ABSTRACT
Tree peony seeds oil (TPSO, Paeonia ostii) extracted at different roasting temperatures were studied for color, acid values, total phenols, fatty acid composition, antioxidant capacity, volatiles, and benzoic acid. Roasting led to increased antioxidant capacity and acid values. Headspace analysis of raw peony seed oil showed only six volatile compounds, while after roasting volatiles such as pyrazines, aldehydes and furans were observed and contributed to nutty aromas. Unsaturated fatty acids constituted more than 90% of the total fatty acids with α-linolenic acid as the predominant fatty acid of TPSO and was not significantly changed with roasting temperature. Benzoic acid concentration increased significantly as roasting temperature increased. Although the occurrence of benzoic acid in virgin oil might be a concern, it can be removed through refining for commercial production.
Introduction
Tree peony (Paeonia ostii) is an economically important ornamental plant native to China.[Citation1] It is called the “the king of flowers” in China due to its being a cultural symbol of peace and happiness.[Citation2] Tree peony cultivation exceeds 20,000 hectares which seed yields over 57,000 tonnes in China.[Citation3] It is also a potential source of oil (>27% yield) that is high in unsaturated fatty acids (>90%).[Citation4] P. ostii is one of two cultivars used for oil production in eastern and southern China.[Citation5] The National Health and Family Planning Commission of the People’s Republic of China (2011) has authorized this oil to claim to be a new resource food. Recent research focused on the fatty acids composition, phenolic compounds and antioxidant activity of tree peony seeds.[Citation6,Citation7]
Roasting plays an important role in the pretreatments of oil seeds before oil extraction. Complex reactions such as the Maillard reaction, Strecker degradation, caramelization, and lipid oxidation lead to beneficial aromatic compounds.[Citation8] For example, Park et al.[Citation9] showed that roasting perilla seeds oil led to desirable headspace volatiles. They suggested roasting prior to sale would be beneficial. Roasting also provided strong roasty and nutty aromas to peanut oil, mainly because of the pyrazines generated by the Maillard reactions.[Citation10] Roasting also made the oil more resistant to oxidative deterioration.[Citation11]
Benzoic acid has been used as a food preservative and is found in cheese, sour cream, yoghurt, and soy sauce[Citation12,Citation13], and in many fruits, vegetables, and nuts at low concentration.[Citation14] According to the Chinese Hygienic Standard for Uses of Food Additives[Citation15], the upper concentration of benzoic acid allowed in food is 2.0 g/kg. The maximum concentration of benzoic acid in foods in the USA is 1 g/kg.[Citation16] It is a colorless volatile aromatic compound.[Citation14,Citation17] Roasted tree peony seeds have not been previously studied. Thus, the aim of this study was to characterize its color, acid values, free fatty acids, antioxidant activity, volatile compounds, and benzoic acid concentrations.
Materials and methods
Materials
Fresh tree peony seeds (P. ostii) (~10 kg) used in this study were obtained from Beijing Forestry University Forestry Experiment Station (Beijing, China) in August 2016. They were oven-dried at 35°C for 12 h, and then the outer black hulls were removed manually.
2,2-Diphenyl-1-picrylhydrazyl (DPPH), 2,2ʹazino-bis-(3-ethylbenzthiazoline-6-sulfonic acid) (ABTS) and gallic acid were purchased from Sigma-Aldrich (St. Louis, MO, USA); benzoic acid, 1,2,3-trichloropropane, and trolox were purchased from AccuStandard Inc. (New Haven, CT, USA). Ammonium acetate (chromatography grade) was bought from Anpel Laboratory Technologies Inc. (Shanghai, China). The authentic reference compounds 2-ethyl-6-methylpyrazine, eugenol, (Z)-hex-3-en-1-ol, benzaldehyde, furfural, hexanoic acid, and acetic acid were purchased from Sigma-Aldrich. HPLC-grade methanol was purchased from Mreda Technology Inc. (Beijing, China). Other chemicals were analytical grade and obtained from Tianjin Yongda Chemical Reagent Co. (Tianjin, China).
