 during fruit storage at 4°C for 40 days. This can be attributed to the increase of squalene, which increased 4.3 times during storage for 40 days.
Table 3. Intracuticular wax constituents of ‘Bingtang’ fruit cuticle during storage at 4◦C and 25◦C for 40 days.
The changes in cutin monomers
The changes in content of cutin monomers of ‘Bingtang’ fruits during storage are presented in . Nine cutin monomers, namely cinnamic acid, pentadecanoic acid, hexadecanoic acid, hexadecanedioic acid, cis-9-hexadecenoic acid, octadecanoic acid, octadecanedioic acid, docosanoic acid, and phenol, were identified in samples at harvest time. Cis-9-hexadecenoic acid and cinnamic acid were found to be the predominant components of the cutin monomers, which is different from the findings of Wang et al.[Citation28] These two components remained the main cutin monomers of ‘Bingtang’ fruits during storage at 4°C and 25°C. No significant differences were found in the proportions of each cutin monomer between the fruits stored at 4°C and fruits stored at 25°C (). Octadecanedioic acid monomer could not be detected in the fruit stored at 4°C between 20 and 40 days of the storage period, and tetracosane acid was only detected in the fruit stored at 25°C. The higher cutin content in the fruit stored at 25◦C accompanied by higher weight loss suggests that cutin does not function as a main hydrophobic barrier but rather provides a framework for cuticular wax deposition.[Citation38] This was approved by the regression analysis, which showed a positive association between weight loss and amount of cutin or cutin monomers. These results are in a good agreement with changes in cuticle lipid composition and water loss of pepper (Capsicum).[Citation27] However, Parsons et al.[Citation26] found that certain monomers of pepper cutin showed a negative association with water loss. The inconsistency among different studies may be attributed to the differences in the composition of different cultivar cuticle.
Table 4. Cutin monomer composition of ‘Bingtang’ fruits during storage at 4◦C and 25◦C for 40 days.
The changes in the crystalline structure of epicuticular wax
Scanning electron microscopy (SEM) analysis indicated that the epicuticular wax crystals of ‘Bingtang’ fruits are presented in flattened platelet form at harvest time (), which are common in mature citrus fruits. These small platelet crystals on the surface of the fruits became applanate and wrinkled shaped after 20 days of storage at 4°C (). Then, these crystals distributed continuously, and cracks were emerged on the entire fruit surface after 30 days of storage (). However, with prolonged storage up to 40 days, the crystals on the surface of fruits stored at 4°C degraded and decreased ().
Figure 5. Epicuticular wax crystal structure of ‘Bingtang’ fruits during storage for 40 days as imaged at ×400 and ×1500 magnifications. (a): Samples at harvest. (b–e): Samples stored at 4°C from 10 to 40 days. (f–i): Samples stored at 25°C from 10 to 40 days.
