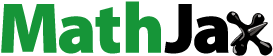
ABSTRACT
This study aimed to identify angiotensin I-converting enzyme (ACE) inhibitory peptides in enzymatic hydrolysates from pork loin. Pork hydrolysates were produced by injecting different enzymes to the loins as follows: un-injected pork (fresh pork), injected with water (no enzyme control), 100 ppm protease type XIII (E1), 80 ppm thermolysin (E2), and the combination of 100 ppm protease type XIII and 80 ppm thermolysin (E3). The highest degree of hydrolysis (96.7%) and ACE inhibitory activity (90.1%) were observed in the E2-treated hydrolysate. Next, the E2-treated hydrolysate was fractionated and purified by using two different chromatographic methods, and the fractions with the highest ACE inhibitory activity were selected at each step. Finally, two peptides (Leu-Val-Gly-Arg-Pro-Arg-His-Gly-Gln and Val-Phe-Pro-Ser) were identified in the E2-treated hydrolysate using UPLC-QTOF-MS/MS. The smaller peptide, Val-Phe-Pro-Ser, showed a lower IC50 value for ACE inhibitory activity than the nona-peptide and captopril, a commercial ACE inhibitor. The results of this study suggest that the isolated nona-peptide, Leu-Val-Gly-Arg-Pro-Arg-His-Gly-Gln, possessed strong ACE inhibitory activity. Therefore, overnight incubation of fresh pork in a refrigerator after thermolysin injection may make the pork more beneficial for human consumption.
Graphical Abstract
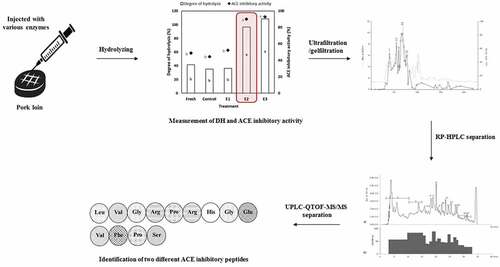
Introduction
The renin-angiotensin system plays a major role in cardiovascular homeostasis as regulating blood pressure.[Citation1] The system starts by converting angiotensinogen to the prehypertensive hormone angiotensin I via renin, which is secreted by the kidneys.[Citation2] Angiotensin I converting enzyme (ACE) induces conversion of decapeptide angiotensin I (DRVYIHPFHL) into angiotensin II (DRVYIHPF) which is the active form of the hormone. The ACE, Zn4+ metallopeptidase, removes the C-terminal dipeptide from the angiotensin I to produce the potent angiotensin II and, at the same time, inactivate the vasodilator bradykinin (RPPGFSPFR) or the encephalitis.[Citation3] Captopril, which was first isolated from snake venom, was developed as an ACE inhibitor with an IC50 of 2.3 × 10−8 M.[Citation4,Citation5] However, the use of captopril has various side effects, such as rashes and a loss of taste.[Citation6] Therefore, many researchers have sought to isolate naturally occurring ACE inhibitors with little or no side effects from muscle and plant proteins.[Citation7–Citation10]
To improve the tenderness and juiciness of fresh meat, injection of solutions containing salt, phosphate, and additional flavor components is commonly used as a marination process.[Citation11] This method induces relatively rapid enhancements in sensory qualities and other functionalities. Recently, Seol et al.[Citation9] showed that injection (80 ppm) of Hanwoo beef with a proteolytic enzyme (thermolysin) and storage at 5°C for 3 days generated potent bioactive peptides with various activities, including ACE inhibitory and antioxidative activities. Thus, injection of meat with proteolytic enzymes could satisfy consumers’ sensory and health-promoting expectations. To our knowledge, no studies have isolated ACE inhibitory peptides from proteolytic enzyme-injected pork. Consequently, the purpose of the present study was to determine the effect of injecting different types of proteolytic enzymes into pork loin during cold storage on the generation of ACE inhibitory peptides.