Tree peony seeds, roasting and oil extraction
De-hulled tree peony seeds (~200 g) on aluminum foil were oven-roasted (T3-L383b, Media Kitchen 98, Appliance Manufacturing Co., Ltd., Foshan, Guangdong, China) at 120°C, 130°C, 140°C, and 150°C, respectively, for 15 min. The unroasted and roasted seeds were put into a single-setting lab-scale oil press (YKY-6YL-550, Longyan Machinery Manufacturing Co., Ltd., Longyan, Fujian, China). The crude oil was centrifuged (HC-2518R, Zonkla Scientific Instrument Co., Ltd., Hefei, Anhui, China) at 2200 × g for 15 min and stored at −20°C in brown bottles in the dark for a maximum of 8 wk.
Color, acid value and fatty acid composition
The color was determined at room temperature (20°C) using the DTQC-10A Chromameter with a 4-mm-diameter viewing aperture (Beijing Oriental Precision Technology Co., Beijing, China). The three CIE coordinates L* (lightness), a* (green-red) and b* (blue-yellow) were measured. The colorimeter was re-calibrated using a standard white plate after each measurement. Acid value (AV) was measured according to AOCS Official Method Cd 3d-63.[Citation18]
Fatty acid methyl esters of oil samples were prepared according to the method of Ning et al..[Citation19] Aliquots of the methylated samples were auto injected and analysed using an Agilent Technologies 6890 GC gas chromatograph (Agilent Technologies, Palo Alto, CA, USA) equipped with a split/splitless injection port and a flame ionization detector and a Cd-Acidwax capillary column (30 m × 0.25 mm ×25 µm, Anpel Laboratory Technologies Inc.). The injection volume was 1 µL, and the split ratio was 30:1 with nitrogen as the carrier gas (≥99.999% purity, Beijing Ruyuanruquan Technology Co., Ltd., Beijing, China) at a flow rate of 1 mL/min. The detector and injector temperatures were 250°C and 280°C, respectively. The operation conditions were: initial oven temperature 150°C, then increased at 8°C/min to 190°C and held for 3 min, then 10°C/min to 230°C and held for 10 min. The fatty acids were identified by comparing the retention times with a reference mixture of fatty acid methyl ester standards from Sigma-Aldrich, and peak areas were determined using the computer provided with the instrument. The percentage of each fatty acid was determined by taking each observed peak area as the percent of the total peak area.
Methanol extracts
Methanol extracts of tree peony seeds oil (TPSO) were prepared according to the methods of Durmaz et al.[Citation11] with minor modifications. Briefly, 1 g of oil and 2 mL of methanol were mixed and agitated for 1 min using a Vortex mixer (QL-901, Haimen Kylin-Bell Lab Instruments Co., Ltd., Nantong, Jiangsu, China). After centrifugation at 6000 × g for 5 min at room temperature, the upper methanolic phase was removed and the residue was re-extracted two times. The supernatants were combined. The final methanolic solutions were used for analysis of the phenol and antioxidant capacity.
Antioxidant capacity and phenols
DPPH and ABTS assays[Citation20] were used to determine the total antioxidant capacity of oil samples. The results of both tests were expressed as µg trolox equivalent/mL methanolic extract based on a calibration curve prepared using trolox assuming 100% purity. Total phenol content was measured using the Folin and Ciocalteu method[Citation21] and the results were expressed as µg gallic acid equivalents/mL of methanolic extracts of the oil samples based on a calibration curve prepared using gallic acid assuming 100% purity.
Extraction of volatile compounds
Two g oil samples were weighed and combined with 10 µL of 1,2,3-trichloropropane (1.0 mg/mL in methanol) used as an internal standard (IS) as it has not previously been found in any peony products. The 15 mL vial was heated on a hot plate (laboratory stirrer/hotplate PC-620D, Corning Inc., Corning, NY, USA) at 60°C for 20 min. A 1 cm long and 65 µm diameter polydimethylsiloxane/divinylbenzene fiber (Supelco, Bellefonte, PA, USA) was inserted into the headspace for 30 min to absorb volatile compounds. The fiber was then transferred to the injection port and desorbed at 250°C for 5 min.
