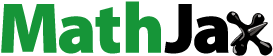
ABSTRACT
Current work evaluated the effects of 12-month storage, at chilled (4ºC) and ambient (25ºC) temperatures, on the physical properties, resistant starch (RS) content and antioxidant profile of purple sweet potato (PSP) powder. This study revealed two findings: (i) The antioxidant content of food powders was not always reflective of its antioxidant capacity and (ii) Chilled storage could not prevent the loss of RS and antioxidant activity upon 12-month storage. It is suggested that measurements of antioxidant content and antioxidant activity should be performed concurrently on the same sample.
Introduction
The health benefits of purple sweet potato (PSP) have been widely recognized in present days. This tuberous crop is high in vitamin A, vitamin C and manganese, and represents a good source of dietary fiber, copper, potassium and iron.[Citation1] Also, PSP is rich in anthocyanin, a phenolic pigment that contributes significantly to the vibrant purple color and powerful antioxidative nature of PSP.[Citation2] Besides being incorporated into yogurt drinks, confectionaries and fruit jams as natural food colorant, anthocyanin demonstrates exceptional nutraceutical properties including antioxidative, anticancer, antimicrobial effects and promotes cardiovascular as well as visual health.[Citation2] In particular, anthocyanin from PSP has been shown to produce in vivo hypoglycemic activity and hepatoprotective effect against alcohol-induced liver injury in mice,[Citation3,Citation4] thus it is viewed as a high-value ingredient.
Despite the multiple benefits of anthocyanin, PSP is highly perishable and difficult to store. In order to extend its shelf life, the fresh tuber is turned into flour/powder product that is less susceptible to spoilage. The versatility of PSP flour has been widely demonstrated as soup/sauce thickener, baby food, ingredient for making waffle, smoothies and ice cream, as well as gluten-free alternative for wheat flour in bakery goods. Recently, PSP powder has been added into biscuit[Citation5] and cake formulations[Citation6] that demonstrated good consumers’ acceptability. Alternatively, PSP starch is isolated for different purposes in food and non-food industries, such as noodles, candy products and textile industry, due to its outstanding structural and functional properties.[Citation7]
While most work focus on maximizing the application of PSP as food ingredients and products (i.e., powder and starch), little pays attention to the changes in the physical properties and nutritional quality upon long-term storage. Powdered product is shelf-stable and remains edible for a couple of years. However, storage temperature and condition are found to affect the physical and antioxidant properties of food powders.[Citation8,Citation9] To date, the effects of long-term storage on the physical properties and nutritional quality of PSP powder have not been reported elsewhere. This study was conducted to evaluate the effect of chilled (4 ± 2ºC) and ambient (25 ± 2ºC) temperatures on the physical properties, resistant starch content and antioxidant profile of PSP powder after long-term (12 months) storage.
Materials and methods
Materials
Well-matured, unblemished purple sweet potatoes (Ipomoea batatas ‘Chiran Murasaki’) were obtained from FAMA (Federal Agricultural Marketing Authority, Malaysia) after 105 days of plantation. The tubers were brought directly to the laboratory within one week of harvest and stored in a cool, ventilated area until use. Folin–Ciocalteu reagent, gallic acid and (+)(-) catechin were purchased from Sigma-Aldrich (St. Louis, MO, USA). Trolox and 2,4,6-tripyridyl-s-triazine (TPTZ) were obtained from Acros Organics (Morris, NJ, USA) while 2,2-diphenyl-1-picrylhydrazyl (DPPH) radical was from Merck (Darmstadt, Germany). Resistant starch assay kit was bought from Megazyme International (Bray, Ireland). All other chemicals used were of analytical grade.
Preparation of PSP powder
PSP powder was produced according to the optimized processing conditions previously determined by the same research group (data not shown). Briefly, unpeeled purple sweet potatoes (PSP) were washed, cut into cubes of 3 cm (l) x 3 cm (h) x 1.5 cm (w) and steamed for 30 min at a tuber to water ratio of 1:4 (w/v). Samples were cooled and mashed into a puree, then fed onto the heated trough of a double drum dryer (R. Simon Dryers Ltd., Nottingham, England) at fixed processing parameters of steam pressure = 3 bar and drum speed = 2 rpm. The dried flakes were collected, ground and sieved through a 425 µm mesh screen to produce PSP powder. The powder was vacuum packed into aluminium pouches with a thickness of 120 µm (predetermined as the most superior barrier for moisture, gas and light than any other polymeric materials), stored at chilled (4 ± 2ºC) and ambient (25 ± 2ºC) temperatures for 12 months before the physical properties, antioxidant profile and resistant starch content were assayed at 0, 3, 5, 7, 9, 11 and 12 months of storage.
