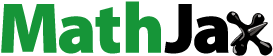
ABSTRACT
The present study investigated the protective effects of the polyphenol epicatechin on the protein structural changes, gel properties and N-nitrosodiethylamine (NDEA) formation of oxidatively stressed myofibrillar protein (MP). Results showed that oxidation treatments to MP significantly increased their carbonyl content and surface hydrophobicity as compared to the control groups (p < 0.05). Epicatechin inhibited protein carbonyl content and surface hydrophobicity, especially treated with 2 mM epicatechin. Under oxidative conditions, the fluorescence intensity of tryptophan gradually decreased with the increase of epicatechin concentrations from 0 to 2 mM. The storage modulus (G’) was increased gradually after oxidation and epicatechin treatment. Epicatechin reduced the loss of P22 and water holding capacity (WHC), promoted the hydration of the proteins. Under oxidative conditions, epicatechin had a concentration dependent effect on the formation of NDEA; low concentrations of epicatechin promoted NDEA formation, while high concentrations inhibited NDEA production. In conclusion, an appropriate quantity of polyphenols can inhibit the oxidation of MP and formation of N-nitrosamines.
Introduction
Meat is a substance that is rich in protein and lipid, and is rather susceptible to oxidation. During events such as meat processing (i.e., storing, cooking and drying), protein oxidation can occur frequently. As a result, the quality of meat products is reduced through phenomena such as the loss of juiciness and reduced cooking yield.[Citation1,Citation2]Therefore, it is of great importance to control protein oxidation and reduce lipid oxidation in meat products.
In the past, synthetic antioxidants have traditionally been used to prevent the oxidation of proteins and lipids in food products. However, recent consumer concerns have been focused on the potential of synthetic antioxidants in being carcinogenic. As a result, interest has developed in the use of natural antioxidants in food products and garnered attention from the scientific community. Among various natural antioxidants, plant-derived antioxidants have been widely used in recent years.[Citation3,Citation4] Antioxidants found in plant extracts are primarily phenolic compounds. These extracts provide promising antioxidant properties through their capacity to regulate intra-cellular hydrogen supplies and metal-ion chelation potentials.[Citation5] Polyphenols have widely been used to inhibit the oxidant modification of meat products. However, while the potential of polyphenols to act as antioxidants in meat products has proven to be effective in delaying lipid oxidation, the same is not true concerning protein oxidation. In some studies, phenolic acids have been found to accelerate carbonylation and sulfhydryl loss. For example, green tea extracts increased thiol loss and protein polymerization in Bologna-type sausages prepared from UV-irradiated pork.[Citation6] Furthermore, beef patties treated with polyphenol-rich willowherb extracts resulted in reduced lipid oxidation, but accelerated protein carbonylation and discoloration.[Citation7] Rosemary and oregano essential oils were found to retard thiol loss, whereas garlic essential oil promoted thiol loss and myosin cross-linking formation in pork patties during chill storage.[Citation8] In protein-rich foods, phenolic substances can interact with proteins through covalent bonds and non-covalent bonds, thereby altering the structure of proteins, leading to functional changes.[Citation9] The oxidation of processed muscle foods was inevitable due to loss of tissue integrity during meat processing and through endogenous prooxidants in fresh muscles. When these phenolic compounds are oxidized, they produce quinones when added to the meat product formulations. Quinones may be associated with the oxidation of proteins during meat processing or cooking.[Citation10]
N-nitrosamines (NAs) are potentially carcinogenic, mutagenic and teratogenic effects to various animals and humans.[Citation11] There are three primary ways of human interaction with NAs: foods, other environmental sources and internal endogenous synthesis. NAs are formed from primary, secondary amines and nitrosating agents in foods such as fish and meat. Therefore, it is essential to reduce or completely inhibit the formation of NAs. The degradation and oxidation of proteins into small molecules may be the reason for the formation of NAs.[Citation12,Citation13]The chemical changes (oxidation, nitrosation and proteolysis) of meat proteins during digestion lead to a decrease in their nutritional value.[Citation14] To clarify the mechanisms of NAs formations, the model system has commonly been used to investigate the different processing parameters or precursors. Although meat proteins are an important reactant in NAs formation, the potential effects of protein oxidation on NAs formation has rarely been reported.
Although there have been some studies on the use of phenolic compounds to inhibit MP oxidation, few reports have studied the effects of phenolic compounds on NAs in the oxidation systems. In general, plant polyphenols include catechins, epicatechin, procyanidins, chlorogenic acid and caffeic acid. Epicatechin alias: 2-(3,4-dihydroxyphenyl)-2,3,4-trihydro-3,5,7-trihydroxychromene, has two hydroxyl groups at the ortho position of the aromatic ring. Epicatechin is a phenolic compound with the potential to protect products against oxidative/nitrative stress. The purpose of this investigation was to study the protective effects of epicatechin on the protein structure characteristics, water distribution of protein gel, and NAs formation.