Gas chromatography-mass spectrometry (GC-MS)
The analysis was done using a polar capillary HP-INNOWAX column (60 m × 0.25 mm × 0.25 µm, J &W Scientific, Folsom, CA, USA) connected to an Agilent 6890 GC and a nonpolar Rtx-5MS column (30 m × 0.25 mm × 0.25 µm, Restek, Bellafonte, PA, USA) connected to a QP2010 GC-MS system (Shimadzu, Kyoto, Japan). The operating conditions for the Agilent system were: injection port temperature was 250°C, and the injection mode was splitless; purified nitrogen (≥99.999%, Beijing Ruyuanruquan Technology Co., Ltd., Beijing, China) was used as the carrier gas with a flow rate of 1 mL/min; the oven temperature program was 1 min at 50°C, then 3°C/min up to 220°C and held for 5 min; mass range, m/z = 20–350. The operating conditions for the Shimadzu system were: injection port temperature was 250°C in a split mode of 1:10; the initial oven temperature was 45°C for 10 min, then 3°C/min up to 100°C and held for 1 min, 5°C/min up to 150°C and held for 5 min, then 10°C/min up to 250°C with a 10-min final hold. Helium gas (≥99.999%, Beijing AP BAIF Gases Industry Co., Ltd., Beijing, China) was used as the carrier gas with a flow rate of 1 mL/min. All mass spectra were obtained at an ionization energy of 70 eV and 230°C ion source temperature.
Identification and semi-quantification of volatile compounds
Identification of volatile compounds was done using a combination of the NIST (National Institute of Standards and Technology) mass spectrum library and the gas chromatographic retention times of authentic standard compounds. In addition, the volatiles were identified by comparison with retention indices (RI) from the literature as collected by the laboratory where this part of the work was also done. The RI was calculated using n-alkanes (C7-C24) under the same conditions and appropriate calculations (Liu et al., 2011). Approximate relative concentrations were calculated by comparing peak areas of the identified volatile compounds with the peak area of the internal standard.
Determination of benzoic acid using HPLC
The extraction of benzoic acid used the Chinese National Standard Method GB 5009.28 (Ministry of Health of the People’s Republic of China and the Standardization Administration of the People’s Republic of China, 2016).[Citation22] Approximately 2 g oil were weighed and diluted with 10 mL n-hexane and heated in a 60°C water bath for 5 min. Then, 25 mL aqueous ammonia (1:99, v/v) and 1 mL absolute ethanol added and vigorously agitated. Samples were then sonicated (JAC 600, Aobo Co. Ltd., Jining, Shandong, China) at 50°C for 20 min. After the mixture cooled to room temperature, 2 mL each of 0.22 mol/L potassium ferrocyanide and 1 mol/L zinc acetate solutions were added and then centrifuged at 6000 × g for 5 min. The water phase was collected and the residues were re-extracted. The combined water phases were brought to 50 mL in a volumetric flask, then filtered using a 0.22 µm membrane filter (Jinteng Laboratory Equipment Co., Ltd., Tianjin, China). Samples were injected into a high-performance liquid chromatograph (Shimadzu LC-2010A HT) coupled with a C18 column (250 mm × 4.6 mm i.d., 5 µm; Bonna-Agela Technologies Inc., Wilmington, DE, USA) at a flow rate of 1 mL/min. The mobile phase was a 5:95 v/v mixture of methanol and 20 mmol/L ammonium acetate buffer. The injection volume was 10 µL, and the benzoic acid peak was detected at a wavelength of 230 nm (2016, GB 5009.28). The retention time of an authentic benzoic acid sample was obtained, and a calibration curve was prepared using the authentic standard assuming 100% purity.
Statistical analysis
The mean values and standard deviations of triplicate measurements are reported. Data processing was done using the SPSS 22.0 version (SPSS Inc., Chicago, IL, USA) and Microsoft Excel 2016 (Microsoft Corp., Redmond, WA, USA) software. Figures were drawn using Origin 8.0 software (OriginLab Corp., Northampton, England). Significant differences using Duncan’s multiple range test were accepted when the p< 0.05.