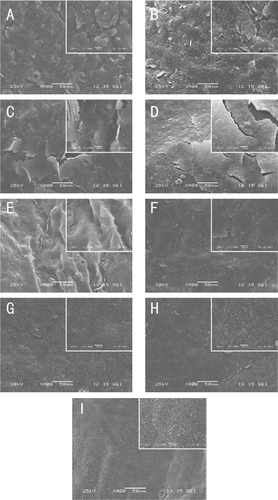
For fruits stored at 25°C, the shape of the surface crystals changed from flattened platelets to small granule-like wax crystals after storage for 20–40 days. A high magnification image indicated that these small granule-like wax crystals increased gradually and clustered together with prolonged storage time (). The wax crystals with three-dimensional structures on plant surface arise from self-assembly of the wax compounds, and their changes can cause a transition in wax morphology and appearance at the cuticle interface.[Citation9,Citation14] The alkanes and fatty alcohols are known crystalline compounds[Citation46] and determine the crystal structure of the epicuticular waxes.[Citation14] In this study, the contents of alkanes and alcohols increased significantly during storage at 25°C (). This suggests that, as structure substances, the changes in contents of the solid compounds should be reflected in changes of epicuticular crystal structures. Interestingly, the new-formed granule-like wax crystals are confirmed by the SEM images. Similarly, the decrease and degradation of the epicuticular wax crystals during storage at 4°C for 30–40 days could be ascribed to the reduction of alkane and alcohol contents (). The crystal structure is affected by storage temperature, and this is in accordance with the results of other studies. For example, it was suggested that low temperature is associated with larger platelet size because of the greater chilling injury.[Citation47] Also, heat treatment was found to cause a rearrangement of the epicuticular wax layers, which minimize or eliminate cracking.[Citation48] However, Shepherd et al.[Citation10] concluded that higher temperatures contributed to plates crystal structure, while lower temperatures favor more vertical structures such as rods and tubes. Simultaneously, the alteration of epicuticular components is another important reason for crystal structure changes. Furthermore, alkane plays an important role in assembling flattened platelets.[Citation13] In this study, the alkane proportion to epicuticular wax was gradually decreased during storage at 4°C, while it increased during storage at 25°C for 40 days. These changes in alkane are consisted with the changes in crystal structure. Baker[Citation49] found that temperature and crystallization rate are more significant than soluble concentration and chemical composition in determining wax crystal structure. Therefore, the influence of temperature on crystal structure should be further investigated. Crystal structure of fruit surface plays an important role in the reduction of water loss.[Citation48] In this study, the changes in the crystal structure of fruits during storage at 25°C are consistent with the higher weight loss. The granule-like structure of crystal may have a positive effect on weight loss. Also, the effect of stomata morphology on water loss should be considered.
Conclusion
This study investigated the changes in structure and chemical composition of sweet orange ‘Bingtang’ fruit cuticle during storage at 4°C or 25°C for 40 days. The results indicated that storage temperature affects cuticle wax accumulation and results in changes in wax crystal structure. Also, the current study further confirms that the fruit epicuticular waxes predominantly consist of aliphatic compounds, and similar aliphatics are present in the intracuticular wax, but they are mixed with a large number of cyclics. Furthermore, the degradation of cutin of sweet orange fruits during storage at 4°C may improve the resistance to biological and other environmental stresses. The results obtained in this study provide new understandings and explanations for the changes in the cuticle of sweet orange ‘Bingtang’ during storage at different temperatures and provide important information, which may help in preserving of citrus fruit quality during storage.
Acknowledgments
The study was supported by the National Natural Science Foundation of China (31501543), Natural Science Foundation of Hunan Province, China (2016JJ6077), and Agricultural Science and Technology Innovation Project of Hunan Province, China (2017GC03).
Additional information
Funding
References
- Domínguez, E.; Cuartero, J.; Heredia, A. An Overview of Plant Cuticle Biomechanics. Plant Science. 2011, 181, 77–84. DOI: 10.1016/j.plantsci.2011.04.010.
- Järvinen, R.; Kaimainen, M.; Kallio, H. Cutin Composition of Selected Northern Berries and Seeds. Food Chemistry. 2010, 122, 137–144. DOI: 10.1016/j.foodchem.2010.02.030.
- Szakiel, A.; Paczkowski, C.; Pensec, F.; Bertsch, C. Fruit Cuticular Waxes as a Source of Biologically Active Triterpenoids. Phytochemistry Reviews. 2012, 11, 263–284. DOI: 10.1007/s11101-012-9241-9.
- Lara, I.; Belge, B.; Goulao, L.F. The Fruit Cuticle as a Modulator of Postharvest Quality. Postharvest Biology and Technology. 2014, 87, 103–112. DOI: 10.1016/j.postharvbio.2013.08.012.
- Bernard, A.; Joubès, J. Arabidopsis Cuticular Waxes: Advances in Synthesis, Export and Regulation. Progress in Lipid Research. 2013, 52, 110−129. DOI: 10.1016/j.plipres.2012.10.002.