Materials and methods
Reagents
ACE (from rabbit lung), hippuryl-L-histidyl-L-leucine (HHL), protease type XIII (P2143), and thermolysin (T7902) were purchased from Sigma (St. Louis, MO, USA). Acetonitrile (LC-MS grade) was obtained from Roth GmbH (Karlsruhe, Germany).
Meat sample preparation
Thirty loins (M. longissimus) were obtained within 24 h of slaughter from 30 pigs at a slaughterhouse. The pork loins were transferred to the laboratory in a cooler containing ice packs. The pork loins (each 100 g) were then left untreated, injected with water, or injected with two different enzymes either alone or in combination as follows: (1) fresh pork (no injection), (2) distilled water (no enzyme), (3) 100 ppm protease type XIII, (4) 80 ppm thermolysin; (5) 100 ppm protease type XIII and 80 ppm thermolysin. The types and concentrations of the enzymes used were determined based on studies by Choe et al.[Citation1] and Jang et al.[Citation12] After injection, the loins were stored at 5°C for 24 h to induce degradation of the muscular proteins via enzymatic proteolysis.
Extraction of crude peptide
Peptides were extracted using the modified peptide extraction method of Choe et al.[Citation1] Briefly, a 5 g pork sample was homogenized in 20 mL of distilled water and incubated in a 90°C water bath for 20 min to denature enzymes. Then, the mixture was filtered and centrifuged at 10,000 × g for 20 min. The supernatant was collected and used as a crude peptide extract (CPE) for measuring the degree of hydrolysis (DH) and ACE inhibitory activity.
Measurement of the degree of hydrolysis (DH)
The protein content of each extract was measured using a protein assay kit (Sigma), and bovine serum albumin was used as the standard. The DH was determined by measuring the amount of nitrogen dissolved in 10% trichloroacetic acid (TCA). Equal volume of 10% TCA was added to the CPE and reacted at room temperature for 30 min. After centrifugation at 3,290 × g for 10 min, the nitrogen content of supernatant was measured using a protein assay kit and the DH was calculated as follows:
ACE inhibitory activity
ACE inhibitory activity was assessed by spectrophotometric method according to Choe et al.[Citation1] The IC50 value was determined by measuring the ACE inhibitory activity and peptide concentration of each extract after regression analysis.
where S is the O.D. of the sample, S.C. is the O.D. of the sample control, B is the O.D. of the blank, and B.C. is the O.D. of the blank control.
Ultra-filtration system
High molecular weight peptides (>10 kDa) were removed by ultra-filtration at 4°C using a PM-10 membrane (MWCO 10,000 Da; Amicon, Beverly, MA, USA). To further separate the low molecular weight peptides (<3 kDa), the filtrates were centrifuged at 4,000 × g for 45 min using an Ultracel 3K membrane (MWCO 3,000 Da; Amicon). The separated CPE was subsequently lyophilized and stored in a deep-freezer (−74°C) until measurement of ACE inhibitory activity or further separation.
Gel-filtration system
The ultra-filtration extracts were filtered again through a Millipore membrane filter (0.45 μm) and applied to a column (Φ25 × 1,200 mm; Amersham Pharmacia Biotech) containing saturated P-2 resin (eluent, distilled-deionized water; flow rate, 0.8 mL/min). The fractions were collected at 15-min intervals with a fraction collector (Amersham Pharmacia Biotech), and the absorbance at 220 nm of every fraction was measured to confirm the peptide concentration. Fractions containing peptides of similar molecular weights were pooled, lyophilized, and their ACE inhibitory activity was measured. The fraction with the highest ACE-inhibiting activity was further purified by RP-HPLC.