Results and discussion
Color
L*, a*, and b* color values are given in . Roasting led to decreased L* values and no significant changes (p ≥ 0.05) in a* and b* values from control. The L* decrease from control was greatest at 130°C (p < 0.05) and all samples were significantly darker than the control (p< 0.05). The decrease in L* was similar to that for oils from raw and roasted pistachios.[Citation23] The darkening is probably due to chemical reactions such as caramelization and Maillard reactions.[Citation9]
Table 1. Physiochemical characteristics of TPSO with different roasting temperatures
Acid values
According to the Hygienic Standard for Edible Vegetable Oil of the People’s Republic of China (Ministry of Health of the People’s Republic of China and Standardization Administration of the People’s Republic of China, 2005)[Citation24], edible oils with acid values (i.e., free fatty acids) below 3 mg KOH/g oil are considered acceptable. If higher, then the oil needs to be refined. shows the acid values. Generally, acid values were significantly higher (p < 0.05) at each temperature compared to the prior temperature indicating that heating led to more free fatty acids but was still acceptable at 150°C. However, these values were lower than those in melon seeds oil (1.2–4 mg KOH/g).[Citation25]
Fatty acids composition
Fatty acids compositions are shown in . The five major fatty acids all remained essentially unchanged (p ≥ 0.05) with the three major unsaturated acids accounting for more than 90% of the total fatty acids composition, which agrees with previous work with unroasted peony seeds oil.[Citation7] Similarly, the fatty acids compositions of safflower oils were not significantly affected by roasting temperatures.[Citation26] TPSO with its high level of α-linolenic acid, which is an essential fatty acid, may allow it to be marketed as a nutraceutical.
Antioxidant capacity and phenolic content
The results of the antioxidant capacity and phenolic content are summarized in . Roasting resulted in an increased antioxidant capacity with both the DPPH and ABTS assays. DPPH scavenging increased significantly (p< 0.05) at each temperature compared to the prior temperature. ABTS also increased although some increased temperatures were not significantly different (p ≥ 0.05) from the prior temperature. Increased radical scavenging could be explained by the possible formation of reducing compounds. Such compounds have been identified in other oils as being intermediates in Maillard reactions.[Citation27] Ling et al. found that the antioxidant activity of pistachio kernels (Pistacia vera L. Var Kerman) oil measured using the ABTS assay also increased after roasting.[Citation23]
Phenolic content decreased significantly (p< 0.05) with roasting. The three lower roasting temperatures had a significant (p< 0.05) greater loss of phenolics compared to both the control and the sample at 150°C. Natural phenolic compounds can be degraded with high temperature, while some antioxidative Maillard reaction products may be generated during roasting which may increase the phenolic content.[Citation27] However, phenolic content was increased through the roasting process. This is probably caused by the relatively polar compounds that accumulated during roasting. Phenolic compounds were reported to release from bound structures at higher temperatures and passed to the oil phase better if the oil was extracted from roasted seeds.[Citation11]
Volatile compounds of roasted and unroasted TPSO
A total of 42 volatile compounds were identified in unroasted and/or roasted TPSO samples. Changes in relative concentrations of volatiles with roasting temperature are given in . Volatiles included pyrazines, alcohols, phenols, furans, aldehydes, ketones, acids, alkenes, and other compounds. Overall, the profiles for many of these volatiles changed significantly (p < 0.05) with roasting. Some volatiles were generated during roasting and increased with temperature such as some pyrazines, aldehydes, and acids. A few of these compounds will be discussed below.