Materials and methods
Materials
Fresh pork meat from the Longissimus muscle of pig carcasses (24-hours postmortem) was bought from a local supermarket (Suguo, Nanjing, Jiangsu, China). Individual loin chops (weighing~100 g) were packaged and stored in a freezer (−18ºC) until use within one month. In the present study, the chemicals were of reagent grade, and purchased from Aladdin Industrial Corp. or Sigma-Aldrich Co.
MP extraction and sample preparation
MP extraction: MP was prepared according to methods of Park & Xiong[Citation15] and Feng et al.[Citation16]with some modifications. About 400 g of meat was chopped into small sections. The isolation buffer containing 20 mM of sodium phosphate, 0.1 M of NaCl, 2 mM of MgCl2, and 2 mM of EGTA at pH 7.0 was used for the MP extraction. Then 50 g of meat and 200 g of isolation buffer were homogenized using an Ultra Turrax dispersing instrument (T18-Digital, IKA, Staufen, Germany) in an ice bath. After filtration through four layers of gauze, the homogenate was spun twice at 2000 × g for 15 min at 4°C. Subsequently, the pellets were washed twice with 4 L of a 0.1 M NaCl solution. Afterwards, the collected pellets were washed again with 4 L of 20 mM PBS (pH 7.0). The concentration of the obtained protein was measured by the Biuret method.
Oxidative treatments with epicatechin: MP suspensions were prepared with 15 mM piperazine-N,N´-bis (2-ethanesulfonic acid) (PIPES) buffer containing 0.6 M of NaCl at pH 6.25. Six different reaction mixtures (protein concentration, 40 mg/mL) were prepared with epicatechin (0, 0.1, 0.2, 0.5, 1 and 2 mM). Samples with or without epicatechin were oxidized at 4ºC for 12 hours using a hydroxyl radical generation system (10 μM of FeCl3, 100 μM of ascorbic acid, and 1 mM of H2O2).[Citation17] Oxidation was stopped by adding a Trolox C/EDTA mixture (1 mM). A nonoxidized and epicatechin-free MP suspension was used as the control.
Changes of protein side chain structure
Sample carbonyl contents were analyzed using the 2,4-dinitrophenylhydrazine (DNPH) colorimetric method.[Citation18] Briefly, the carbonyl groups were reacted with DNPH to produce protein hydrazones in acidic conditions. The absorbance was measured at 370 nm with an absorption coefficient of 22,000 M−1 cm−1 in order to calculate the carbonyl content.
Total sulfydryl content and free sulfydryl content were measured using absorbance at 412 nm with 5,5ʹ -dithio-bis (2-nitrobenzoic acid) (DTNB) used as an indicator.[Citation19] The total sulfhydryl group was measured with a solution containing urea (8 M urea, 0.086 M Tris, 0.09 M glycine, 4 mM EDTA, pH 8.0), and the free sulfhydryl group was determined using a solution without urea(0.086 M Tris, 0.09 M glycine, 4 mM EDTA, pH 8.0). A molar extinction coefficient of 13,600 M−1cm−1 was used to calculate sulfydryl content.
Surface hydrophobicity was measured using data obtained from absorbance at 595 nm with the bromophenol blue (BPB) binding method.[Citation20] Tryptophan fluorescence was determined using an F-4600 fluorescence spectrophotometer (Hitachi, Japan). MP suspensions (0.1 mg/mL) were excited at 283 nm, with the emission spectra recorded from 300 to 400 nm.
Gelation properties
MP suspensions (5 g, 40 mg/mL in 15 mM PIPES) were transferred into glass vials. These were covered with aluminum foil, heated in a water bath from 20ºC to 80ºC at 1ºC/min increments, and were then maintained at 80ºC for 20 minutes. After heating, the formed gels were immediately chilled in an ice bath for 30 minutes and then the juice was removed from the surface of the gels. Cooking yield was calculated using the following formula, in which Wgel and Wsol are the weight of the gel and the original solution, respectively.
The water holding capacity (WHC) of the samples was measured using the method described by Kocher and Foegeding.[Citation21] Exactly 5 g of gel was placed in a centrifuge tube and centrifuged at 10,000 × g for 10 minutes at 4ºC. Then, WHC was calculated as the percentage of the retained weight of a gel after centrifugation relative to its initial weight.