Physical properties analyses of PSP powder
Moisture content
Moisture was determined by oven drying method. PSP powder (1.50 g) was accurately weighted in a clean, predried aluminium dish. The sample was placed overnight in an oven at 105ºC until a constant mass was obtained. The sample was cooled in a desiccator for 30 min prior to weighing.
Water activity (aw)
The water activity of the samples was determined using an automated aw meter (model: AquaLab 3TE meter, METER Group, Inc., Pullman, WA, USA).
Colour
The color attributes of the samples were analyzed using an Ultra Scan PRO spectrophotometer (Hunter Laboratories Inc., Reston, VA, USA). A sample weighing 3.0 g was placed in a 20-mm cell, and the Hunter values comprising of L (lightness), a (redness) and b (yellowness) were recorded using the total transmittance mode with illuminant D65. Total color difference (ΔE) was determined using the following equation:
where L, a, b were the color parameters for PSP powder after storage and L0, a0, b0 were color parameters for PSP powder before storage (L0 = 45.93, a0 = 15.81, b0 = −3.98).
Water solubility index (WSI) and water absorption capacity (WAC)
The water solubility index and water absorption capacity of samples were evaluated using the method described by Anderson.[Citation10] PSP powder weighing 0.50 g was mixed with 6.0 mL of distilled water and continuously stirred for 30 min in a water bath at 30ºC. Then, the solution was centrifuged at 3000 g for 10 min. The supernatant was dried at 105ºC for 4 h to obtain the dried solids mass. The mass of the wet residue obtained from the centrifugation was taken. The WSI and WAC were calculated using the following equations:
Resistant starch (RS) analyses in PSP powder
The resistant starch (RS), non-resistant starch (NRS) and total starch (TS) contents were analyzed using the resistant starch assay kit according to the protocol provided by Megazyme International.[Citation11] Basically, 0.1 g of PSP powder was mixed with 4.0 mL of pancreatic α-amylase solution (10 mg/mL) containing amyloglucosidase (3 U/mL). The samples were subsequently incubated at 37ºC with continuous shaking for 16 h. To aid in dispersion, 4.0 mL of 99% ethanol was added. This was followed by centrifugation at 1500 g for 10 min. The supernatants were drawn and kept for NRS analysis. The pellet was resuspended in 2.0 mL and washed in 6.0 mL of 50% ethanol, respectively, for RS determination. In contrast, the centrifuged washings were combined with the decanted supernatant for NRS determination.
Determination of resistant starch (RS)
The pellets collected from section 2.4 were resuspended with 2.0 mL of 2 M KOH. After a continuous stirring for 20 min in an ice bath, 8 mL of 1.2 M sodium acetate buffer (pH 3.8) and 0.1 mL of amyloglucosidase (3300 U/mL) was added. The mixture was placed in a water bath at 50ºC for 30 min, then centrifuged at 1500 g for 10 min. An aliquot of supernatant (0.1 mL) was treated with 3.0 mL of glucose oxidase-peroxidase (GOPOD) reagent and kept at 50ºC for 20 min. A blank (0.1 mL of 100 mM sodium acetate buffer pH 4.5 + 3.0 mL of GOPOD) and glucose standards (0.1 mL of D-glucose at 0.0–1.0 mg/mL + 3.0 mL of GOPOD), were incubated concurrently. The absorbance was read at 510 nm to calculate the RS content.
Non-resistant starch (NRS)
The supernatant obtained from section 2.4 was adjusted to 100 mL with 100 mM sodium acetate buffer (pH 4.5). The diluted aliquot (0.1 mL) was incubated with 10 μL of amyloglucosidase (300 U/mL) in 100 mM sodium maleate buffer (pH 6.0) at 50ºC for 20 min, followed by a second incubation with 3.0 mL of GOPOD reagent at 50ºC for another 20 min. The absorbance was measured at 510 nm to calculate NRS content. The total starch (TS) was calculated as the sum of NRS and RS fractions.