Table 2. Volatile compounds of TPSO with different roasting temperatures
Most pyrazines were first detected at 130°C while a few others were first detected at 140°C. Previous research indicated that pyrazines contributed to roasted, toasted, nutty and burnt aromas.[Citation28] Among the identified pyrazines, 2,6-dimethylpyrazine increased significantly (p< 0.05) at each temperature and was the most dominant pyrazine. The odor thresholds of 2-methylpyrazine, 2,6-dimethylpyrazine and trimethylpyrazine have been reported to be 27, 8 and 27 ppm/oil, respectively.[Citation10] Therefore, only the 2,6-dimethylpyrazine at 150°C was above its odor threshold and could then add additional roasted and nutty aromas. Pyrazines are usually generated during Strecker degradation, and the formation of pyrazine compounds was probably related to the carbonyl-amine condensation of two amino ketones.[Citation8] Furthermore, earlier research also suggested that most pyrazines were generated when thermal processing temperatures were above 100°C.[Citation8]
Four furan derivatives were found in roasted TPSO. The occurrence of furan derivatives has been reported in roasted almonds, pumpkin seeds, and peanuts oils.[Citation10,Citation29,Citation30] Lipid peroxidation and degradation of carbohydrates are two possible pathways.[Citation30] Moreover, 4-hydroxy-2,5-dimethyl-3(2H)-furanone was first found at 130°C and increased significantly (p < 0.05) at each higher temperature. Earlier research also reported that this compound was detected in roasted sesame seeds and contributed a sweet aroma to roasted almonds.[Citation29,Citation31]
Aldehydes and ketones were thermally induced. As can be seen from , most aldehydes were detected at 130°C and showed a significant (p < 0.05) increasing trend with further heating. Phenylacetaldehyde, which was identified at 120°C has been reported to have a honey-like aroma with roasted cocoa beans.[Citation32] Benzaldehyde, an aromatic aldehyde with an almond-like flavor[Citation33], increased significantly (p < 0.05) at each roasting temperature. Furfural, a furan-containing aldehyde, has been reported during thermal degradation of sugars like fructose and glucose.[Citation33]
Ketones were abundant in the roasted TPSO with 2,3-dihydro-3,5-dihydroxy-6-methyl-4(H)-pyran-4-one and 3-hydroxy-2-methyl-4H-pyran-4-one being the main ketones and both increased significantly (p < 0.05) at each temperature. The increases might be caused by lipid oxidation during thermal processing. Hydroxyacetone, 2,3-pentadione and acetoin were only found at and above 130°C.
Some alcohols, phenols, and acids were also detected in roasted TPSO samples. Alcohols like (Z)-hex-3-en-1-ol, hexan-1-ol and 1-octen-3-ol were only presented in oil samples at 120°C and were lost at higher temperatures. Aliphatic alcohols like 1-octen-3-ol have been reported to be generated by the thermal decomposition of methyl linoleate hydroperoxide through lipid oxidation.[Citation33] However, the aliphatic alcohols have relatively high odor threshold values and are therefore presumed to not contribute to the aroma of the roasted oils.[Citation10] Eugenol, phenol and methyl eugenol were found at low relative concentrations in roasted oil samples.
Benzoic acid increased significantly (p< 0.05) with each roasting temperature. Benzoic acid has also been found in roasted palm kernel oil.[Citation8] Acetic acid also increased significantly (p < 0.05) at each temperature. It was reported as the most abundant acid in palm kernel oil and is generated early in the Maillard reactions.[Citation8]
Alkenes including α-pinene and β-myrcene both disappeared above 120°C. The relative concentration of α-pinene at 120°C decreased when compared to unroasted oil samples. Kiralan reported that α-pinene in black cumin oils was significantly (p < 0.05) reduced with microwave heating for >8 min.[Citation34]
Toluene, an aromatic hydrocarbon, was only detected in unroasted oil samples. The results suggest that the formation of Maillard reaction products needs a minimum reaction temperature of 130°C when roasting for 15 min. In most cases, the higher roasting temperatures led to more volatile compounds.
Determination of benzoic acid in TPSO
Total ion chromatograms of volatiles obtained from unroasted and roasted oil samples using a polar and a non-polar column are shown in . The peak areas of benzoic acid in both columns increased as temperature increased. Quantitation was done using an HPLC system. As shown in , the benzoic acid standard peak had a retention time of 7.019 min, while that of the oils was 7.148 min. shows the benzoic acid concentration increased significantly (p < 0.05) at each roasting temperature. All samples met the requirements of the US FDA and the Chinese government. This is the first report of benzoic acid in roasted TPSO.
