ABSTRACT
Msalais is a traditional Chinese wine. The strong caramel odor makes Msalais monotonous and inharmonious, with more imbalances in industrially produced Msalais. In this study, the aromatic evolution of Msalais during industrial production was analyzed by sensory testing and gas chromatography-mass spectrometry. The analysis revealed that dynamic changes of corresponding aromatic compounds were consistent with the change of odors. The concentration of grape juice caused strong caramel odor and weak floral and herbaceous odors in industrial Msalais. Further, controlled fermentation ameliorated the imbalance. These findings revealed the traditional aromatic characteristics that should be improved during the industrial production of Msalais.
Introduction
Full knowledge of the aroma evolution of wine is necessary for the control of the quality of the finished wine product, especially for the improvement and stability of key active-odor compounds[Citation1,Citation2], and to control the off-flavors.[Citation3] Msalais is a traditional wine produced by the Uygur ethnic group. The method used to produce Msalais is unique among wines. The juice of the local grape “Hetianhong” grape (Vitis vinifera Hetianhong) is concentrated and then spontaneously fermented into Msalais.[Citation4] The sensory characteristics of Msalais, i.e., its unique flavor and absence of astringent taste, are completely different from those of other types of wine.[Citation5] We have previously shown that caramel is the typical aroma characteristic of Msalais.[Citation5]The method of winemaking contributes to the aromatic characteristics of Msalais more than the grape cultivar, Hetianhong, an indigenous grape variety with a neutral aroma. However, the strong caramel odor often makes the aromatic characters of Msalais monotonous and inharmonious. The phenomenon is more obvious in industrially produced Msalais wine (IMW), which marks a shift away from craft-made Msalais wine (CMW) (S1 ), accompanying an upsurge in the economic development of China. Meeting the high and diverse demand for Msalais is a big challenge. The IMW Msalais is dominant in the market, with the quality more stable than that of CWM because of the relatively consistent process of large-scale production. However, this risks an imbalance of strong caramel in the final product because of the lack of scientific know-how, and the inapplicability of the experience of CMW enologists to IMW production.
Aromatic compounds in wine can arise from the grape berry, and during fermentation, storage, or aging of wine.[Citation6,Citation7] The evolution of aromatic notes in wine is complex and intricate.[Citation7] The complexity of aromatic development during Msalais production is no less complex than that of wine. To this end, the maximum improvement of aromatic characteristics of traditional Msalais during IMW production is an urgent issue for high-quality production of this economically important product. In the current study, the aromatic evolution during the production of IMW was investigated using gas chromatography-mass spectrometry (GC-MS) analysis after headspace-solid-phase microextraction (HS-SPME) and by sensory testing. The presented findings may be used to control the aromatic quality of IMW by improving the technological production process.
Materials and methods
Sample collection
Compressed Hetianhong grape juice was automatically pumped into an 8000-L steel steam-jacketed tank. The ºBrix and pH of harvested grape varied between 16 and 22, and 3.55 and 4.07, respectively, according to the harvest time. A-F represented the six different concentrating process of grape juice harvested at different times. The first samples were collected when the tank was full (A1, B1, C1, D1, E1, and F1). The subsequent samples were taken when the juice began to boil (A2, B2, C2, D2, E3, and F2) and then during boiling every hour until a concentration of 25–29 °Brix was achieved (A3-A20; B3-B21, C3-C13, E3-E7, and F3-F8). The final sample was taken once the grape concentrate was cooled down to room temperature (A20, B21, C14, D9, E8, and F9). The notation 5f, 9f, and 11f represent the three different fermenting processes in the 5th, 9th, and 11th steel tanks with 20 KL volume. The first sample was collected on day 0 (5fd0, 9fd0, and 11fd0). The samples were collected daily during the first 18 d of fermentation, and then every week over the course of 100 d (5fd1-5fd107; 9fd1-9fd106; and 11fd2d-11fd90d). The sample data are shown in . All samples were collected in triplicate, with 100 mL collected for each repetition.
Table 1. Samples collected from Awati Dao Lang Msalais Co., Ltd. in 2016
Standards
The standards used were of at least 98% purity and were purchased from Sigma-Aldrich (St. Louis, MO, USA). A synthetic matrix was prepared for dilution of individual compounds. The synthetic matrix was composed of 12% (v/v) ethanol, 200 g/L glucose, and 7 g/L tartaric acid at a pH of 3.3 (pH was adjusted with 1 M NaOH). Fifteen dilutions of standard solutions in the matrix were used to construct the GC-MS calibration curves. These were analyzed in triplicate for every standard.