- Kunst, L.; Samuels, L. Plant Cuticles Shine: Advances in Wax Biosynthesis and Export. Current Opinion in Plant Biology. 2009, 12, 721–727. DOI: 10.1016/j.pbi.2009.09.009.
- Koch, K.; Ensikat, H.J. The Hydrophobic Coatings of Plant Surfaces: Epicuticular Wax Crystals and Their Morphologies, Crystallinity and Molecular Self-Assembly. Micron. 2008, 39, 759–772. DOI: 10.1016/j.micron.2006.12.008.
- Yeats, T.H.; Rose, J.K.C. The Formation and Function of Plant Cuticles. Plant Physiology. 2013, 163, 5–20. DOI: 10.1104/pp.113.222737.
- Koch, K.; Ensikat, H. The Hydrophobic Coatings of Plant Surfaces: Epicuticualr Wax Crystals and Their Morphologies, Crystallinity and Molecular Self-Assembly. Micron. 2008, 39, 759–772. DOI: 10.1016/j.micron.2007.11.010.
- Shepherd, T.; Griffiths, D.W. The Effect of Stress on Plant Cuticular Waxes. New Phytologist. 2006, 171, 469–499. DOI: 10.1111/j.1469-8137.2006.01756.x.
- National Bureau of Statistics. China Statistical Yearbook; China Statistical Publishing House: Beijing, 2017.
- Liu, D.C.; Zeng, Q.; Ji, Q.X.; Liu, C.F.; Liu, S.B.; Liu, Y.A. Comparison of the Ultrastructure and Composition of Fruits’ Cuticular Wax from the Wild-Type ‘Newhall’ Navel Orange (Citrus sinensis [L.] Osbeck Cv. Newhall) and Its Glossy Mutant. Plant Cell Reports. 2012, 31, 2239–2246. DOI: 10.1007/s00299-012-1250-z.
- Wang, J.Q.; Hao, H.; Liu, R.; Ma, Q.; Xu, J.; Chen, F.; Cheng, Y.; Deng, X. Comparative Analysis of Surface Wax in Mature Fruits between Satsuma Mandarin (Citrus unshiu) and ‘Newhall’ Navel Orange (Citrus sinensis) from the Perspective of Crystal Morphology, Chemical Composition and Key Gene Expression. Food Chemistry. 2014, 153, 177–185. DOI: 10.1016/j.foodchem.2013.12.021.
- Liu, D.C.; Yang, L.; Zheng, Q.; Wang, Y.C.; Wang, M.L.; Zhuang, X.; Wu, Q.; Liu, C.F.; Liu, S.B.; Liu, Y. Analysis of Cuticular Wax Constituents and Genes that Contribute to the Formation of ‘Glossy Newhall’, a Spontaneous Bud Mutant from the Wild-Type ‘Newhall’ Navel Orange. Plant Molecular Biology. 2015, 88, 573–590. DOI: 10.1007/s11103-015-0343-9.
- Cajuste, J.F.; González-Candelas, L.; Veyrat, A.; García-Breijo, F.J.; Reig-Armiñana, J.; Lafuente, M.T. Epicuticular Wax Content and Morphology as Related to Ethylene and Storage Performance of ‘Navelate’ Orange Fruit. Postharvest Biology and Technology. 2010, 55, 29−35. DOI: 10.1016/j.postharvbio.2009.07.005.
- Veraverbeke, E.A.; Lammertyn, J.; Saevels, S.; Nicolaï, B.M. Changes in Chemical Wax Composition of Three Different Apple (Malus domestica Borkh.) Cultivars during Storage. Postharvest Biology and Technology. 2001, 23, 197−208. DOI: 10.1016/S0925-5214(01)00128-4.
- Curry, E.;. Effects of 1-MCP Applied Postharvest on Epicuticular Wax of Apples (Malus domestica Borkh.) During Storage. Journal of the Science of Food and Agriculture. 2008, 88, 996−1006. DOI: 10.1002/jsfa.3180.