Table 3. Benzoic acid content of TPSO roasted at different temperatures
Figure 1. Total ion chromatograms of the volatiles formed with different roasting temperatures of TPSO. a. with HP-Innowax column. b. with Rtx-5MS column
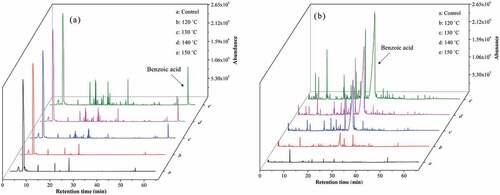
Figure 2. Typical chromatogram of benzoic acid standard at a concentration of 50 mg/L a. and oil sample roasted at 150°C b. The detector wavelength were both set at 230 nm
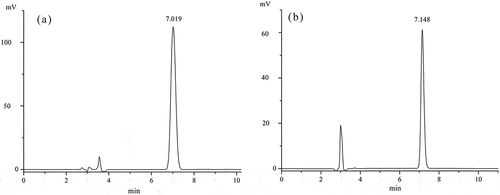
To date, there is no report of benzoic acid in unroasted or roasted tree peony seeds oil. A possible metabolic pathway to explain the generation of benzoic acid is the degradation of phenylalanine.[Citation14] The formation of benzoic acid may be related to the breakdown during heating of the phenolic compound paeoniflorin, a non-volatile compound that would not have been detected in the current study. Mao, et al. found paeoniflorin had a concentration of 23.7 mg/g in tree peony seeds.[Citation7] Another possibility is aquathermolysis of benzaldehyde to benzoic acid.[Citation35] Further study needs to be done to determine the formation of benzoic acid in roasted TPSO.
Conclusion
This study showed that virgin oil extracted from raw and roasted tree peony seeds was abundant in α-linolenic acid. With increased roasting temperatures, the oil became darker and acid values increased significantly while phenol content fluctuated. Antioxidant activity increased significantly with temperature. Roasting prior to oil extraction led to more volatiles such as pyrazines, furans, and aldehydes which contributed pleasant roasty and nutty aromas to the oil, and these volatiles generally increased with temperature. Although the presence of benzoic acid might be a concern, all samples were within regulatory limits. Furthermore, benzoic acid could be removed by refining if necessary. Thus, these results suggest that further work to develop TPSO commercially is justified.
Acknowledgments
This paper was supported by Beijing Municipal Science & Technology Project (Z181100009318012). Thanks for the kind help during the operation of the HS-SPME-GC-MS by Prof. Zhu Baoqing and his laboratory in the Department of Food Science and Engineering, Beijing Forestry University. The authors have no conflicts of interest with respect to this manuscript.
Additional information
Funding
References
- Wu, J.; Cheng, F.-Y.; Cai, C. F.; Zhong, Y.; Jie, X. Association Mapping for Floral Traits in Cultivated Paeonia Rockii Based on SSR Markers. Mol. Genet. Genomics. 2016, 292, 187–200. DOI: 10.1007/s00438-016-1266-0.
- Xu, -X.-X.; Cheng, F.-Y.; Xian, H.-L.; Peng, L.-P. Genetic Diversity and Population Structure of Endangered Endemic Paeonia Jishanensis, in China and Conservation Implications. Biochem. Syst. Ecol. 2016, 66, 319–325. DOI: 10.1016/j.bse.2016.05.003.
- Li, -S.-S.; Yuan, R.-Y.; Chen, L.-G.; Wang, L.-S.; Hao, X.-H.; Wang, L.-J.; Zheng, X.-C.; Du, H. Systematic Qualitative and Quantitative Assessment of Fatty Acids in the Seeds of 60 Tree Peony (Paeonia Section Moutan DC.) Cultivars by GC-MS. Food Chem. 2015, 173, 133–140. DOI: 10.1016/j.foodchem.2014.10.017.
- Gao, -L.-L.; Li, Y.-Q.; Wang, Z.-S.; Sun, G.-J.; Mo, H.-Z. Physicochemical Characteristics and Functionality of Tree Peony (Paeonia Suf- Fruticosa Andr.) Seed Protein. Food Chem. 2018, 240, 980–988. DOI: 10.1016/j.foodchem.2017.07.124.
- Cui, H.-L.; Cheng, F.-Y.; Peng, L.-P. Determination of the Fatty Acid Composition in Tree Peony Seeds Using Near-Infrared Spectroscopy. J. Am. Chem. Soc. 2016, 93, 943–952.
- Zhang, -X.-X.; Shi, -Q.-Q.; Ji, D.; Niu, L.-X.; Zhang, Y.-L. Determination of the Phenolic Content, Profile, and Antioxidant Activity of Seeds from Nine Tree Peony (Paeonia Section Moutan DC.) Species Native to China. Food Res. Int. 2017, 97, 141–148. DOI: 10.1016/j.foodres.2017.03.018.