Sensory assay
The panel was composed of 17 females and 19 males (23–40 years old), all with previous Msalalais aroma quantitative descriptive analysis experience. This panel was trained to be familiar with technology, seven odors of Msalais wine[Citation5], the reference standards and scale use, and to perform a descriptive analysis of the samples, according to the method described in the GB/T 15038–2006. Seven aroma attributes and “other” produced in the previous work were used for the quantitative analysis of 20 Msalais wines.[Citation5] They included the following: caramel (sugar, sweet, honey, burnt, roasted, cola), baked (bread, cake), fruity (tropical fruity, berry fruity, and tree fruity), dried fruit (raisin, dried apricot, fig, red date, prune), fruit jam (stewed apple, tomato paste), floral (rose or other blossoms), and herbaceous (traditional Chinese medicine, dried tangerine, grass, spice).[Citation5] Samples (ca. 40 mL) were served in wine glasses and labeled with random three-digit numbers. During 30 sessions held over 7 d, the assessors scored each attribute on a 0–5 scale, with 0 signifying no intensity and 5 signifying the highest intensity.
GC-MS analysis
The GC-MS analysis was performed using an Agilent 6890 gas chromatograph coupled with an Agilent 5975C mass spectrometer (Agilent, Santa Clara, CA, USA) with an HP-Innowax column (60 m, 0.25 mm, 0.5 m; Agilent). Automatic HS-SPME was performed using a 2-cm divinylbenzene/carboxen/polydimethylsiloxane 50/30-m SPME fiber (Supelco, Bellefonte, PA, USA) and a CTC CombiPAL autosampler (CTC Analytics, Zwingen, Switzerland). A 5-mL sample of Msalais wine was mixed with 10 μL of 4-methyl-2-pentanol (internal standard, 0.9898 μg/L) and 1 g of NaCl in a 20-mL vial capped with a polytetrafluoroethylene silicon septum. Each sample was injected twice. Retention indices were calculated using a C7–C24 n-alkane series (Supelco) under the same chromatographic conditions. When reference standards were not available, tentative identifications were made according to the methods of Cai et al.[Citation8], by matching the obtained mass spectra and the retention indices after resolution on the HP-Innowax column with those reported in the NIST 11 library. The concentrations of the identified compounds were obtained by interpolating the relative areas from the internal standard area in the calibration curves. The concentration of volatile compounds for which no pure reference was available was obtained using the calibration curves for compounds with the most similar chemical structures.[Citation8] For individual aromatic compounds, the odor activity value (OAV) was obtained based on the concentration/threshold ratio.[Citation8]
Statistical analysis
The peak area of the aroma compounds was normalized in each batch by using the equation:
where i represents compound number and j represents batch number. Canonical correlations between sensory data and concentrations of volatile compounds were calculated using Microsoft Excel software 2010. The heatmap of the standardized data for peak area, the mean sensory test, and the canonical correlations between them were constructed by using Henm1.1.0.3.3 (http://hemi.biocuckoo.org). SPSS software (IBM, Armonk, NY, USA) was used to calculate the mean and variance of data from the sensory tests, and the contents of volatile compounds.
Results and discussion
Sensory aroma evolution during the making of Msalais wine
As shown in , sensory testing indicated that the intensity of caramel, fruity, dried fruit, and fruit jam odor was higher than that of baked, floral, and herbaceous odor, and also much higher at the concentration stage than during fermentation. Seven odors were classified into three groups according to their changing intensity trends during the winemaking process, namely: (1) caramel and baked; (2) fruity, dried fruit, and fruit jam; and (3) floral and herbaceous. With the proceeding grape juice concentration, the intensity of caramel and baked aroma odors became stronger, with that of the fruit odor decreasing in early mid-phase, and increasing in the late phase; meanwhile, the intensities of dried fruit and fruit jam odors both increased. Conversely, the intensities of both floral and herbaceous odors decreased during concentration. With increasing fermentation time, the intensity of all odors, except for baked, decreased, especially the caramel, dried fruity, fruit jam, and fruit odors. The intensity of the baked odor remained stable or slightly increased. During fermentation, the intensity of caramel and fruit odors was stronger than that of other odors, especially the weak floral and herbaceous odors.