Reverse-phase high-performance liquid chromatography (RP-HPLC)
The gel-filtration fraction that showed the highest ACE inhibition activity was further purified RP-HPLC (PU-980; Jasco, Tokyo, Japan). The selected gel-filtration fraction was lyophilized again and re-dissolved in water containing 1% trifluoroacetic acid (TFA). Then, a 20 μL aliquot of the solution was applied to a Vydac C18 reverse-phase column (4.5 × 25 cm, 5 Å, 300 μm pore size) for further purification. The binary gradient elution system consisted of 0.1% TFA in DW (A) and 0.1% TFA in acetonitrile (B). The elution conditions were optimized as follows: a linear gradient from 0% to 12% B (0–5 min), 27% B (6–30 min), 99% B (31–35 min), and 12% B (36–240 min) (flow rate, 1.0 mL/min; injection volume, 20 μL). An ultraviolet (UV) detector (UV-975; Jasco, Tokyo, Japan) was operated at 220 nm. Twelve peak fractions were collected and concentrated using a speed-vac, and ACE-inhibitory activity was measured. The peak fraction with the highest ACE inhibition was applied to an ultra-performance liquid chromatography/quadrupole time-of-flight mass spectrometry (UPLC-QTOF-MS/MS) system to analyze the amino acid sequence of the peptides in the fractions.
Profiling of small molecular peptides using ultra-performance liquid chromatography (UPLC)
A UPLC system (Waters, Milford, MA, USA) equipped with a binary solvent delivery system, autosampler, and photodiode array (PDA) detector was used to analyze the peptides. A UPLC system equipped with an Acquity UPLC BEH C18 column (2.1 × 100 mm, 1.7 μm; Waters) was equilibrated with water containing 0.1% TFA. Then, the HPLC fraction (5 μL) was injected into the column and eluted with a gradient of acetonitrile containing 0.1% TFA (flow rate, 0.35 mL/min; running time, 12 min). The absorbance of the eluate at 214 nm was detected by a PDA detector and QTOF-MS/MS.
Sequence analysis of pork-derived peptides by UPLC/QTOF-MS/MS
Peptides separated by C18-UPLC were analyzed and assigned by a QTOF-MS/MS (Waters) attached to the UPLC system. The Q-TOF mass spectrometer was operated under positive electrospray ionization (ESI) mode with a source temperature of 100°C. Condition of UPLC/QTOF-MS/MS and sequence analysis were followed as described by Choe et al.[Citation1] The peptide sequencing was performed using the Biolynx software by analyzing ion peaks in the m/z range of 50 to 1,000.
Chemical synthesis of the peptides
Chemical synthesis of the identified peptides was examined using a PenptrEXTM automatic peptide synthesizer and lyophilized to confirm their identities and ACE inhibitory activities.
Statistical analysis
The flow chart for the entire procedure from isolation to the identification of ACE inhibitory peptides is shown in . The entire experiment was replicated three times with two observations per replication. Statistical analyses were performed using the SAS statistical program (version 9.3, SAS Institute, Cary, NC, USA). Significant differences among the treatments were analyzed using general linear model (GLM) with Duncan’s multiple range test at a level of P < .05.
Results and discussion
DH and ACE inhibitory activities of CPE from pork loin injected with various enzymes
The type of enzyme injected influenced the DH in CPE derived from the treated pork loin (). The CPE generated by the E2 and E3 treatments had higher (P < .05) DH than the other CPE. There were no significant differences in the DH between the E1-derived CPE and the control (injected with water only). The control showed a similar DH (P > .05) to that of fresh pork (no injection). These results showed that the enzyme activity in the pork loins depends on the type of enzyme injected. This result corresponds with the findings of a previous study by Seol et al.[Citation13], which exhibited a significantly higher DH in Hanwoo loin samples treated with thermolysin or a combination of protease type XIII and thermolysin compared to the protease type XIII-injected sample. Protein substrates with a greater DH contained lower molecular weight peptides and higher ACE inhibitory activity.[Citation14] A relationship between the DH and ACE inhibitory activity was observed in the previous study.[Citation13] This is consistent with the results of the present study, as we observed that the CPE with a higher DH exhibited higher ACE inhibitory activity (). In other words, the CPE from the E2 or E3 treatment showed significantly higher ACE inhibitory activities than the CPE with the lower DH. However, the ACE inhibitory activity of the CPE released by E3 treatment was slightly higher (P > .05) than that of the CPE released by E2 treatment, namely, there was no synergetic effect of thermolysin and protease type XIII treatment. Therefore, the E2 CPE was selected for further purification and identification of the ACE inhibitory peptides.