- Mao, Y.-M.; Han, J.-H.; Tian, F.; Tang, X.; Hu, Y.-H.; Guan, Y. Chemical Composition Analysis, Sensory, and Feasibility Study of Tree Peony Seed. J. Food Sci. 2017, 82, 553–561. DOI: 10.1111/1750-3841.13593.
- Zhang, W.-Z.; Wang, R.; Yuan, Y.-H.; Yang, T.-K.; Liu, S.-Q. Changes in Volatiles of Palm Kernel Oil before and after Kernel Roasting. LWT - Food Sci. Technol. 2016, 73, 432–441. DOI: 10.1016/j.lwt.2016.06.051.
- Park, M. H.; Seol, N. G.; Chang, P.-S.; Yoon, S. H.; Lee, J. H. Effects of Roasting Conditions on the Physicochemical Properties and Volatile Distribution in Perilla Oils (Perilla Frutescens Var. Japonica). J. Food Sci. 2011, 76, C808–816. DOI: 10.1111/j.1750-3841.2011.02214.x.
- Liu, X.-Y.; Jin, Q.-Z.; Liu, Y.-F.; Huang, J.-H.; Wang, X.-G.; Mao, W.-Y.; Wang, -S.-S. Changes in Volatile Compounds of Peanut Oil during the Roasting Process for Production of Aromatic Roasted Peanut Oil. J. Food Sci. 2011, 76, C404–412. DOI: 10.1111/j.1750-3841.2011.02073.x.
- Durmaz, G.; GöKmen, V. Changes in Oxidative Stability, Antioxidant Capacity and Phytochemical Composition of Pistacia Terebinthus Oil with Roasting. Food Chem. 2011, 128, 410–414. DOI: 10.1016/j.foodchem.2011.03.044.
- Qi, P.; Hong, H.; Liang, X.-Y.; Liu, D.-H. Assessment of Benzoic Acid Levels in Milk in China. Food Control. 2009, 20, 414–418. DOI: 10.1016/j.foodcont.2008.07.013.
- Ding, M.-Z.; Peng, J.; Ma, S.-L.; Zhang, Y.-C. An Environment-Friendly Procedure for the High Performance Liquid Chromatography Determination of Benzoic Acid and Sorbic Acid in Soy Sauce. Food Chem. 2015, 183, 26–29. DOI: 10.1016/j.foodchem.2015.03.025.
- Sieber, R.; Bütikofer, U.; Bosset, J. O. Benzoic Acid as a Natural Compound in Cultured Dairy Products and Cheese. Int. Dairy J. 1995, 1995(5), 227–246. DOI: 10.1016/0958-6946(94)00005-A.
- Ministry of Health of the People’s Republic of China and Standardization Administration of the People’s Republic of China. Hygienic Hygienic Standard for Uses of Food Additives. China, GB 2760-2014, 2005.
- Cheng, H.-Y.; Friis, A.; Leth, T. Partition of Selected Food Preservatives in Fish Oil–Water Systems. Food Chem. 2010, 122, 60–64. DOI: 10.1016/j.foodchem.2010.01.070.
- Garcia, C. V.; Quek, S. Y.; Stevenson, R. J.; Winz, R. Characterisation of Bound Volatile Compounds of a Low Flavour Kiwifruit Species: Actinidia Eriantha. Food Chem. 2012, 134, 655–661. DOI: 10.1016/j.foodchem.2012.02.148.
- AOCS. Official Methods and Recommended Practices of the American Oil Chemists’ Society, 4th ed.; AOCS press: Champaign, IL, 1997.
- Ning, C.-L.; Jiang, Y.; Meng, J.-S.; Zhou, C.-H.; Tao, J. Herbaceous Peony Seed Oil: A Rich Source of Unsaturated Fatty Acids and γ‐tocopherol. Eur. J. Lipid Sci. Technol. 2014, 117, 532–542. DOI: 10.1002/ejlt.201400212.
- Durmaz, G.; Karabulut, I.; Topçu, A.; Asiltürk, M.; Kutlu, T. Roasting-Related Changes in Oxidative Stability and Antioxidant Capacity of Apricot Kernel Oil. J. Am. Chem. Soc. 2010, 87, 401–409.
- Gutfinger, T.;. Polyphenols in Olive Oils. J. Am. Chem. Soc. 1981, 58, 966–968.