- Belge, B.; Llovera, M.; Comabella, E.; Gatius, F.; Guillén, P.; Graell, J.; Lara, I. Characterization of Cuticle Composition after Cold Storage of “Celeste” and “Somerset” Sweet Cherry Fruit. Journal of Agricultural and Food Chemistry. 2014, 62, 8722–9729. DOI: 10.1021/jf502650t.
- Russo, M.; Bonaccorsi, I.; Inferrera, V.; Dugo, P.; Mondello, L. Underestimated Sources of Flavonoids, Limonoids and Dietary Fiber: Availability in Orange’s By-Products. Journal of Functional Foods. 2015, 12, 150–157. DOI: 10.1016/j.jff.2014.11.008.
- Wibowo, S.; Vervoort, L.; Tomic, J.; Santiago, J.S.; Lemmens, L.; Panozzo, A.; Grauwet, T.; Hendrickx, M.; Van Loey, A. Colour and Carotenoid Changes of Pasteurised Orange Juice during Storage. Food Chemistry. 2015, 171, 354–361. DOI: 10.1016/j.foodchem.2014.09.007.
- Ebrahimi, A.; Qotbi, A.A.A.; Seidavi, A.R.; Laudadio, V.; Tufarelli, V. Effect of Different Levels of Dried Sweet Orange (Citrus sinensis) Peel on Broiler Chickens Growth Performance. Archiv Fur Tierzucht- Archives of Animal Breeding. 2013, 56, 11–17. DOI: 10.7482/0003-9438-56-002.
- Pourhossein, Z.; Qotbi, A.A.A.; Seidavi, A.R.; Laudadio, V.; Centoducati, G.; Tufarelli, V. Effect of Different Levels of Dietary Sweet Orange (Citrus sinensis) Peel Extract on Humoral Immune System Responses in Broiler Chickens. Animal Science Journal. 2015, 86, 105–110. DOI: 10.1111/asj.12250.
- Ying, Y.; Niu, M.; Clarke, A.R.; Harvatine, K.J. Effect of a Citrus Extract in Lactating Dairy Cows. Journal of Dairy Science. 2017, 100, 5468–5471. DOI: 10.3168/jds.2016-12233.
- Sicari, V.; Pellicano, T.M.; Lagana, V.; Poiana, M., Use of Orange By-Products (Dry Peel) as an Alternative Gelling Agent for Marmalade Production: Evaluation of Antioxidant Activity and Inhibition of HMF Formation during Different Storage Temperature. Journal of Food Processing and Preservation. 2018, 42, e13429. DOI: 10.1111/jfpp.13429.
- Putnik, P.; Kovacevic, D.B.; Jambrak, A.R.; Barba, F.J.; Cravotto, G.; Binello, A.; Lorenzo, J.M.; Shpigelman, A., Innovative “Green” and Novel Strategies for the Extraction of Bioactive Added Value Compounds from Citrus Wastes-A Review. Molecules. 2017, 22, 680. DOI: 10.3390/molecules22050680.
- Parsons, E.P.; Popopvsky, S.; Lohrey, G.T.; Lü, S.; Alkalai-Tuvia, S.; Perzelan, Y.; Paran, I.; Fallik, E.; Jenks, M.A. Fruit Cuticle Lipid Composition and Fruit Postharvest Water Loss in an Advanced Backcross Generation of Pepper (Capsicum Sp.). Physiologia Plantarum. 2012, 146, 15–25. DOI: 10.1111/j.1399-3054.2012.01598.x.
- Parsons, E.P.; Popopvsky, S.; Lohrey, G.T.; Lü, S.; Alkalai-Tuvia, S.; Perzelan, Y.; Bosland, P.; Bebeli, P.J.; Paran, I.; Fallik, E.;; et al. Fruit Cuticle Lipid Composition and Water Loss in a Diverse Collection of Pepper (Capsicum). Physiologia Plantarum. 2013, 149, 160–174. DOI: 10.1111/ppl.12035.