Gel whiteness was determined using a CR-400 chroma meter (Konica Minolta, Osaka, Japan). Color analyses were performed using the CIE coordinates (L*, a*, and b*). Whiteness value was calculated as:
Dynamic rheological testing was measured during the heat-induced gelation with a model AR1000 rheometer (TA Instruments, West Sussex, U.K.) in an oscillatory mode equipped with parallel plate geometry (40 mm diameter). Gelation was induced by heating the protein emulsion from 20 to 90°C at a rate of 2°C/min. Changes in the storage modulus (G’) were monitored continuously.
Low-field nuclear magnetic resonance (LF-NMR) measurements were performed on a 22.6 MHz NMR analyzer PQ001 (Niumag Co., Ltd., Shanghai, China).[Citation22,Citation23] Transverse relaxation (T2) was measured using the Carr-Purcell-Meiboom-Gill (CPMG) sequence. Each measurement was performed in triplicate. The following parameters: T21, T22, and T23 were presented the relaxation times, P21, P22 and P23 were the corresponding area fractions.
NDEA in an oxidized protein solution
The formation of NDEA was measured on according to the report with slight modifications.[Citation13,Citation24] MP suspensions (10 mg/mL), diethylamine (50 mM) and sodium nitrite (5 mM) were mixed into a 50 mM phosphate buffer (pH 3.0, a simulated gastric acid system) (all concentration was final). Two series reactions were carried out in stoppered conical flasks. The reactions of one group were set for 4 h at 37°C, while the other group was set for 1 h at 80°C. After the reaction, the mixtures were mixed with 5 mM of sulfanilic acid to stop the reaction, and centrifuged at 3,000 × g for 5 min at 4°C. The clear liquid on the upper section of the tube was immediately subjected to HPLC analysis for nitrosamines, targeting NDEA.
An HPLC unit (Agilent 1260, CA, USA) equipped with an Agilent UV detector was employed. Liquid chromatography separation was carried out on a ZORBAX SB-C18 column (150 cm × 4.6 mm, 5 μm) and peaks were detected at 230 nm. An elution program was used with a mixture of 35% methanol and 65% water at a flow rate of 1 mL per minute. A linear relationship was obtained over a range of the concentration between 0.1 and 10.0 μg/mL. The obtained correlation coefficients (R2) were greater than 0.99.
Statistical analyses
The entire experiment was replicated three times on different occasions. Statistical treatments were performed with SPSS version 18.0 (SPSS Inc., Chicago, IL). One way analysis of variance (ANOVA) was applied to assess the significance by means of Duncan’s multiple range tests. The statistical significance was set at p < 0.05. All results were calculated as the means and standard deviations.
Results and discussion
Carbonyl content
Carbonyl levels are a well-known index for the level of protein oxidation in meat products during processing.[Citation2,Citation25] The carbonyl content of the control MP was 1.08 nmol/mg protein () which was close to the report of freshly prepared MP as described by Cao and Xiong.[Citation26] Oxidation significantly increased the carbonyl content of samples (3.63 nmol/mg protein) when compared to the control MP (p < 0.05). The presence of epicatechin significantly inhibited the formation of the carbonyl, especially at a large epicatechin dose of 2 mM (1.14 nmol/mg protein), which was near to the control MP. Epicatechin was oxidized into a quinine derivative in this oxidation system. This study was in accordance with those previous reports in which phenolic compounds inhibited protein carbonyl content, such as quercetin[Citation27],rosmarinic acid[Citation28]and EGCG.[Citation29,Citation30]
Table 1. Physicochemical changes and gel property of myofibrillar protein treated with epicatechin
Total and free sulfydryl groups
MP is rich in sulfydryl groups, which are susceptible to attack by reactive oxygen species and can then convert to intra- and intermolecular disulfide bond linkages.[Citation10] As shown in , the total sulfydryl group content of control MP was 109.63 nmol/mg protein. About 6.6% total sulfydryl group (p > 0.05) was lost when MP samples were exposed to hydroxyl groups. Compared to the control MP, the total sulfydryl group contents were significantly lower in the absence of epicatechin. Furthermore, the total sulfydryl group levels exhibited an epicatechin dose dependent decrease. Compared to the control MP, about 44% total sulfydryl group (p < 0.05) was lost when MP samples were exposed to hydroxyl groups and 2 mM epicatechin. The free sulfydryl group levels in oxidized MP had the same transformation tendency as the total sulfydryl group levels. This could be explained by the formation of thiol-quinone adducts. These observed results are in accordance with previous reports. Cao & Xiong observed that chlorogenic acid had no protection on thiol groups, and high chlorogenic acid revealed the lowest sulfydryl content.[Citation26]
Surface hydrophobicity
It is generally believed that the amount of protein binding BPB can be used as an estimator of protein hydrophobicity estimation. The surface hydrophobicity of the oxidized MP was significantly higher (p < 0.05) than that of the control (). The surface hydrophobicity of the oxidized MP significantly decreased in the presence of EC (p < 0.05), especially in a large dose (2 mM). This decrease of surface hydrophobicity with increased epicatechin may be due to protein aggregation caused by the addition of epicatechin. The decrease was consistent with the change trend of carbonyl content. This study was consistent with other previous reports which indicate that phenolic compounds inhibit the surface hydrophobicity.[Citation29] It is also shown that phenolic compounds can protect protein side-chain groups.