Evolution of volatile compounds during the making of Msalais wine
Overall, 130 compounds were detected during the whole process with highly complex evolution at different stages. The changes of all volatiles were outline the profile (). Representative samples were selected for points at which most of the 130 compounds had obviously fluctuated during the industrial process. The representative samples included the specific materials, such as grape juice or newly boiled grape juice (A2, B1, C1, D1, E1, and F1); end concentration stage (A18, B19, C14, D9, E8, and F9); initial or early fermentation (5fd0, 9fd0, and 11fd2); and the final Msalais products (5fd107, 9fd106, and 11fd90). OAVs are often used to determine the contribution of specific compounds to the aroma of wine[Citation7,Citation8]. Although only those compounds with an OAV≥1 can be perceived and contribute to the overall aroma[Citation7,Citation8], the changing content of 38 compounds with OAVs≥0.1 during the industrial production was analyzed in detail (–). These compounds were analyzed as potential compounds that could be used to improve the monotonous and inharmonious Msalais aroma.
Table 2. The potential aromatic compounds with maximum OAVs ≥ 0.1
Table 3. Contents of 38 compounds with OAV≥0.1 in the representative samples for Msalais process (μg/L)
Table 4. Contents of 38 compounds with OAV≥0.1 in the representative samples for Msalais process (μg/L)
Figure 2. Evolution of volatile compounds during Msalais wine production based on the normalization data matrix of the determined peak area
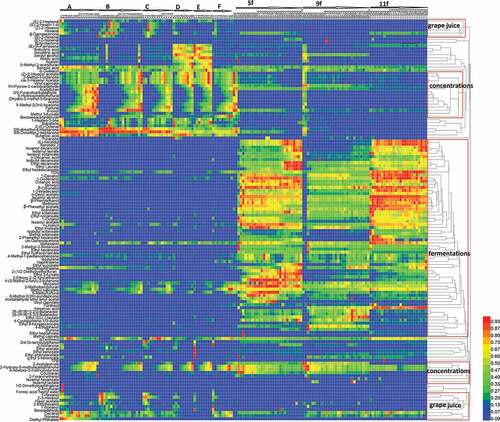
As shown in , the species and content of volatile compounds in Msalais were influenced by the grape juice and the processing technology, including grape juice concentration and natural fermentation. The volatile compounds were present in grape juice, and those produced by grape juice concentration were distinct from those produced during fermentation. The process of grape juice concentration resulted in a reduction in the levels of most grape juice compounds present such as C6 aldehydes and alcohols. However, grape juice concentration promoted the formation of heterocyclic compounds, mainly furans. The natural fermentation process was an important step for the formation of many alcohols, aldehydes, fatty acids, and esters, although a few of them were present (albeit their abundant was very low) in the concentrated juice. Very few compounds from grape juice such as some monoterpenes and C13-norisoprenoids were observed in wine during these two processes. Based on the points of high abundance of 130 compounds detected during the entire process, the majority of these compounds could be roughly classed in three groups, as most abundant in grape juice, or during concentration and fermentation.
Volatiles from grape juice
C6 aldehydes and alcohols, monoterpenes, and C13-norisoprenoids are important for the grape aroma.[Citation6,Citation7,Citation9] Some esters, such as acetate esters of short-chain alcohols, and some monoterpene alcohols occur naturally in fruit. They were all found in the grape juice, but their evolution in Msalais production was much more complex than during wine fermentation because of the concentration of grape juice. The evolution of these aroma compounds followed a general rule wherein a large number of aroma compounds from grape exhibited low abundance that decreased rapidly during concentration, including C6 compounds, terpenes, aldehydes, esters, alcohols, and volatile fatty acids. The main reason for such decrease during the concentration of grape juice was chemical transformation or physical evaporation under low pH, aerobic, and high-temperature conditions.
Three compound subgroups from grape juice () included all C6 compounds, aldehydes [(E)-2-heptenal, (E)-2-nonenal, 2-(E)-octenal, decanal, benzaldehyde], ketones (1-hepten-3-one;2,6-dimethyl-4-heptanone and 4,6-dimethyl-2-heptanone), 1-nonanol, β-damascone, sulcatone, and butanoic acid. They were present in the grape juice and at the early stage of grape juice concentration, with their levels decreasing or disappearing during the concentration process, and continued to decrease or shifted to increase during the fermentation process. As shown in and , (E)-2-hexenal was present in grape juice and at early concentration stages in varying amounts (7–20 μg/L) in different batches. Its levels decreased to approximately 6.5 μg/L during grape juice concentration and further decreased to trace level during fermentation. The 1-Hexanol content was high during early concentration (nearly 2000 μg/L), and then rapidly decreased to trace level at the end of the concentration process, slightly increasing (to 200 μg/L) during late fermentation. The levels of C6 aldehydes, especially (Z)-2-hexenal and n-hexanal, compounds which are abundant in the grape and grape juice, decreased during fermentation because of oxygenation.[Citation6] They are also the precursors of ethyl hexanoate[Citation10], an important fruity ester produced during fermentation. The highest levels of (E)-2-heptenal were noted during grape concentration; they then slightly increased to approximately 0.6 μg/L during fermentation. (E)-2-Nonenal, (Z)-geranylacetone, and decanal were present in trace amounts or not detected during concentration, but their levels increased during fermentation. The content of nonanal was low (2–5 μg/L) during grape juice concentration and slightly higher (no more than 15 μg/L) during late fermentation.