- Wang, J.Q.; Sun, L.; Xie, L.; He, Y.Z.; Luo, T.; Sheng, L.; Luo, Y.; Zeng, Y.L.; Xu, J.; Deng, X.X.;; et al. Regulation of Cuticle Formation during Fruit Development and Ripening in ‘Newhall’ Navel Orange (Citrus sinensis Osbeck) Revealed by Transcriptomic and Metabolomic Profiling. Plant Science. 2016, 243, 131–144. DOI: 10.1016/j.plantsci.2015.12.010.
- Dore, A.; Molinu, M.G.; Venditti, T.; D’Hallewin, G. Sodium Carbonate Induces Crystalline Wax Generation, Activates Host-Resistance, and Increases Imazalil Level in Rind Wounds of Oranges, Improving the Control of Green Mold during Storage. Journal of Agricultural and Food Chemistry. 2010, 58, 7297−7304. DOI: 10.1021/jf101013j.
- Shen, Y.; Yang, H.; Chen, J.; Liu, D.H.; Ye, X.Q. Effect of Waxing and Wrapping on the Phenolic Content and Antioxidant Activity of Citrus during Storage. Journal of Food Processing and Preservation. 2013, 37, 222–231. DOI: 10.1111/j.1745-4549.2011.00639.x.
- Leide, J.; Hildebrandt, U.; Vogg, G.; Riederer, M. The Positional Sterile (PS) Mutation Affects Cuticular Transpiration and Wax Biosynthesis of Tomato Fruits. Journal of Plant Physiology. 2011, 168, 871–877. DOI: 10.1016/j.jplph.2010.11.014.
- Vogg, G.; Fischer, S.; Leide, J.; Emmanuel, E.; Jetter, R.; Levy, A.A.; Riederer, M. Tomato Fruit Cuticular Waxes and Their Effects on Transpiration Barrier Properties: Functional Characterization of a Mutant Deficient in a Very-Long-Chain Fatty Acid β-Ketoacyl-CoA Synthase. Journal of Experimental Botany. 2004, 55, 1401–1410. DOI: 10.1093/jxb/erh013.
- Kosma, D.K.; Bourdenx, B.; Bernard, A.; Parsons, E.P.; Lü, S.; Joubès, J.; Jenks, M.A. The Impact of Water Deficiency on Leaf Cuticle Lipids of Arabidopsis. Plant Physiology. 2009, 151, 1918–1929. DOI: 10.1104/pp.109.146589.
- Belge, B.; Llovera, M.; Comabella, E.; Graell, J.; Lara, I. Fruit Cuticle Composition of a Melting and a Nonmelting Peach Cultivar. Journal of Agricultural and Food Chemistry. 2014, 62, 3488−3495. DOI: 10.1021/jf5003528.
- Morice, I.M.; Shortland, F.B. Composition of the Surface Waxes of Apple Fruits and Changes during Storage. Journal of the Science of Food and Agriculture. 1973, 24, 1331−1339. DOI: 10.1002/jsfa.2740241104.
- Dong, X.; Rao, J.; Huber, D.J.; Chang, X.; Xin, F. Wax Composition of ‘Red Fuji’ Apple Fruit during Development and during Storage after 1-Methylcyclopropene Treatment. Horticulture Environment and Biotechnology. 2012, 53, 288−297. DOI: 10.1007/s13580-012-0036-0.
- Raffaele, S.; Vailleau, F.; Léger, A.; Joubès, J.; Miersch, O.; Huard, C.; Blée, E.; Mongrand, S.; Domergue, F.; Roby, D. A MYB Transcription Factor Regulates Very-Long-Chain Fatty Acid Biosynthesis for Activation of the Hypersensitive Cell Death Response in Arabidopsis. Plant Cell. 2008, 20, 752–767. DOI: 10.1105/tpc.107.054858.
- Isaacson, T.; Kosma, D.K.; Matas, A.J.; Buda, G.J.; He, Y.; Yu, B.; Pravitasari, A.; Batteas, J.D.; Stark, R.E.; Jenks, M.A.;; et al. Cutin Deficiency in the Tomato Fruit Cuticle Consistently Affects Resistance to Microbial Infection and Biomechanical Properties, but Not Transpirational Water Loss. Plant Journal. 2009, 60, 363–377. DOI: 10.1111/j.1365-313X.2009.03969.x.