Intrinsic tryptophan fluorescence
As shown in , when compared with the control MP, the intensity of tryptophan fluorescence from oxidized MP was significantly decreased indicating that the protein was denatured. The inclusion of epicatechin promoted the loss of intrinsic fluorescence, especially at the concentration of 2 mM epicatechin. This was consistent with the change seen in surface hydrophobicity, indicating that oxidation leads to further expansion and possible interactions of protein side chains and phenolic compounds. The fluorescence characteristic of tryptophan was particularly sensitive to the polarity of tryptophan microenvironment. It was widely used as a measure index of protein tertiary structure. Similar results were obtained when binding chlorogenic acid to MP[Citation26] or epigallocatechin (EGC) to β-lactoglobulin.[Citation31] In the process of protein oxidation, non-covalent and covalent interactions involving proteins, as well as possible quinone-protein interactions, eventually lead to modifications of proteins in primary, secondary and tertiary structures.
Gel properties
The cooking yield, WHC and whiteness of myofibrillar protein gels are summarized in . Cooking yield was slightly reduced when treated with epicatechin, but this had no significant effect on the cooking yield with or without epicatechin in the hydroxyl radical generating system (p > 0.05). The results of this study may be influenced by the amount of epicatechin addition. In the present study, the amount of epicatechin utilized was low. The WHC of the MP gels increased significantly (p < 0.05) as the epicatechin concentration increased from 0 to 2 mM. This indicates that epicatechin can increase the WHC of the gel, allowing it to be used in meat processing for improving the quality of meat products. The addition of epicatechin at 0.1 mM had no effect on gel whiteness (p > 0.05), while epicatechin from 0.2 to 2 mM reduced gel whiteness (p < 0.05). The reduction in whiteness was possibly due to the chelation of ferric iron and epicatechin oxidation.
The storage modulus (G’) are used to represent the amount of recoverable energy stored in the elastic gel (). The oxidized MP had a final G’ significantly higher than the control, suggesting that oxidation promoted protein interactions. The G’ of the MP emulsion gel treated with 2 mM epicatechin was higher than 0 mM epicatechin, suggesting that epicatechin did not prevent the cross-linking induced by heating under oxidative stress. This was consistent with previous research on MP treated with EGCG.[Citation29]
The LF-NMR technique is an alternative method for assessing water distribution and mobility during protein gel formation. shows the T2 relaxation time distribution of MP gels. The calculated relaxation times, T21, T22 and T23, correspond to the combined water, immobilized water, and free water in the gel, respectively.[Citation32] shows the effect of epicatechin induced modification on the proportions of P21, P22 and P23 of MP gels. The proportions of P21 were not significantly (p > 0.05) affected by oxidation and epicatechin. The oxidation treatment resulted in a lower P22 when compared with the control MP gels (p < 0.05). P22 was slightly increased with increasing epicatechin concentration from 0 to 2 mM, corresponding to the results of WHC in this study. Tea polyphenols may increase the exposure of sensitive side chain groups and enhance the reaction between proteins and phenolic compounds. This hastened the formation of protein gelation, which is retained in the MP network.[Citation33] For P23, the peak area was increased significantly (p < 0.05) by oxidation. The peak area was decreased with increasing epicatechin concentration from 0 to 2 mM (p > 0.05), thus indicating that phenolic compounds can protect the oxidized protein. These findings suggest that oxidation decreased the presence of immobilized water in the gel,while phenolic compounds have a tendency to increase the occurrence of immobilized water. In addition, compared to the oxidized MP, gels with high epicatechin had much higher immobilized water content. This proved that phenolic compounds can be good antioxidants and inhibit protein oxidation, reduce the loss of WHC, and improve the hydration of the proteins.[Citation34]
Table 2. Peaks area proportion of myofibrillar protein gels treated with epicatechin
NDEA analyses
Previous studies have shown that NAs can be formed in cured meat and fish: N-nitrosodimethylamine (NDMA), NDEA and the other NAs.[Citation12,Citation13,Citation35] According to the classification of carcinogenic compounds, NDMA and NDEA fit in the group of probable carcinogens, whereas other NAs fall into the group of possible carcinogens.[Citation36] Previous studies have also shown that only the level of NDEA was high in most samples, which is easy to measure by HPLC.[Citation13,Citation24] Therefore, NDEA was specifically measured in the present study.