Table 1. Degree of hydrolysis and ACE inhibitory activity of crude peptide extracts derived from pork loin injected with various enzymes
Size exclusion chromatography
The CPE generated by E2 treatment was further purified by using a 3K membrane and a P-2 gel-filtration column, and the peptide content was measured using spectrophotometer at 220 nm. Fractions of the E2-derived CPE purified from the P-2 gel-filtration column with a similar molecular weight were pooled and ACE inhibitory activity was measured, and four fractions (I–IV) exhibited relatively higher activity than the others (). In particular, fraction III (E2-FIII) showed 82.3% relative ACE inhibitory activity; therefore, it was selected for further separation by RP-HPLC. This result corresponded to the results of a previous study[Citation12] in which four gel-filtration fractions with ACE inhibitory activity had an optimal molecular weight of 3 kDa.
Isolation and identification of separated ACE inhibitory peptides
The gel-filtration fraction with high ACE inhibitory activity (E2-FIII) was separated by RP-HPLC. The major peak fractions from the RP-HPLC (1–17) were pooled and concentrated, and ACE inhibitory activity was measured. The peptide patterns in the E2-FIII fractions and the ACE inhibitory activity of each peak are shown in . A peak with a medium retention time exhibited high ACE inhibitory activity, and this result agreed with the findings of Megías et al.[Citation15], who reported that peptides rich in hydrophobic amino acids with medium-high retention times on a C18 reverse-phase column exhibited ACE inhibitory activity.
Figure 3. (a) RP-HPLC profile and (b) ACE inhibitory activity of fraction E2-FIII. Peaks were divided into 17 groups (1–17), and their ACE inhibitory activity was measured
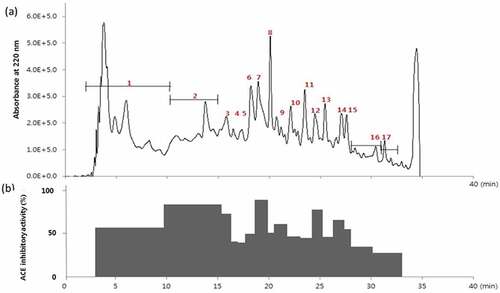
To determine the amino acid sequence of the ACE inhibitory peptides, the most potent peak (E2-FIII-P7), which showed significantly high ACE inhibitory activity (89%, ), was analyzed by LC-MS/MS. Based on the MS/MS spectrum, all the peptides in E2-FIII-P7 were identified by de novo sequencing. Two candidate peptides were identified in the thermolysin-injected pork loin sample E2-FIII-P7, Leu-Val-Gly-Arg-Pro-Arg-His-Gly-Gln, and Val-Phe-Pro-Ser ().
Figure 4. UPLC-QTOF-MS/MS analysis. Peptide with the sequence [(a) Leu-Val-Gly-Arg-Pro-Arg-His-Gly-Gln and (b) Val-Phe-Pro-Ser] of E2-FIII-P7
![Figure 4. UPLC-QTOF-MS/MS analysis. Peptide with the sequence [(a) Leu-Val-Gly-Arg-Pro-Arg-His-Gly-Gln and (b) Val-Phe-Pro-Ser] of E2-FIII-P7](/cms/asset/44c28864-8dd6-4369-b470-b25252596088/ljfp_a_1629690_f0004_oc.jpg)
To verify the ACE inhibitory activity of the isolated peptides, they were chemically synthesized, and the IC50 was determined. The relative ACE inhibitory IC50 values were as follows: Val-Phe-Pro-Ser > captopril (positive control) ≥ Leu-Val-Gly-Arg-Pro-Arg-His-Gly-Gln (). In other words, the nona-peptide Leu-Val-Gly-Arg-Pro-Arg-His-Gly-Gln exhibited higher ACE inhibitory activity than captopril, which is a commercial antihypertensive drug. According to Fujita and Yoshikawa[Citation16], peptides generated from food sources have a higher affinity for tissue; therefore, compared to synthetic captopril, its effects are retained in the circular system for extended time.