- Peschel, S.; Knoche, M. Studies on Water Transport through the Sweet Cherry Fruit Surface: XII. Variation in Cuticle Properties among Cultivars. Journal of American Society for Horticultural Science. 2012, 137, 367−375.
- Ding, Y.; Chang, J.; Ma, Q.; Chen, L.; Liu, S.; Jin, S.; Han, J.; Xu, R.; Zhu, A.; Guo, J. Network Analysis of Postharvest Senescence Process in Citrus Fruits Revealed by Transcriptomic and Metabolomic Profiling. Plant Physiology. 2015, 168, 357–376. DOI: 10.1104/pp.114.255711.
- Bessire, M.; Chassot, C.; Jacquat, A.C.; Humphry, M.; Borel, S.; Petetot, J.M.; Metraux, J.P.; Nawrath, C. A Permeable Cuticle in Arabidopsis Leads to a Strong Resistance to Botrytis cinerea. Embo Journal. 2007, 26, 2158–2168. DOI: 10.1038/sj.emboj.7601806.
- L’Haridon, F.; Besson-Bard, A.; Binda, M.; Serrano, M.; Abou-Mansour, E.; Balet, F.; Schoonbeek, H.J.; Hess, S.; Mir, R.; Leon, J.;; et al. A Permeable Cuticle Is Associated with the Release of Reactive Oxygen Species and Induction of Innate Immunity. PLoS Pathogens. 2011, 7, e1002148. DOI: 10.1371/journal.ppat.1002148.
- Shepherd, T.; Robertson, G.W.; Griffiths, D.W.; Birch, A.N.E. Effects of Environment on the Composition of Epicuticular Wax Esters from Kale and Swede. Phytochemistry. 1997, 46, 83–96. DOI: 10.1016/S0031-9422(97)00272-0.
- Yang, Y.Q.; Zhou, B.; Zhang, J.; Wang, C.; Liu, C.H.; Liu, Y.L.; Zhu, X.B. Relationships between Cuticular Waxes and Skin Greasiness of Apples during Storage. Postharvest Biology and Technology. 2017, 131, 55–67. DOI: 10.1016/j.postharvbio.2017.05.006.
- Buschhaus, C.; Herz, H.; Jetter, R. Chemical Composition of the Epicuticular and Intracuticular Wax Layers on the Adaxial Side of Ligustrum vulgare Leaves. New Phytologist. 2007, 176, 311–316. DOI: 10.1111/j.1469-8137.2007.02190.x.
- Ensikat, H.J.; Boese, M.; Mader, W.; Barthlott, W.; Koch, K. Crystallinity of Plant Epicuticular Waxes: Electron and X-Ray Diffraction Studies. Chemistry and Physics of Lilips. 2006, 144, 45–59. DOI: 10.1016/j.chemphyslip.2006.06.016.
- Lara, J.M.;. Content, Chemical Composition and Morphology of Epicuticular Wax of Fortune Mandarin Fruits in Relation to Peel Pitting. Journal of the Science of Food and Agriculture. 2000, 80, 1887–1894. DOI: 10.1002/1097-0010(200010)80:13<1887::AID-JSFA730>3.0.CO;2-W.
- López-Castaňeda, J.; Corrales-García, J.; Terrazas-Salgado, T.; Colinas-León, T. Effect of Vapor Heat Treatments on Weight Loss Reduction and Epicuticular Changes in Six Varieties of Cactus Pear Fruit. Journal of Professional Association for Cactus Development. 2010, 12, 37–47.
- Baker, E.A.;. The Influence of Environment on Leaf Wax Development in Brassica oleracea Var. Gemmifera. New Phytologist. 1974, 73, 955–966. DOI: 10.1111/nph.1974.73.issue-5.