The representative chromatogram of NDEA by HPLC was shown in . NDEA can achieve a good symmetrical peak shape under test conditions with good accuracy and precision. The retention time of standard and sample was the same in this study. The levels of NDEA in the stimulated nitrite curing solution are shown in . The content of NDEA in the solution for 4 h at 37°C increased when the epicatechin content increased from 0 to 1 mM, attaining the maximum quantity of NDEA (4.88 µg/mL). However, when epicatechin content was 2 mM, the amount of NDEA production decreased to zero. The content of NDEA in the solution for 1 h at 80°C solution increased when the epicatechin content increased from 0 to 0.5 mM, attaining the maximum quantity of NDEA (10.22 µg/mL). However, when epicatechin content was at 1 or 2 mM, the amount of NDEA production decreased near to zero. Overall, the amount of NDEA formation was first increased to a certain amount, and subsequently rapidly decreased. The formation trend of NDEA was consistent under different temperature treatments. This study also showed that epicatechin concentration higher than 2 mM can completely inhibit the formation of nitrosamines. Choi et al. reported that green tea had a strong inhibitory effect on the nitrosation of secondary amines under gastric conditions.[Citation37] However, since they used green tea at high concentrations, and not at low concentrations, they were not able to observe the promoting effects of green tea on the formation of NAs. Other studies reported both the promoting and inhibiting effects of green tea on NAs formation in vitro, which was similar to that in the present study.[Citation38] From these results, we considered that the promoting and inhibitory effects of epicatechin on the information NDEA might depend on the concentration of epicatechin and nitrite.
Furthermore, Nakamura and Kawabata also studied that the nitrosation reaction could be inhibited by other polyphenols, such as flavonols, flavones and isoflavones.[Citation39] Masuda also investigated the effects of eight chemicals in catechins on the formation of nitrosomorpholine. Resorcinol and phloroglucinol, which have an A-ring model structure, accelerated the formation of nitrosomorpholine at a low concentration, and inhibited it at a high concentration.[Citation38] A conceivable mechanism was for the catalysis of nitrosation by some of phenolic conpounds. Nitroso derivatives may be formed by further reaction with the nitrite to generate a more powerful nitrosating agent, which could be a nitrosoquinone oxime derivative. Its reaction with the nitrosatable substrate produces the nitroso compounds. This mechanism indicates that increasing amounts of catalytically active phenolic compounds lead to higher concentrations of nitroso intermediates. This mechanism could explain the optimum ratio for nitrite to phenolic compound. Accordingly, a large excess of phenolic compounds would inhibit nitrosation, because the nitrosating agent would be completely used up in forming the catalyst agent and none would be left to continue the catalytic reaction. Although we confirmed that the A-ring structure in catechins affected the formation of NAs, the mechanisms have yet to be clearly clarified.
In the present study, the heating temperature at 37°C was representative of gastrointestinal temperature. Meanwhile, the heating temperature at 80°C was representative of the processing for most instant meat products. However, further studies are needed to determine whether high temperatures (100 degrees or higher) could increase the contents of NAs or form other types of NAs.
Correlation coefficients between NDEA and protein oxidation variables
The correlation coefficients between NDEA, protein oxidation variables and gel properties are shown in . NDEA was significantly correlated with free sulfhydryl content, which is consistent with the results reported by Sun.[Citation40] In addition, carbonyl production or total sulfhydryl reduction was significantly correlated (p < 0.01 or p < 0.05) to that of surface hydrophobicity, suggesting that MP fraction oxidation processes might affect each other. WHC and whiteness were extremely and significantly correlated with free and total sulfhydryl content (p < 0.01), suggesting that protein oxidation affects gel properties. Overall, the significant correlation between the content of NDEA and protein oxidation systems when reacted with nitrite suggests that the oxidative formation of carbonyls and oxidative breakage of sulfhydryls with possible deamination contributed to the production of the secondary amine compounds in the manufacture of cured meat.