Table 2. ACE inhibitory activity (IC50) of chemically synthesized peptides identified in thermolysin-injected pork loin
Typically, ACE inhibitory peptides contain 3–20 amino acids[Citation17], and many ACE inhibitory peptides compose 2–12 amino acids.[Citation18] This agrees with our results, as we identified a nona-peptide (Leu-Val-Gly-Arg-Pro-Arg-His-Gly-Gln) and a tetra-peptide (Val-Phe-Pro-Ser) with ACE inhibitory activity. These peptides composing only a few amino acid residues are stable in digestive epithelial barrier and the blood vessels hence, it is prone to reach the peripheral organs and exert their beneficial effects.[Citation19] Previous study observed that small-sized peptides are more resistant to intestinal enzymes and easily absorbed after oral administration.[Citation20] Furthermore, Jang and Lee[Citation12] reported that a peptide of ~3,000 Da from a beef hydrolysate showed high ACE inhibitory activity. Matthews and Payne[Citation21] suggested that short-chain peptides tend to be stable to proteolytic enzymes and can be effectively absorbed since the size of peptides taken up by the absorptive cells in the small intestine is confined to tri- or tetra-peptides. However, Roberts et al.[Citation22] presented evidence that peptides larger than di- or tri-peptides could be imbibed through the small intestine wall of male rats. However, the mechanism for the absorption of intact peptides has not yet been elucidated.
There have been many studies on the structure and function of peptides derived from natural sources with ACE inhibitory activity, and they share some common features. First, ACE selectively reacts with di-peptide sequence of its substrates and inhibitors at the C-terminus, and aliphatic, aromatic, and basic (Lys, Arg) residues are preferred in the penultimate position.[Citation23] Second, ACE prefers substrates and competitive inhibitors that compose hydrophobic amino acids, including Pro, Phe, and Tyr, at the third position from the C-terminus.[Citation24] The tetra-peptide identified in the current study, Val-Phe-Pro-Ser, contains a Phe at the third position from the C-terminus, and it showed an IC50 of 3,606 mM, which is lower than the ACE inhibitory activity of captopril. Third, peptides containing aliphatic (Ala, Val, Leu, or Ile) amino acids at the N-terminus can effectively bind to ACE as an inhibitor.[Citation25] In this study, the tetra- and nona-peptides contained Val and Leu, respectively, at the amino terminus. Phe, an aromatic amino acid, led to the inhibition of ACE. Fourth, the presence of hydrophobic amino acids can contribute to potent ACE inhibition. In addition, previous author[Citation26] suggested that Val-, Leu-, or Ile- N-terminus and Pro-C-terminus in tripeptide possessed strong ACE inhibitory activity. These factors might influence the ACE activity of the tetra- and nona-peptides derived from thermolysin-injected pork loin.
Conclusion
The results of the present study indicated that thermolysin injection of fresh pork loin generated ACE inhibitory peptides (Leu-Val-Gly-Arg-Pro-Arg-His-Gly-Gln and Val-Phe-Pro-Ser). In particular, the nona-peptide Leu-Val-Gly-Arg-Pro-Arg-His-Gly-Gln showed ACE inhibitory activity levels similar to that of captopril, which is a commercial antihypertensive drug. In other words, the application of thermolysin-injected pork loin could provide health benefits. However, the efficacy of these ACE inhibitory peptides may be reduced by gastrointestinal proteases. For this reason, in future studies, the stability of the ACE inhibitory peptides isolated from thermolysin-injected pork in the digestive system should be confirmed.