Antioxidant analyses of PSP powder
Total anthocyanin content (TAC)
TAC was measured following two previously described methods from Burgos et al.[Citation12] and Kim et al.[Citation13] with some modifications. PSP powder (1.0 g) was mixed with 15.0 mL of methanol, premixed with 1.0 M HCL at 75:25 (v/v). The mixture was stirred in the dark for 3 h and centrifuged at 1500 g for 10 min. The supernatant was collected and the absorbance was measured using a UV-vis spectrophotometer (model: Lambda-25, Perkin Elmer, Waltham, MA, USA) at 535 nm. TAC was calculated based on the following equation:
where A = absorbance, Mw = molecular weight of cyanidin-3glucoside chloride (C21H21ClO11, 484.84 Da), DF = dilution factor, ԑ = molar absorptivity (34300), W = sample mass (g).
Total phenolic content (TPC)
TPC was measured using the Folin Ciocalteu assay as described by Swain and Hillis.[Citation14] The sample (0.10 g) was extracted with 20.0 mL of 75% methanol, vortexed and centrifuged at 1500 g for 10 min. One milliliter of the extract or gallic acid solution (50–150 mg/L) was mixed with 5 mL of Folin-Ciocalteau’s reagent (prior diluted with water at 1:10, v/v). After 3 min, 4.0 mL of Na2CO3 solution (7.5%, w/v) was added. One milliliter of this mixture was diluted to 10.0 mL with distilled water and allowed to stand for another 2 h. The absorbance was measured at 760 nm. TPC was expressed as milligram of gallic acid equivalents per gram of sample (mg GAE/g).
Total flavonoid content (TFC)
Determination of TFC was conducted using the aluminium chloride colorimetric assay based on a previously reported method described by Ardekani et al.[Citation15] The sample (0.10 g) was mixed with 3.0 mL of methanol and centrifuged at 1500 g for 10 min. One milliliter of sample or catechin solution (0, 50, 100, 150, 200, 250 and 300 mg/L) was mixed with 4.0 mL of deionized water. Then, 0.3 mL of NaNO2 (5% w/v) and 0.3 mL of AlCl3 (10% w/v) were added and allowed to stand for 5 min. Following this, 2.0 mL of 1 M NaOH was added, and the mixture was made up to 10.0 mL using deionized water. The absorbance was measured at 510 nm. TFC was expressed as milligram of catechin equivalents per gram of sample (mg CE/g).
DPPH radical scavenging activity
The DPPH scavenging activity was analyzed according to the procedure from Brand-Williams et al.[Citation16] The sample (0.10 g) was mixed with 3.0 mL of methanol and centrifuged at 660 g for 5 min. The supernatant (0.1 mL) or trolox solution (0–500 μM) was incubated with 3.9 mL of 80 μM methanolic DPPH solution for 3 h before measuring the absorbance at 517 nm. Results were expressed as μmol trolox equivalents per gram of sample (μmol TE/g).
Ferric reducing antioxidant power (FRAP)
FRAP was assayed following the method established by Benzie and Strain[Citation17] with some modifications. FRAP reagent was freshly prepared by mixing 300 mM acetate buffer (pH 3.6), 10 mM 2,4,6-tripyridyl-s-triazine (TPTZ) in 40 mM HCl and 20 mM FeCl3•6H2O in a volume ratio of 10:1:1 and warmed to 37ºC prior to analysis. PSP powder (0.10 g) was mixed with 3.0 mL of methanol and centrifuged at 660 g for 5 min. The supernatant (0.3 mL) or trolox solution (0–500 μM) was mixed with 2.7 mL of the freshly prepared FRAP reagent and allowed to stand for 10 min before reading the absorbance at 593 nm. Results were expressed in μmol trolox equivalents per gram of sample (μmol TE/g).
Statistical analysis
PSP powder was produced in two different batches (replicate) in storage study, and all analytical measurements (physical properties, resistant starch content and antioxidant properties) were performed in triplicates. Results were expressed as mean ± standard deviations (SD). The data were subjected to analysis of variance (ANOVA), and the differences were analyzed using Tukey’s test at p ≤ 0.05 using Minitab software (Version 14, State College, Philadelphia, USA).