Table 3. Correlation coefficients between NDEA, protein oxidation variables and gel properties
Conclusion
In the present study, epicatechin influenced oxidation-induced changes through modifying the structure of proteins. This action was demonstrated to be concentration-dependent, in which carbonyl content, sulfydryl content, surface hydrophobicity, and intrinsic tryptophan fluorescence decreased when epicatechin increased from 0 to 2.0 mM. LF-NMR analysis showed that weak hydration of MP induced by oxidation could be improved by the addition of epicatechin. We also demonstrated that the formation of NDEA was inhibited by high epicatechin concentration, and was accelerated by low epicatechin concentration. In conclusion, these findings demonstrate potential benefits of the antioxidant treatments for meat products, such as through reducing the production of unwanted secondary amines by inhibiting the oxidation of proteins.
Acknowledgments
The authors are deeply grateful to the technical support provided by the National Center of Meat Quality and Safety Control of Nanjing Agricultural University.
Disclosure statement
The authors declare no competing financial interest.
Additional information
Funding
References
- Zhou, G.; Zhang, W.; Xu, X. China’s Meat Industry Revolution: Challenges and Opportunities for the Future. Meat Sci. 2012, 92(3), 188–196. DOI: 10.1016/j.meatsci.2012.04.016.
- Zhang, W.; Xiao, S.; Ahn, D. U. Protein Oxidation: Basic Principles and Implications for Meat Quality. Crit. Rev. Food Sci. Nutr. 2013, 53(11), 1191–1201. DOI: 10.1080/10408398.2011.577540.
- Jiang, J.; Xiong, Y. L. Natural Antioxidants as Food and Feed Additives to Promote Health Benefits and Quality of Meat Products: A Review. Meat Sci. 2016, 120, 107–117. DOI: 10.1016/j.meatsci.2016.04.005.
- Li, L.; Shao, J. H.; Zhu, X.; Xu, X.; Zhou, G. Effect of Plant Polyphenols and Ascorbic Acid on Lipid Oxidation, Residual Nitrite and N-Nitrosamines Formation in Dry-Cured Sausage. Int. J. Food Sci. Technol. 2013, 48(6), 1157–1164. DOI: 10.1111/ijfs.12069.
- Zhang, Q.; Rui, X.; Li, W.; Chen, X.; Jiang, M.; Dong, M. Anti-Swarming and -Biofilm Activities of Rose Phenolic Extract during Simulated in Vitro Gastrointestinal Digestion. Food Control. 2016, 64, 189–195. DOI: 10.1016/j.foodcont.2015.12.030.
- Jongberg, S.; Tørngren, M. A.; Gunvig, A.; Skibsted, L. H.; Lund, M. N. Effect of Green Tea or Rosemary Extract on Protein Oxidation in Bologna Type Sausages Prepared from Oxidatively Stressed Pork. Meat Sci. 2013, 93(3), 538–546. DOI: 10.1016/j.meatsci.2012.11.005.
- Cando, D.; Morcuende, D.; Utrera, M.; Estévez, M. Phenolic-Rich Extracts from Willowherb (Epilobium Hirsutum L.) Inhibit Lipid Oxidation but Accelerate Protein Carbonylation and Discoloration of Beef Patties. Eur. Food Res. Technol. 2014, 238(5), 741–751. DOI: 10.1007/s00217-014-2152-9.
- Nieto, G.; Jongberg, S.; Andersen, M. L.; Skibsted, L. H. Thiol Oxidation and Protein Cross-Link Formation during Chill Storage of Pork Patties Added Essential Oil of Oregano, Rosemary, or Garlic. Meat Sci. 2013, 95(2), 177–184. DOI: 10.1016/j.meatsci.2013.05.016.
- Ozdal, T.; Capanoglu, E.; Altay, F. A Review on Protein–Phenolic Interactions and Associated Changes. Food Res. Int. 2013, 51(2), 954–970. DOI: 10.1016/j.foodres.2013.02.009.
- Jongberg, S.; Gislason, N. E.; Lund, M. N.; Skibsted, L. H.; Waterhouse, A. L. Thiol–Quinone Adduct Formation in Myofibrillar Proteins Detected by LC-MS. J. Agric. Food Chem. 2011, 59(13), 6900–6905. DOI: 10.1021/jf200965s.
- Honikel, K. O. The Use and Control of Nitrate and Nitrite for the Processing of Meat Products. Meat Sci. 2008, 78(1–2), 68–76. DOI: 10.1016/j.meatsci.2007.05.030.
- Drabikmarkiewicz, G.; Dejaegher, B.; De, M. E.; Kowalska, T.; Paelinck, H.; Vander, H. Y. Influence of Putrescine, Cadaverine, Spermidine or Spermine on the Formation of N-Nitrosamine in Heated Cured Pork Meat. Food Chem. 2011, 126(4), 1539–1545. DOI: 10.1016/j.foodchem.2010.11.149.