- Ministry of Health of the People’s Republic of China and Standardization Administration of the People’s Republic of China Determination of Benzoic Acid, Sorbic Acid and Saccharin Sodium in Foods. China, GB/T 5009.28-2016 (2016).
- Ling, B.; Yang, X.-M.; Li, R.; Wang, S.-J. Physicochemical Properties, Volatile Compounds, and Oxidative Stability of Cold Pressed Kernel Oils from Raw and Roasted Pistachio (Pistacia Vera L. Var Kerman). Eur. J. Lipid Sci. Technol. 2016, 118, 1368–1379. DOI: 10.1002/ejlt.v118.9.
- Ministry of Health of the People’s Republic of China and Standardization Administration of the People’s Republic of China. Hygienic Standard for Edible Vegetable Oil. China, GB 2716-2005 (2005).
- Mabaleha, M. B.; Mitei, Y. C.; Yeboah, S. O. A Comparative Study of the Properties of Selected Melon Seed Oils as Potential Candidates for Development into Commercial Edible Vegetable Oils. J. Am. Chem. Soc. 2007, 84, 31–36.
- Lee, Y. C.; Oh, S. W.; Chang, J.; Kim, I. H. Chemical Composition and Oxidative Stability of Safflower Oil Prepared from Safflower Seed Roasted with Different Temperatures. Food Chem. 2004, 84, 1–6. DOI: 10.1016/S0308-8146(03)00158-4.
- Durmaz, G.; Alpaslan, M. Antioxidant Properties of Roasted Apricot (Prunus Armeniaca L.) Kernel. Food Chem. 2007, 100, 1177–1181. DOI: 10.1016/j.foodchem.2005.10.067.
- Lasekan, O.;. Headspace Solid-Phase Microextraction Gas Chromatography-Mass Spectrometry (HS-SPME-GC-MS) Determination of Volatile Compounds in Roasted Plantains (French Sombre and Dwarf Kalapua). LWT - Food Sci. Technol. 2012, 46, 536–541. DOI: 10.1016/j.lwt.2011.11.012.
- Yang, J.-H.; Pan, Z.-L.; Takeoka, G.; Mackey, B.; Bingol, G.; Brandl, M. T.; Garcin, K.; McHugh, T. H.; Wang, H. Shelf-Life of Infrared Dry-Roasted Almonds. Food Chem. 2013, 138, 671–678. DOI: 10.1016/j.foodchem.2012.09.142.
- Siegmund, B.; Murkovic, M. Changes in Chemical Composition of Pumpkin Seeds during the Roasting Process for Production of Pumpkin Seed Oil (Part 2: Volatile Compounds). Food Chem. 2004, 84, 367–374. DOI: 10.1016/S0308-8146(03)00241-3.
- Shahidi, F.; Aishima, T.; Abou-Gharbia, H. A.; Youssef, M.; Shehata, A. Y. Effect of Processing on Flavor Precursor Amino Acids and Volatiles of Sesame Paste (Tehina). J. Am. Chem. Soc. 1997, 74, 667–678.
- Frauendorfer, F.; Schieberle, P. Changes in Key Aroma Compounds of Criollo Cocoa Beans during Roasting. J. Agric. Food Chem. 2008, 56, 10244–10251. DOI: 10.1021/jf802098f.
- Xiao, L.; Lee, J.; Zhang, G.; Ebeler, S. E.; Wickramasinghe, N.; Seiber, J.; Mitchell, A. E. HS-SPME GC/MS Characterization of Volatiles in Raw and Dry-Roasted Almonds (Prunus Dulcis). Food Chem. 2014, 151, 31–39. DOI: 10.1016/j.foodchem.2013.11.052.
- Kiralan, M.; Kiralan, S. S. Changes in Volatile Compounds of Black Cumin Oil and Hazelnut Oil by Microwave Heating Process. J. Am. Chem. Soc. 2015, 92, 1445–1450.
- Loch, C.; Reusch, H.; Ruge, I.; Godelmann, R.; Pflaum, T.; Kuballa, T.; Schumacher, S.; Lachenmeier, D. W. Benzaldehyde in Cherry Flavour as a Precursor of Benzene Formation in Beverages. Food Chem. 2016, 206, 74–77. DOI: 10.1016/j.foodchem.2016.03.034.