Volatiles produced during concentration of grape juice
During concentration, heterocyclic compounds (mainly furans) were produced in large quantities, to decrease and even disappear rapidly during fermentation (). For example, as shown in and furfural levels clearly decreased from over 1000 μg/L to approximately 70 μg/L during fermentation. However, the levels of few such compounds increased during both concentration and fermentation, e.g., 5-methylfurfural. The level of 5-methylfurfural kept increasing from 20 to 30 μg/L during concentrations to 500 μg/L during fermentation. Strecker aldehydes, hydroxyl ketones, furans, etc., are often present in the food matrix and formed by non-enzymatic browning reactions.[Citation12] The levels of the majority of aromatic compounds formed during concentration were reduced or disappeared rapidly during fermentation because these aromatic compounds are often intermediate products of the Maillard reaction; e.g., 5-hydroxymethyl furfural (5-HMF), furanones, and acetylacetals, which are unstable and easily converted into larger polymer compounds, such as melanoidins.[Citation13] The levels of some furanes from carbohydrate degradation, such as furfural, ethyl furoate, 2-acetylfuran, furfuryl alcohol, 2-ethoxymethyl-5-furfural, and 2-hydroxymethyl-5-furfural, decrease to the point where they cannot be detected during wine aging.[Citation6] 5-Methylfurfural levels also increased during fermentations, which is attributed to the oxidation of the resulting 5-HMF.[Citation14] α-Guaiacol appeared to not be influenced by the Msalais winemaking process, and their content was stable during concentration and fermentation. α-Terpineol levels also increased greatly during concentration, which may be caused by the release from a precursor of bounded linalool or conversation from geraniol.[Citation15] Pasteurization of grape juice significantly increases the release of bound α-terpineol from grape juice[Citation16], which is consistent with the findings of the current study. Further, benzoic acid, (Z)-2-hexenyl acetate, 2-methyl-3-ocanone, and vinyl caproate accumulated during grape juice concentration, and decreased or disappeared during fermentation.
Volatiles greatly accumulated during fermentation
In wine, acetate esters and fatty acid ethyl esters are produced during fermentation, including ethyl esters of diprotic acids, such as ethyl acetate. C4–C11 alcohols branched and unsaturated short-chain alcohols, including some aromatic alcohols, are found in grape, but they are produced in bulk during fermentation. Ethanol, glycerol, diols, and higher alcohols are other important alcohols produced by yeast.[Citation6,Citation7] Most fatty acids, lactones, volatile phenols, and volatile sulfur compounds are generated during fermentation[Citation6,Citation7] . The levels of the majority of higher alcohols and fatty acids decrease to some degree because of esterification during storage[Citation6,Citation7] . These aromatic compounds are generated by spontaneous fermentation of primary and secondary metabolites by indigenous microorganisms, or by enzymatic and chemical hydrolytic release of the bound aromatic compounds from grape materials, e.g., β-citronellol, β-damascone, isoamyl alcohol, and benzoic acid.[Citation9,Citation17] At the same time, levels of some acetates decrease during wine storage, depending on the equilibrium with their corresponding acids and alcohols.[Citation6,Citation7,Citation18] The concentration of straight chain fatty acid ethyl esters remains fairly constant during storage.[Citation6,Citation7]
Comparing the evolutions of the complex aromatic compounds during the winemaking process, the changes of aromatic compounds during Msalais fermentation were more complex. Most compounds within this group, including most alcohols, esters, and fatty acids were not found or present in only low quantities in grape juice (). Their levels gradually decreased during concentration but significantly increased during fermentation (). The potential aromatic compounds with OAVs ≥ 0.1 (maximum values during production) were scrutinized as follows (–)