- Yang, H.; Meng, P.; Xiong, Y. L.; Ma, L.; Wang, C.; Zhu, Y. Oxidation in HiOx-packaged Pork Longissimus Muscle Predisposes Myofibrillar and Sarcoplasmic Proteins to N-Nitrosamine Formation in Nitrite-Curing Solution. Meat Sci. 2013, 95(3), 465–471. DOI: 10.1016/j.meatsci.2013.05.038.
- Pomélie, D. D. L.; Santélhoutellier, V.; Sayd, T.; Gatellier, P. Oxidation and Nitrosation of Meat Proteins under Gastro-Intestinal Conditions: Consequences in Terms of Nutritional and Health Values of Meat. Food Chem. 2018, 243, 295–304. DOI: 10.1016/j.foodchem.2017.09.135.
- Park, D.; Xiong, Y. L. Oxidative Modification of Amino Acids in Porcine Myofibrillar Protein Isolates Exposed to Three Oxidizing Systems. Food Chem. 2007, 103(2), 607–616. DOI: 10.1016/j.foodchem.2006.09.004.
- Feng, X.; Li, C.; Ullah, N.; Hackman, R. M.; Chen, L.; Zhou, G. Potential Biomarker of Myofibrillar Protein Oxidation in Raw and Cooked Ham: 3-Nitrotyrosine Formed by Nitrosation. J. Agric. Food Chem. 2015, 63(51), 10957–10964. DOI: 10.1021/acs.jafc.5b04107.
- Xia, T.; Zhao, X.; Yu, X.; Li, L.; Zhou, G.; Han, M.; Xu, X. Negative Impacts of In-Vitro Oxidative Stress on the Quality of Heat-Induced Myofibrillar Protein Gelation during Refrigeration. Int. J. Food Prop. 2018, 21(1), 2205–2217. DOI: 10.1080/10942912.2018.1505754.
- Oliver, C. N.; Ahn, B. W.; Moerman, E. J.; Goldstein, S.; Stadtman, E. R. Age-Related Changes in Oxidized Proteins. J. Biol. Chem. 1987, 262(12), 5488–5491.
- Liu, G.; Xiong, Y. L.; Butterfield, D. A. Chemical, Physical, and Gel-Forming Properties of Oxidized Myofibrils and Whey‐And Soy‐Protein Isolates. J. Food Sci. 2000, 65(5), 811–818. DOI: 10.1111/j.1365-2621.2000.tb13592.x.
- Chelh, I.; Gatellier, P.; Santé-Lhoutellier, V. Technical Note: A Simplified Procedure for Myofibril Hydrophobicity Determination. Meat Sci. 2006, 74, 681–683. DOI: 10.1016/j.meatsci.2006.05.019.
- Kocher, P. N.; Foegeding, E. A. Microcentrifuge-Based Method for Measuring Water-Holding of Protein Gels. J. Food Sci. 1993, 58(5), 1040–1046. DOI: 10.1111/j.1365-2621.1993.tb06107.x.
- Liu, J. G.; Liu, D. M.; Yao, Y. B.; Wu, J. G.; Li, J. Q. Application of Low-Field Nuclear Magnetic Resonance (LFNMR) in Characterizing Coal Pores and Permeability. Adv. Mater. Res. 2013, 718–720. DOI: 10.4028/www.scientific.net/AMR.718-720.1012.
- Ji, H.; Dong, S.; Han, F.; Li, Y.; Chen, G.; Li, L.; Chen, Y. Effects of Dielectric Barrier Discharge (DBD) Cold Plasma Treatment on Physicochemical and Functional Properties of Peanut Protein. Food Bioprocess Technol. 2018, 11(2), 344–354. DOI: 10.1007/s11947-017-2015-z.
- Li, L.; Zhang, C.; Zhou, G.; Xu, X. Effects of Polyphenols on the Nitrite Scavenging and N-Nitrosamine Formation in Simulated Gastric Acid. J. Nanjing Agric. Univ. 2013, 36(3), 111–116. DOI: 10.7685/j.issn.1000-2030.2013.03.019.
- Estévez, M. Protein Carbonyls in Meat Systems: A Review. Meat Sci. 2011, 89(3), 259–279. DOI: 10.1016/j.meatsci.2011.04.025.
- Cao, Y.; Xiong, Y. L. Chlorogenic Acid-Mediated Gel Formation of Oxidatively Stressed Myofibrillar Protein. Food Chem. 2015, 180, 235–243. DOI: 10.1016/j.foodchem.2015.02.036.
- Utrera, M.; Estévez, M. Impact of Trolox, Quercetin, Genistein and Gallic Acid on the Oxidative Damage to Myofibrillar Proteins: The Carbonylation Pathway. Food Chem. 2013, 141(4), 4000–4009. DOI: 10.1016/j.foodchem.2013.06.107.