Disclosure statement
There are no conflicts of interest to declare.
Additional information
Funding
References
- Choe, J.; Seol, K. H.; Kim, H. J.; Hwang, J. T.; Lee, M.; Jo, C. Isolation and Identification of Angiotensin I-Converting Enzyme (ACE) Inhibitory Peptides Derived from Thermolysin-Injected Hanwoo M. Longissimus. Asian-Australas. J. Anim. Sci. 2019, 32, 430–436. DOI: 10.5713/ajas.18.04.
- Maruyama, S.;. Angiotensin Converting Enzyme Inhibitors. In Methods of Study the Nutraceuticals Compounds in Foods; Kawagishi, S., Ed.; Kakkaishutban Center: Tokyo, Japan, 1997; pp 116–129.
- Takei, Y.; Ando, H.; Tsutsui, K. Handbook of Hormones: Comparative Endocrinology for Basic and Clinical Research. In Renin-Angiotensin System; Wong, M. K. S., Ed.; Elsevier: Oxford, 2016; pp iv.
- Maruyama, S.; Nakagomi, K.; Tomizuka, N.; Suzuki, H. Angiotensin-I Converting Enzyme Inhibitor Derived from an Enzymatic Hydrolysate of Casein. II. Isolation and Dradykinin-Potentiating Activity in the Uterus and Ileum of Rats. Agric. Biol. Chem. 1985, 49, 1405–1409. DOI: 10.1080/00021369.1985.10866901.
- Saito, Y.; Nakamura, K.; Kawato, A.; Imayasu, S. Angiotensin-I Converting Enzyme Inhibitors in Sake and Its Byproducts. Nippon Nogeik. Kaishi 1992, 66, 1081–1087. DOI: 10.1271/nogeikagaku1924.66.1081.
- Bhattacharya, R.; Jana, K.; Sahana, D. An Overview of Management of Hypertension with Angiotensin-Converting Enzyme Inhibitors. J. Drug Discov. Ther. 2017, 5, 01–10.
- Saiga, A.; Okumura, T.; Makihara, T.; Katsuta, S.; Shimizu, T.; Yamada, R.; Nishimura, T. Angiotensin I-Converting Enzyme Inhibitory Peptides in a Chicken Breast Muscle Extract. J. Agric. Food Chem. 2003, 51, 1741–1745. DOI: 10.1021/jf020604h.
- Salampessy, J.; Reddy, N.; Phillips, M.; Kailasapathy, K. Isolation and Characterization of Nutraceutically Potential ACE-Inhibitory Peptides from Leatherjacket (Meuchenia Sp.) Protein Hydrolysates. LWT-Food Sci. Technol. 2017, 80, 430–436. DOI: 10.1016/j.lwt.2017.03.004.
- Seol, K. H.; Choe, J.; Kim, H. J.; Hwang, J. T.; Lee, M.; Jo, C. Bioactivities of Peptide Fractions Derived from Proteolytic Enzyme-Injected Hanwoo Longissimus Muscle in a Model System. Int. J. Food Proper. 2018, 21, 220–227. DOI: 10.1080/10942912.2018.1440241.
- Wang, X.; Chen, H.; Fu, X.; Li, S.; Wei, J. A Novel Antioxidant and ACE Inhibitory Peptide from Rice Bran Protein: Biochemical Characterization and Molecular Docking Study, LWT-Food Sci. Technol. 2017, 75, 93–99. DOI: 10.1016/j.lwt.2016.08.047.
- Yoon, J. W.; Lee, D. G.; Lee, H. J.; Choe, J.; Jung, S.; Jo, C. Microbial, Physicochemical, and Sensory Characteristics of Quality Grade 2 Beef Enhanced by Injection of Pineapple Concentrate and Honey. Korean J. Food Sci. Anim. Resour. 2017, 37, 494–501. DOI: 10.5851/kosfa.2017.37.4.494.