Results and discussion
Physical properties of PSP powder after 12 months of storage
The physical properties of PSP powder, namely moisture content, aw, color, water absorption capacity (WAC) and water solubility index (WSI), are depicted in . Moisture content and aw are measured because water can accelerate microbiological or chemical deterioration during storage. After 12 months of storage at different temperatures, the moisture content increased significantly from 3.21% to 8.10% and 6.35%, for samples stored at 4ºC and 25ºC, respectively, while the aw increased significantly from 0.49 to 0.54, only for sample stored at 4ºC. These observations indicated that PSP powder stored at chilled temperature absorbed moisture more rapidly, and thus contained a higher amount of free water, than that stored at ambient temperature. Even though the moisture content increased significantly after 12 months, it was within the maximum acceptable limit of 15% moisture for flour products, as required by U.S. Food and Drug Administration (revised as of April 1, 2018).[18] Also, aw < 0.60 for most dried products is tolerable, as osmophilic yeasts, and few molds are generally inhibited at this range of aw.[Citation19] In terms of color, all samples had +a values (redness) and – b values (blueness). These color parameters represented the purple hue that objectively described the attractive purplish appearance of the powder ().
Table 1. Physical properties of purple sweet potato powder before and after 12 months of storage at chilled and ambient temperatures
Figure 1. The appearance of purple sweet potato powder (a) before and after 12 months of storage at (b) 4ºC and (c) 25ºC
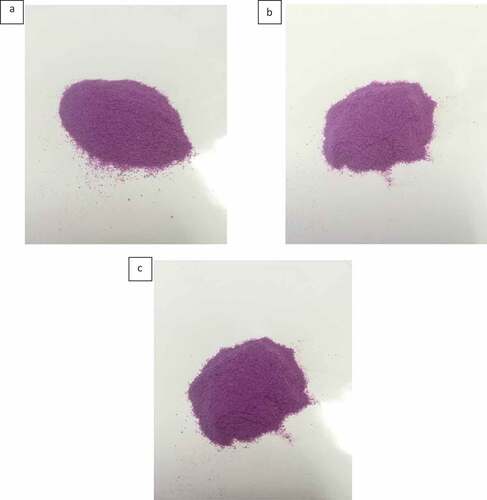
Both WAC and WSI are estimations of a product’s behavior upon further processing.[Citation20] Thus these parameters were measured in PSP powder, since it is used as soup/sauce thickener and ingredient for desserts and bakeries, which is meant to be further processed before consumption. WAC increased significantly for sample stored at 25ºC, but no significant difference was detected for sample stored at 4ºC. However, irrespective of storage temperature, all samples depicted superior WAC quality, even surpassing potato starch that is well known for its extraordinary WAC of 752%.[Citation21] The high WAC of PSP powder allows it to act as an excellent food thickener among diverse starches. Meanwhile, WSI depicted significant reduction at 25ºC but not at 4ºC, indicating that WSI of PSP powder was adversely affected when stored at ambient temperature, because a flour product with high WSI is always preferable as it indicates good starch solubility and digestibility.[Citation22] Even though the WSI reduced in PSP powder stored at 25ºC, it remained comparable to that of wheat-guava blend extrudate (WSI = 18.4–23.0%) and yam-rice-corn flour snack (WSI = 18.1–30.4%) as reported by Gandhi and Singh[Citation23] and Seth et al.[Citation24], respectively. Based on these observations, the physical quality of PSP powder is considered stable throughout 12 months of storage.
Resistant starch content of PSP powder after 12 months of storage
Resistant starch (RS) is defined as the starch fraction that resists amylolytic digestion in small intestine and reaches the large intestine intact, serving as an ideal substrate for colon microbial fermentation that produces various health-promoting byproducts, including short-chain fatty acid that prevents the growth of cancerous colonic cells.[Citation25] Besides, RS could reduce glycaemic response for managing diabetes,[Citation26] enhance satiety and reduce appetite for weight watchers[Citation27] and treat chronic kidney disease.[Citation28] These beneficial effects on human health has lead RS to becoming a popular functional food ingredient.[Citation29]
The RS, non-RS and total starch content in PSP powder before and after 12 months of storage are shown in . While non-RS and total starch remained unchanged after 12 months, the RS content reduced significantly at both 4ºC and 25ºC. This implies that the amount of RS reduces with increasing storage time, irrespective of storage temperature. Similarly, showed that RS content is negatively correlated with storage time, recording linear correlations of R2 = 0.86 and R2 = 0.93, for samples stored at 4ºC and 25ºC, respectively. Chilled sample contained a higher amount of RS than ambient sample, possibly due to the higher rate of starch reassociation and recrystallization at chilled temperature that facilitates RS formation.[Citation30] This observation is in line with the findings from Sullivan et al.[Citation31], who observed that RS formation is greater in white bread stored at chilled temperature than that stored at frozen (−17ºC) or ambient (20ºC) temperatures.