The content of isoamyl alcohol was low, 300 μg/L, early in the concentration process. It was reduced to trace level during the grape juice concentration, increasing during fermentation to over 10 mg/L. The content of isobutanol was relatively stable during grape juice concentration, approximately 11–14.5 mg/L, but it increased to 80–136 mg/L later during fermentation. The content of β-phenylethanol in grape juice concentrate was less than 500 μg/L, and slightly decreased with concentration time, to approximately 200 μg/L. However, during fermentation, it increased more than 90-fold to 18.82 mg/L. Ethyl decanoate and ethyl octanoate were present at nearly 14 μg/L and 17 μg/L, respectively, and did not change during concentration. During fermentation, ethyl decanoate levels increased to 200–400 μg/L and those of ethyl octanoate increased to over 70 μg/L. In some concentrated samples, the levels of ethyl hexadecanoate and ethyl hydrocinnamate were low, but this varied between batches, suggesting that the two compounds were likely affected by grape quality. During fermentation, their levels slightly increased to approximately 300 μg/L and 3.0 μg/L, respectively. Ethyl heptanoate, ethyl hexanoate, and ethyl linoleate were not detected during concentration, whereas they cumulated up to 5 μg/L, 50 μg/L, and approximately 1 μg/L, respectively, during fermentation. Ethyl phenylacetate was present at the early concentration stages; its content decreased to trace level by the end of concentration and subsequently increased to 49 μg/L during fermentation. Ethyl acetate and isoamyl acetate were both present during grape juice concentration. The levels of ethyl acetate changed dramatically, decreasing from 260 to 975 μg/L in the grape juice or at early concentration stage to 13–22 μg/L at the end of concentration, and then increasing to over 3400 μg/L at the end of fermentation. The isoamyl acetate content was maintained at approximately 480 μg/L during grape juice concentration, but increased 2–3 times during the concentration period. β-Phenethyl acetate was present in grape juice concentrates at a level of less than 5.5 μg/L, which slightly decreased with concentration time, to approximately 1.4 μg/L. However, during fermentation, its levels increased over 100-fold, to 537 μg/L. Isoamyl octanoate was not detected during concentration, but its levels increased during fermentation. For fatty acids, butanoic acid and hexanoic acid were both present during concentration, at 80–154 μg/L and nearly 200 μg/L, respectively. The levels of the two compounds both increased during fermentation, by over 100-fold and two-fold, respectively. Isobutyric acid levels decreased during grape concentration by 0–150 μg/L, and increased to over 130 μg/L during fermentation. Ethyl 3-furoate, ethyl succinate, ethyl decanoate, octanoic acid, and decanoic acid were highly abundant during the aging period.
In addition, most complex volatiles with low abundance in the grape juice with, including terpenes, C13 norisoprenoids, volatile phenols, sulfides, and benzenes, were highly abundant in the “fermentation” group. This was consistent with high content produced during wine fermentation.[Citation18] The levels of some terpenes increased both during concentration and fermentation, such as β-citronellol and β-linalool, these compounds were both obviously produced during fermentation, and their content increased with fermentation time, from 11 μg/L to 13μ g/L over 90 days. β-Linalool was detected during concentration, at less than 1 μg/L, and its levels slightly decreased during grape concentration. β-Citronellol was not detected during concentration. C13 norisoprenoids were detected in two cases. The levels of β-damascone decreased rapidly during concentration, but markedly increased during fermentation, while those of 1,1,6-trimethyl-1,2-dihydronaphthalene (TDN) and 4-(2,3,6-trimethylphenyl)buta-1,3-diene (TPB) increased significantly during fermentation. The levels of TDN were relatively stable at approximately 0.6 g/L, while those of β-damascenone slightly decreased from near 2.5 μg/L to approximately 0.4 μg/L. Their levels increased to 2.7 μg/L and 12 μg/L, respectively, during fermentation. Methionol content in grape concentration samples was stable at approximately 325 μg/L, but greatly increased to over 1000 μg/L during fermentation. The levels of naphthalene and its derivatives decreased or remained stable at the late stage of concentration, but increased during the fermentation period. For example, the naphthalene content was stable at approximately 2.2 μg/L, and slightly increased to 12.8 μg/L during fermentation. In addition, 4-ethylphenol and 1-catechol content remained stable during concentration and fermentation, while that of guaiacol increased during both concentration and fermentation. 1-Dodecanol, myrceol, 2-methybenzofuran, and methyl salicylate levels increased during concentration, and also during fermentation. Pyranone, isodurene, and 4-cyclopentene-1,3-dione were similarly produced in large quantities during fermentation.