- Wang, S.; Zhang, Y.; Chen, L.; Xu, L.; Zhou, G.; Li, Z.; Feng, X. Dose-Dependent Effects of Rosmarinic Acid on Formation of Oxidatively Stressed Myofibrillar Protein Emulsion Gel at Different NaCl Concentrations. Food Chem. 2018, 243, 50–57. DOI: 10.1016/j.foodchem.2017.09.114.
- Feng, X.; Chen, L.; Lei, N.; Wang, S.; Xu, X.; Zhou, G.; Li, Z. Emulsifying Properties of Oxidatively Stressed Myofibrillar Protein Emulsion Gels Prepared with (−)-Epigalloepicatechin-3-Gallate and NaCl. J. Agric. Food Chem. 2017, 65(13), 2816–2826. DOI: 10.1021/acs.jafc.6b05517.
- Cao, Y.; Ai, N.; True, A. D.; Xiong, Y. L. Effects of (−)-Epigallocatechin-3-Gallate Incorporation on the Physicochemical and Oxidative Stability of Myofibrillar Protein–Soybean Oil Emulsions. Food Chem. 2018, 245, 439–445. DOI: 10.1016/j.foodchem.2017.10.111.
- Wu, X.; Wu, H.; Liu, M.; Liu, Z.; Xu, H.; Lai, F. Analysis of Binding Interaction between (−)-Epigallocatechin (EGC) and β-lactoglobulin by Multi-Spectroscopic Method. Spectrochim. Acta, Part A: Mol. Biomol. Spectrosc. 2011, 1, 164–168. DOI: 10.1016/j.saa.2011.07.028.
- Han, M.; Wang, P.; Xu, X.; Zhou, G. Low-Field Nmr Study of Heat-Induced Gelation of Pork Myofibrillar Proteins and Its Relationship with Microstructural Characteristics. Food Res. Int. 2014, 62, 1175–1182. DOI: 10.1016/j.foodres.2014.05.062.
- Wang, L.; Zhang, M.; Bhandari, B.; Gao, Z. Effects of Malondialdehyde-Induced Protein Modification on Water Functionality and Physicochemical State of Fish Myofibrillar Protein Gel. Food Res. Int. 2016, 86, 131–139. DOI: 10.1016/j.foodres.2016.06.007.
- Niu, H.; Chen, Y.; Zhang, H.; Kong, B.; Liu, Q. Protective Effect of Porcine Plasma Protein Hydrolysates on the Gelation of Porcine Myofibrillar Protein Exposed to a Hydroxyl Radical-Generating System. Int. J. Biol. Macromol. 2018, 107, 654–661. DOI: 10.1016/j.ijbiomac.2017.09.036.
- Li, L.; Wang, P.; Xu, X.; Zhou, G. Influence of Various Cooking Methods on the Concentrations of Volatile N-Nitrosamines and Biogenic Amines in Dry-Cured Sausages. J. Food Sci. 2012, 77(5), C560–565. DOI: 10.1111/j.1750-3841.2012.02667.x.
- Crews, C. The Determination of N- Nitrosamines in Food. Qual. Assur. Saf. Crops Food. 2010, 2(1), 2–12. DOI: 10.1111/j.1757-837X.2010.00049.x.
- Choi, S. Y.; Chung, M. J.; Sung, N. J. Volatile N-Nitrosamine Inhibition after Intake Korean Green Tea and Maesil (Prunus Mume SIEB. Et ZACC.) Extracts with an Amine-Rich Diet in Subjects Ingesting Nitrate. Food Chem. Toxicol. 2002, 40(7), 949–957. DOI: 10.1016/S0278-6915(02)00025-X.
- Masuda, S.; Uchida, S.; Terashima, Y.; Kuramoto, H.; Serizawa, M.; Deguchi, Y.; Yanai, K.; Sugiyama, C.; Oguni, I.; Kinae, N. Effect of Greeen Tea on the Formation of Nitrosamines,And Cancer Mortality. J. Health Sci. 2006, 52(3), 211–220. DOI: 10.1248/jhs.52.211.
- Nakamura, M.; Kawabata, T. Effect of Japanese Green Tea Oh Nitrosamine Formation in Vitro. J. Food Sci. 2010, 46(1), 306–307. DOI: 10.1111/j.1365-2621.1981.tb14592.x.
- Sun, W.; Meng, P.; Ma, L. Relationship between N-Nitrosodiethylamine Formation and Protein Oxidation in Pork Protein Extracts. Eur. Food Res. Technol. 2014, 239(4), 679–686. DOI: 10.1007/s00217-014-2264-2.