Table 2. Resistant starch content of purple sweet potato powder before and after 12 months of storage at chilled and ambient temperatures
Figure 2. Changes in resistant starch content (mg/100 g) for purple sweet potato powder stored over 12 months at 4ºC and 25ºC
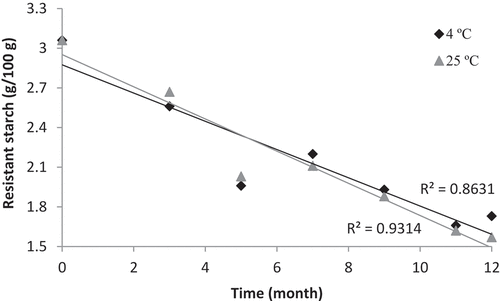
The reduction in RS over time could be explained by: (i) the increment in moisture content during storage and (ii) the high sugar content in PSP powder. According to Franco et al.[Citation32], corn starch is more susceptible to α-amylase and amyloglucosidase hydrolysis at high moisture level than at low moisture level. This indicates that, when moisture content increases, larger amount of starch could be hydrolyzed to result in the reduction of overall RS content. Also, Platel and Shurpalekar[Citation33] noticed that dry heat treatment resulted in higher RS content in tubers and legumes than did wet heat treatment. It is deduced that low moisture is more favorable to increase RS formation than high moisture condition. From , it is clear that the moisture content of PSP powder stored at both chilled and ambient temperatures increased significantly during storage; thus, the RS content in both samples dropped. The reduction in RS might also be due to the presence of naturally occurring sugars in PSP. Sweet potato, as its name suggests, contains a considerable amount of simple sugars (sucrose, glucose, fructose) which contributes to the natural sweetness of the tuber. These soluble sugars act as anti-plasticizer and interact with neighboring starch polymers in a way that interferes the crystallization process among starch molecules, thus modifying the matrix of gelatinized starch and reduces the RS content.[Citation34]
Antioxidant profile of PSP powder after 12 months of storage
The antioxidant profile of PSP powder after 12 months of storage at 4ºC and 25ºC are shown in . An interesting observation was made whereby the total anthocyanin content (TAC) increased significantly irrespective of storage temperature, from 121.71 mg/100g (control) to 197.61 mg/100g (4ºC sample) and 185.33 mg/100g (25ºC sample) after 12 months. This phenomenon could be explained by two processes that take place gradually in the PSP powder, namely, copigmentation and self-association. Copigmentation is the non-covalent π-π interaction between the B ring of free anthocyanin molecules and a planar B ring of a suitable colorless copigment, usually a phenolic compound[Citation35] or other molecules, such as alkaloids, amino acids, organic acids and polysaccharides.[Citation36] During copigmentation, the oxidation and polymerization reactions between anthocyanin and free phenolic compounds occur at a slow rate.[Citation37] The participation of phenolics to form copigments partially explain the reduction in total phenolic content for 4ºC sample, as detected after 12 months of storage. Meanwhile, self-association is the hydrophilic interaction among anthocyanin molecules themselves. It takes place at the glucose moieties of the involved molecules and is stabilized through the hydrophobic repulsion between the anthocyanins’ aromatic nuclei.[Citation35,Citation38] Even though both processes involve different mechanisms, they improve the stability of weak complexes of monomeric anthocyanins to form new anthocyanin products with higher stability, which contributed to the increment in TAC.
Table 3. Antioxidant properties of purple sweet potato powder before and after 12 months of storage at chilled and ambient temperatures
The antioxidant activity of PSP powder, i.e., the DPPH scavenging activity and FRAP assay, decreased significantly after 12 months of storage despite the increment in total anthocyanin and flavonoid contents (). These findings are similar to works reporting on the antioxidant activities of different food products upon long-term storage. Tsai et al.[Citation39] observed a reduction in FRAP for mulberry wine stored for 12 months; Muzzaffar et al.[Citation40] observed a decrease in FRAP and DPPH scavenging activity for pumpkin candy stored for 3 months; while Davies et al.[Citation41] noticed a reduction in DPPH scavenging activity in orange vinegar after 6 months of storage. Based on these findings, it can be concluded that, the drop in antioxidant activity in food powders is inevitable after long-term storage, irrespective of storage temperatures.