Furaneol was not detected during concentration, but was present during fermentation, at over 18.6 mg/L at the end of fermentation. However, furaneol levels increased continuously during fermentation. The compound is a product of chemical transformation (via the Maillard reaction) or microbial biotransformation (via enzymatic hydrolysis of glycosides), as well as synthesized from the Maillard products as substrates.[Citation19–Citation21] Additionally, furanones such as 3-hydroxy-4, 5-dimethyl-2 (5H)-furanone, 2,5-dimethyl-4-hydroxy-3(2H)-furanone, and 2-ethyl-5-methyl-4-hydroxy-3(2H)-furanone are present as important aromatic compounds in oxidative wine or in oak wood-aged wine.[Citation22] In Msalais, the increase of furanones contents was not possible because of Msalais aging in a jar instead of oak wood barrel.
The majority of aromatic compounds were produced in large quantities during fermentation, and they were retained in the final Msalais wine product as the main aromatic components. Thus, high-quality wine strains and effective fermentation conditions can be useful and important for improving the composition of compounds associated with floral and herbaceous odors, and/or for reducing the content of compounds associated with caramel odor. For example, the balance between strong caramel and weak floral odors in the final Msalais wine can be achieved by increasing β-citronellol, β-linalool,β-damascenone,β-phenylethanol, and ester content, and/or decreasing furaneol and 5-methylfurfural content by controlling the fermentation conditions.
Canonical correlations between sensory characteristics and aromatic compounds
Comparison of and 2 revealed that the aroma kept changing during Msalais production because of the evolution of a series of aromatic compounds. This was roughly indicated by the canonical correlations between the sensory analysis and content of the volatile matrix (). In , (E)-2-Hexenol, (Z)-2-hexenol, (E)-2-hexenal, and hexanal in Group 1 and (Z)-3-hexenol, 2-ethylhexanol, decanal, and nonanal in Group 5 were from the grape juice compounds (). They were highly correlated with the floral and herbaceous odors. Compounds from Group 2 were highly correlated with the caramel and baked odors. Acetylfuran, furfural, 5-methyl-2(3H)-furanone, acetol, methyl-3-furoate, butyolactone, and α-ionol were the important compounds in this group. α-Ionol was also highly correlated with the dried fruit odor. Compounds from Group 3 were highly correlated with the dried fruit and fruit jam odors. These compounds were mainly heterocycles including 2-(1,2-diethoxyethyl)furan, 2-ethoxy-2-(2-furyl)ethanol, ethyl-3-furate, 2-methybenzofuran, 5-methylfurfural, ethyl hydrocinnamate, 4-(5-methyl-furyl)-2-butanone, 6-methyl-3(2H)-pyridazinone, vinyl caprylate, ethyl benzoate, and ethyl linoleate, with most of them produced during grape juice concentration (). Most of these compounds were also highly correlated with the caramel and baked odors, e.g., 5-methylfurfural, 2-(1,2-diethoxyethyl)furan, and ethyl hydrocinnamate. Compounds from Group 4 were roughly correlated with the fruit jam odor.
Based on the correlations between volatiles and odors during IMW production, the caramel, baked, dried fruit, and fruit jam odors were strong during grape juice concentration but became weaker during fermentation. This corresponded to the bulk production of furan volatiles during grape juice concentration, decreasing during fermentation. While weak floral and herbaceous odors in the final products were associated with their corresponding compounds in the grape juice, they were difficult to retain during concentration and fermentation. The contributing compounds to fruit odor were complex, and included compounds that were produced during concentration (esters and furans) and whose levels decreased during fermentation, or those that were not abundant in grape juice and during grape juice concentration but highly abundant during fermentation (most fruity esters). Strong fruit odor during concentration may arise from the synergistic interaction of the compounds produced during grape juice concentration. In fact, fruity has been shown to be the important odor in caramelized food.[Citation23]Fruity odor was decreased during fermentations, although fruity esters accumulate in bulk during fermentation. This might be attributed to the high odor threshold values of fruity esters and masking the effect of strong caramel associated with furans. In fact, the interaction between odors should not be ignored, such as dried fruit and fruit jam, a result of interaction of esters, furans, sulfides and β-Damascenone[Citation24–Citation26]
Conclusion
The strong caramel odor and weak floral and herbaceous odors were attributed to the aromatic imbalance in Msalai wine. Floral and herbaceous odors were less pronounced in grape juice decreased rapidly during grape juice concentration, while caramel, baked, fruit jam, dried fruit, and fruit odors were produced during grape juice concentration and then decreased rapidly during fermentation. The changes of corresponding aromatic compounds during production were consistent with the change of odors. This study provides a good theoretical basis for the improvement of the aromatic characteristics of Msalais, by controlling the fermentation to increase the levels of the potential compounds associated with floral, herbaceous, and fruit odors, such as β-citronellol, β-linalool, β-damascenone, β-phenylethanol and esters, or decrease the levels of compounds associated with the caramel odor, especially furaneol.