Correlation analysis for TAC vs. DPPH
shows the correlation between antioxidant content (total anthocyanin content, TAC) and antioxidant activity (DPPH scavenging) of PSP powder stored for 12 months, at 4ºC and 25ºC, respectively. TAC and DPPH are well correlated in both samples, showing R2 = 0.97 for 4ºC sample and R2 = 0.96 for 25ºC sample. TAC and DPPH depict a negative correlation, i.e., increment in TAC did not contribute to a stronger DPPH scavenging activity. This is due to the formation of larger, bulkier polymeric anthocyanin compounds via copigmentation and self-association over time, which creates steric hindrance when approaching radical substances, causing limited access of anthocyanins on the reactive sites,[Citation42] therefore lowering the scavenging ability of anthocyanins to exert antioxidant activity. This finding indicates that, for food powder that has been stored for long-term, the amount of antioxidant compounds does not always reflect its antioxidant activity. In the case of PSP powder, even though TAC increases, the antioxidant activity reduces. Measurement of antioxidant activity along with antioxidant content is advisable in order to reflect a product’s overall antioxidant capacity. It is also noteworthy that the storage temperature did not affect the reduction of antioxidant activity in the product. PSP powder kept at refrigerated temperature experienced a reduction in antioxidant activity (), indicating that chilled storage has insignificant effect in preventing the loss of antioxidant activity for a long stored powder product. While chilled temperatures could slow down the degradation rate of antioxidant potency of PSP powder, the temperature is insufficient to prevent activity loss when the product is stored over a long period of time (12 months). In any food product, quality degradation over time is inevitable.
Conclusion
Current study evaluates the effects of 12-month storage, at chilled and ambient temperatures, on the physical properties, RS and antioxidant qualities of PSP powder. While results showed that the physical properties of PSP powder remained stable throughout the study period, the RS content andantioxidant activities were negatively affected by storage time and temperature, despite the increment in total anthocyanin content. Antioxidant content is not always positively correlated with the antioxidant activity for a long stored food powder, thus measuring both is advisable to get an accurate estimation of the overall antioxidant capacity. Furthermore, 12-month storage of PSP powder, at refrigerated temperature, observed a reduction in RS content and antioxidant activity, suggesting that chilled storage has an insignificant effect on preventing the loss of RS and antioxidant activity in food powders that are stored for long term.
Acknowledgments
The financial support from UPM Research University Grant Scheme, Malaysia (RUGS project no. 9396000) and Indonesia Endowment Fund for Education, Indonesia (LPDP project no. 20130752020004) are gratefully acknowledged. All authors declare no conflict of interest.
Additional information
Funding
References
- Ternes, T.; The Okinawan sweet potato: A purple powerhouse of nutrition. 2011 (accessed 17 July 2018)
- Khoo, H. E.; Azlan, A.; Tang, S. T.; Lim, S. M. Anthocyanidins and Anthocyanins: Colored Pigments as Food, Pharmaceutical Ingredients, and the Potential Health Benefits. Food Nutr. Res. 2017, 61(1), 1361779.
- Cai, Z.; Song, L.; Qian, B.; Xu, W.; Ren, J.; Jing, P.; Oey, I. Understanding the Effect of Anthocyanins Extracted from Purple Sweet Potatoes on Alcohol-Induced Liver Injury in Mice. Food Chem. 2018, 245, 463–470.
- Jang, H. H.; Kim, H. W.; Kim, S. Y.; Kim, S. M.; Kim, J. B.; Lee, Y. M. In Vitro and in Vivo Hypoglycemic Effects of Cyanidin 3-Caffeoyl-P-Hydroxybenzoylsophoroside-5-Glucoside, an Anthocyanin Isolated from Purple-Fleshed Sweet Potato. Food Chem. 2019, 272, 688–693.
- Van Toan, N.; Vu Quynh Anh, N. Preparation and Improved Quality Production of Flour and the Made Biscuits from Purple Sweet Potato. J. Food Nutr. 2018, 4, 1–14.
- Hutasoit, M.; Julianti, E.; Lubis, Z., Effect of pretreatment on purple-fleshed sweet potato flour for cake making, in IOP Conference Series: Earth and Environmental Science (International Conference on Agriculture, Environment, and Food Security). 2017: Medan, Indonesia.
- Zhang, L.; Zhao, L.; Bian, X.; Guo, K.; Zhou, L.; Wei, C. Characterization and Comparative Study of Starches from Seven Purple Sweet Potatoes. Food Hydrocolloids. 2018, 80, 168–176.
- Breda, C. A.; Sanjinez-Argandoña, E. J.; Correia, C. Shelf Life of Powdered Campomanesia Adamantium Pulp in Controlled Environments. Food Chem. 2012, 135(4), 2960–2964.
- Fracassetti, D.; Del Bo’, C.; Simonetti, P.; Gardana, C.; Klimis-Zacas, D.; Ciappellano, S. Effect of Time and Storage Temperature on Anthocyanin Decay and Antioxidant Activity in Wild Blueberry (Vaccinium Angustifolium) Powder. J. Agric. Food Chem. 2013, 61(12), 2999–3005.
- Anderson, R.;. Gelatinization of Corn Grits by Roll-And Extrusion-Cooking. Cereal Sci. Today. 1969, 14, 4–12.
- Megazyme International. Resistant Starch Assay Procedure: AOAC Method 2002.02 And AACC Method 32-40.01; Megazyme International Ireland: Wicklow, Ireland, 2011.
- Burgos, G.; Amoros, W.; Muñoa, L.; Sosa, P.; Cayhualla, E.; Sanchez, C.; Díaz, C.; Bonierbale, M. Total Phenolic, Total Anthocyanin and Phenolic Acid Concentrations and Antioxidant Activity of Purple-Fleshed Potatoes as Affected by Boiling. J. Food Compost. Anal.. 2013, 30(1), 6–12.
- Kim, J. M.; Park, S. J.; Lee, C. S.; Ren, C. S.; Kim, S. S.; Shin, M. Functional Properties of Different Korean Sweet Potato Varieties. Food Sci. Biotechnol. 2011, 20(6), 1501–1507.
- Swain, T.; Hillis, W. The Phenolic Constituents of Prunus Domestica L.—The Quantitative Analysis of Phenolic Constituents. J. Sci. Food Agric.. 1959, 10(1), 63–68.
- Ardekani, M. R. S.; Hajimahmoodi, M.; Oveisi, M. R.; Sadeghi, N.; Jannat, B.; Ranjbar, A. M.; Gholam, N.; Moridi, T. Comparative Antioxidant Activity and Total Flavonoid Content of Persian Pomegranate (Punica Granatum L.) Cultivars. Iran. J. Pharm. Res. World. 2011, 10(3), 519.
- Brand-Williams, W.; Cuvelier, M.-E.; Berset, C. Use of a Free Radical Method to Evaluate Antioxidant Activity. LWT Food Sci. Technol. 1995, 28(1), 25–30.
- Benzie, I. F.; Strain, J. J. The Ferric Reducing Ability of Plasma (FRAP) as a Measure of “Antioxidant Power”: The FRAP Assay. Anal. Biochem. 1996, 239(1), 70–76.
- Code of Federal Regulations Title 21– Food and Drugs: Subchapter B–Food for Human Consumption: Subpart B–Requirements for Specific Standardized Cereal Flours and Related Products, in 21. 1977, U.S. Food and Drug Administration: Maryland, USA.
- Fontana, A. J.;. Understanding the Importance of Water Activity in Food. Cereal Foods World. 2000, 45(1), 7–10.
- Oikonomou, N.; Krokida, M. Water Absorption Index and Water Solubility Index Prediction for Extruded Food Products. Int. J. Food Prop. 2012, 15(1), 157–168.
- Suresh, C.;. Assessment of Functional Properties of Different Flours. Afr. J. Agric. Res. 2013, 8(38), 4849–4852.
- Yousf, N.; Nazir, F.; Salim, R.; Ahsan, H.; Sirwal, A. Water Solubility Index and Water Absorption Index of Extruded Product from Rice and Carrot Blend. J. Pharmacogn. Phytochem. 2017, 6(6), 2165–2168.
- Gandhi, N.; Singh, B. Study of Extrusion Behaviour and Porridge Making Characteristics of Wheat and Guava Blends. J. Food Sci. Technol. 2015, 52(5), 3030–3036.
- Seth, D.; Badwaik, L. S.; Ganapathy, V. Effect of Feed Composition, Moisture Content and Extrusion Temperature on Extrudate Characteristics of Yam-Corn-Rice Based Snack Food. J. Food Sci. Technol.. 2015, 52(3), 1830–1838.