Acknowledgments
The funding organizations did not play any role in the study design; collection, analysis, and interpretation of data; reporting; or the decision to submit the article for publication. We thank LetPub for linguistic assistance during the preparation of this manuscript. We sincerely thank the DaoLang Msalais Company and the Hongbaoshi Msalais Company for their generous provision of Msalais wine samples for this study. CQD contributed to the study design and provided the analysis platform; LXZ collected test data and drafted the manuscript; MMZ participated in sample collection; YS revised the manuscript.
Additional information
Funding
References
- Lambrechts, M.; Pretorius, I. S. Yeast and Its Importance to Wine Aroma - A Review. South African J. Enol. Vitic. 2000, 21, 97–129.
- Heras, M. O.; Rivero-Pérez, M. D.; Pérez-Magariño, S.; González-Huerta, C.; González-Sanjosé, M. L. Changes in the Volatile Composition of Red Wines during Aging in Oak Barrels Due to Microoxygenation Treatment Applied before Malolactic Fermentation. Eur. Food Res. Technol. 2008, 226, 1485–1493. DOI: 10.1007/s00217-007-0680-2.
- Ugliano, M.;. Oxygen Contribution to Wine Aroma Evolution during Bottle Aging. J. Agric. Food Chem. 2013, 61, 6125–6136. DOI: 10.1007/s00217-007-0680-2.
- Lixia, Z.; Mingfu, G.; Dongqi, G.; Christensen, M.; Xujie, H.; Hongmei, L.; Yingge, F.; Shu, F. Preliminary Analysis of Yeast Communities Associated with the Spontaneous Fermentation of Musalais, a Traditional Alcoholic Beverage of Southern Xinjiang, China. South African J. Enol. Vitic. 2012, 33, 95–104.
- Zhu, L. X.; Zhen, W.; Wang, L.; Feng, S. Quantitative Descriptive Analysis of Sensory Characteristics of Musalais from A’wati, Xinjiang. Food Sci. 2013, 1, 38–44.
- Rapp, A.; Mandery, H. Wine Aroma. Experientia. 1986, 42, 44–47. DOI: 10.1007/BF01941764.
- Styger, G.; Prior, B.; Bauer, F. F. Wine flavor and aroma. J. Ind. Microbiol. Biotechnol. 2011, 38, 1145–1159. DOI: 10.1007/s10295-011-1018-4.
- Cai, J.; Zhu, B. Q.; Wang, Y. H.; Lu, L.; Bin, L. Y.; Reeves, M. J.; Duan, C. Q. Influence of Pre-Fermentation Cold Maceration Treatment on Aroma Compounds of Cabernet Sauvignon Wines Fermented in Different Industrial Scale Fermenters. Food Chem. 2014, 154, 217–229. DOI: 10.1016/j.foodchem.2014.01.003.
- Cliff, M.; Stanich, K.; Trujillo, J. M.; Toivonen, P.; Forney, C. F. Determination and Prediction of Odor Thresholds for Odor Active Volatiles in a Neutral Apple Juice Matrix. J. Food Qual. 2011, 34, 177–186. DOI: 10.1111/j.1745-4557.2011.00383.x.
- Budić-Leto, I.; Zdunić, G.; Banović, M.; Tomić-Potrebuješ, I.; Lovrić, T. Fermentative Aroma Compounds and Sensory Descriptors of Traditional Croatian Dessert Wine Prošek from Plavac Mali Cv. Food Technol. Biotechnol. 2010, 48, 530–537.
- Genovese, A.; Gambuti, A.; Piombino, P.; Moio, L. Sensory Properties and Aroma Compounds of Sweet Fiano Wine. Food Chem. 2007, 103, 1228–1236. DOI: 10.1016/j.foodchem.2006.10.027.
- Sarrazin, E.; Dubourdieu, D.; Darriet, P. Characterization of Key-Aroma Compounds of Botrytized Wines, Influence of Grape Botrytization. Food Chem. 2007, 103, 536–545. DOI: 10.1016/j.foodchem.2006.08.026.
- Van Boekel, M. A. J. S.;. Formation of Flavour Compounds in the Maillard Reaction. Biotechnol. Adv. 2006, 24, 230–233. DOI: 10.1016/j.biotechadv.2005.11.004.