- Topping, D. L.; Clifton, P. M. Short-Chain Fatty Acids and Human Colonic Function: Roles of Resistant Starch and Nonstarch Polysaccharides. Physiol. Rev. 2001, 81(3), 1031–1064.
- EFSA. Panel on Dietetic Products Nutrition and Allergies, Scientific Opinion on the Substantiation of a Health Claim Related to “Native Chicory Inulin” and Maintenance of Normal Defecation by Increasing Stool Frequency Pursuant to Article 13.5 Of Regulation (EC) No 1924/20061. Efsa J. 2015, 13, 3951.
- Clark, M. J.; Slavin, J. L. The Effect of Fiber on Satiety and Food Intake: A Systematic Review. J. Am. Coll. Nutr. 2013, 32(3), 200–211.
- Sirich, T. L.; Plummer, N. S.; Gardner, C. D.; Hostetter, T. H.; Meyer, T. W. Effect of Increasing Dietary Fiber on Plasma Levels of Colon-Derived Solutes in Hemodialysis Patients. Clin. J. Am. Soc. Nephrol. 2014, 9(9), 1603–1610.
- Lockyer, S.; Nugent, A. Health Effects of Resistant Starch. Nutr. Bull.. 2017, 42(1), 10–41.
- Aguirre, J. F.; Osella, C. A.; Carrara, C. R.; Sánchez, H. D.; Buera, M. D. P. Effect of Storage Temperature on Starch Retrogradation of Bread Staling. Starch‐Stärke. 2011, 63(9), 587–593.
- Sullivan, W. R.; Hughes, J. G.; Cockman, R. W.; Small, D. M. The Effects of Temperature on the Crystalline Properties and Resistant Starch during Storage of White Bread. Food Chem. 2017, 228, 57–61.
- Franco, C. M.; Ciacco, C. F.; Tavares, D. Q. Effect of the Heat‐Moisture Treatment on the Enzymatic Susceptibility of Corn Starch Granules. Starch‐Stärke. 1995, 47(6), 223–228.
- Platel, K.; Shurpalekar, K. Resistant Starch Content of Indian Foods. Plant Foods Human Nutr. 1994, 45(1), 91–95.
- Sajilata, M. G.; Singhal, R. S.; Kulkarni, P. R. Resistant starch–A Review. Compr. Rev. Food Sci. Food Saf. 2006, 5(1), 1–17.
- González-Manzano, S.; Santos-Buelga, C.; Dueñas, M.; Rivas-Gonzalo, J. C.; Escribano-Bailón, T. Colour Implications of Self-Association Processes of Wine Anthocyanins. Eur. Food Res. Technol. 2008, 226(3), 483–490.
- Mazza, G.; Brouillard, R. The Mechanism of Co-Pigmentation of Anthocyanins in Aqueous Solutions. Phytochemistry. 1990, 29(4), 1097–1102.
- Escribano-Bailon, T.; Santos-Buelga, M. ;. C. Anthocyanin Copigmentation-Evaluation, Mechanisms and Implications for the Colour of Red Wines. Curr. Org. Chem. 2012, 16(6), 715–723.
- He, F.; Liang, N. N.; Mu, L.; Pan, Q. H.; Wang, J.; Reeves, M. J.; Duan, C. Q. Anthocyanins and Their Variation in Red Wines II. Anthocyanin Derived Pigments and Their Color Evolution. Molecules. 2012, 17(2), 1483–1519.
- Tsai, P. J.; Huang, H. P.; Huang, T. C. Relationship between Anthocyanin Patterns and Antioxidant Capacity in Mulberry Wine during Storage. J. Food Qual. 2004, 27(6), 497–505.
- Muzzaffar, S.; Baba, W. N.; Nazir, N.; Masoodi, F.; Bhat, M. M.; Bazaz, R. Effect of Storage on Physicochemical, Microbial and Antioxidant Properties of Pumpkin (Cucurbita Moschata) Candy. Cogent Food Agric. 2016, 2(1), 1163650.
- Davies, C. V.; Gerard, L. M.; Ferreyra, M. M.; Schvab, M. D. C.; Solda, C. A. Bioactive Compounds and Antioxidant Activity Analysis during Orange Vinegar Production. Food Sci. Technol. 2017, 37(3), 449–455.
- Prior, R. L.; Wu, X.; Schaich, K. Standardized Methods for the Determination of Antioxidant Capacity and Phenolics in Foods and Dietary Supplements. J. Agric. Food Chem. 2005, 53(10), 4290–4302.