- Cutzach, I.; Chatonnet, P.; Dubourdieu, D. Study of the Formation Mechanisms of Some Volatile Compounds during the Aging of Sweet Fortified Wines. J. Agric. Food Chem.. 1999, 2837–2846. DOI: 10.1021/jf981224s.
- Loscos, N.; Hernández-Orte, P.; Cacho, J.; Ferreira, V. Evolution of the Aroma Composition of Wines Supplemented with Grape Flavour Precursors from Different Varietals during Accelerated Wine Ageing. Food Chem. 2010, 120, 205–216. DOI: 10.1016/j.foodchem.2009.10.008.
- Marais, J.;. Terpenes in the Aroma of Grapes and Wines: A Review. South African J. Enol. Vitic. 1983, 4, 49–58.
- Cordente, A. G.; Curtin, C. D.; Varela, C.; Pretorius, I. S. Flavour-Active Wine Yeasts. Appl. Microbiol. Biotechnol. 2012, 96, 601–618. DOI: 10.1007/s00253-012-4370-z.
- Bordiga, M.; Rinaldi, M.; Locatelli, M.; Piana, G.; Travaglia, F.; Coïsson, J. D.; Arlorio, M. Characterization of Muscat Wines Aroma Evolution Using Comprehensive Gas Chromatography Followed by a Post-Analytic Approach to 2D Contour Plots Comparison. Food Chem. 2013, 140, 57–67. DOI: 10.1016/j.foodchem.2013.02.051.
- Schieberle, P.;. The Carbon Module Labeling (Camola) Technique A Useful Tool For Identifying Transient Intermediates In The Formation Of Maillard-Type Target Molecules. Ann. Ny. Acad. Sci. 2005, 248, 236–248. DOI: 10.1196/annals.1333.029.
- Dahlen, T.; Hauck, T.; Wein, M.; Schwab, W. 2,5-Dimethyl-4-Hydroxy-3(2h)-Furanone as a Secondary Metabolite from D-Fructose-1,6-Diphosphate Metabolism by Zygosaccharomyces Rouxii. J. Biosci. Bioeng. 2001, 91, 352–358. DOI: 10.1196/annals.1333.029.
- Hayashida, Y.; Kumamoto-Ken., I. R. I.; Hatano, M.; Tamura, Y.; Kakimoto, M.; Nishimura, K.; Igoshi, K.; Kobayashi, H.; Kuriyama, H. 4-Hydroxy-2,5-Dimethyl-3(2h)-Furanone (HDMF) Production in Simple Media by Lactic Acid Bacterium, Lactococcus Lactis Subsp. Cremoris IFO 3427. J. Biosci. Bioeng. 2001, 91, 97–99. DOI: 10.1016/S1389-1723(01)80121-3.
- Jarauta, I.; Cacho, J.; Ferreira, V. Concurrent Phenomena Contributing to the Formation of the Aroma of Wine during Aging in Oak Wood: An Analytical Study. J. Agric. Food Chem.. 2005, 53, 4166–4177. DOI: 10.1021/jf0481960.
- Paravisini, L.; Septier, C.; Moretton, C.; Nigay, H.; Arvisenet, G.; Guichard, E.; Dacremont, C. Caramel Odor : Contribution of Volatile Compounds according to Their Odor Qualities to Caramel Typicality. Frin. 2014, 57, 79–88. DOI: 10.1016/j.foodres.2014.01.009.
- Pineau, B.; Barbe, J. C.; Van Leeuwen, C.; Dubourdieu, D. Which Impact For β-Damascenone On Red Wines Aroma? J. Agric. Food Chem. 2007, 55, 4103–4108. DOI: 10.1021/jf070120r.
- Mateo-Vivaracho, L.; Cacho, J.; Ferreira, V. Improved Solid-Phase Extraction Procedure for the Isolation and In-Sorbent Pentafluorobenzyl Alkylation of Polyfunctional Mercaptans: Optimized Procedure and Analytical Applications. J. Chromatogr. A. 2008, 1185, 9–18. DOI: 10.1016/j.chroma.2008.01.037.
- San-Juan, F.; Ferreira, V.; Cacho, J.; Escudero, A. Quality and Aromatic Sensory Descriptors (Mainly Fresh and Dry Fruit Character) of Spanish Red Wines Can Be Predicted from Their Aroma-Active Chemical Composition. J. Agric. Food Chem. 2011, 59, 7916–7924. DOI: 10.1021/jf1048657.