- Jang, A.; Jo, C.; Kang, K. S.; Lee, M. Antimicrobial and Human Cancer Cell Cytotoxic Effect of Synthetic Angiotensin-Converting Enzyme (ACE) Inhibitory Peptides. Food Chem. 2008, 107, 327–336. DOI: 10.1016/j.foodchem.2007.08.036.
- Seol, K. H.; Song, J. H.; Prayad, T.; Kim, H. W.; Jang, A.; Ham, J. S.; Oh, M. H.; Kim, D. H.; Lee, M. Assessment of the Inhibitory Activity of Peptide Extracts from Hanwoo Musculus Longissimus on Angiotensin I-Converting Enzyme. Korean J. Food Sci. An. 2011, 31, 663–667. DOI: 10.5851/kosfa.2011.31.5.663.
- Dhanabalan, V.; Xavier, M.; Kannuchamy, N.; Asha, K. K.; Sngh, C. B.; Balange, A. Effect of Processing Conditions on Degree of Hydrolysis, ACE Inhibition, and Antioxidant Activities of Protein Hydrolysate from Acetes Indicus. Environ. Sci. Pollut. Res. 2017, 24, 21222–21232. DOI: 10.1007/s11356-017-9671-4.
- Megías, C.; Yust, M. D. M.; Pedroche, J.; Lquari, H.; Girón-Calle, J.; Alaiz, M.; Millán, F.; Vioque, J. Purification of an ACE Inhibitory Peptide after Hydrolysis of Sunflower (Helianthus Annuus L.) Protein Isolates. J. Agric. Food Chem. 2004, 52, 1928–1932. DOI: 10.1021/jf034707r.
- Fujita, H.; Yoshikawa, M. LKPNM: A Prodrug-Type ACE Inhibitory Peptide Derived from Fish Protein. Immunopharmacol. 1999, 44, 123–127. DOI: 10.1016/S0162-3109(99)00118-6.
- Pihlanto, L.;. Bioactive Peptides Derived from Bovine Whey Proteins: Opioid and Ace-Inhibitory. Trends Food Sci. Tech. 2000, 11, 347–356. DOI: 10.1016/S0924-2244(01)00003-6.
- Byun, H. G.; Kim, S. K. Structure and Activity of Angiotensin I Converting Enzyme Inhibitory Peptides Derived from Alaskan Pollack Skin. J. Biochem. Mol. Biol. 2002, 35, 239–243. DOI: 10.5483/BMBRep.2002.35.2.239.
- Yust, M. M.; Pedroche, J.; Giron-Calle, J.; Alaiz, M.; Millan, F.; Vioque, J. Production of ACE Inhibitory Peptides by Digestion of Chickpea Legumin with Alcalase. Food Chem. 2003, 81, 363–369. DOI: 10.1016/S0308-8146(02)00431-4.
- Segura-Campos, M.; Chel-Guerrero, L.; Betancur-Ancona, D.; Hernandez-Escalante, V. M. Bioavailability of Bioactive Peptides. Food Rev. Int. 2011, 27, 213–226. DOI: 10.1080/87559129.2011.563395.
- Matthews, D. M.; Payne, W. Transmembrane Transport of Small Peptides. Curr. Top. Membr. Trans. 1980, 14, 331–425. DOI: 10.1016/S0070-2161(08)60119-7.
- Roberts, P. R.; Burney, J. D.; Black, K. W.; Zaloga, G. P. Effect of Chain Length on Absorption of Biologically Active Peptides from the Gastrointestinal Tract. Digestion. 1999, 60, 332–337. DOI: 10.1159/000007679.
- Kohmura, M.; Nio, N.; Kubo, K.; Minoshima, Y.; Munekata, E.; Ariyoshi, Y. Inhibition of Angiotensin-Converting Enzyme by Synthetic Peptides of Human α-casein. Agric. Biol. Chem. 1989, 53, 2107–2114. DOI: 10.1080/00021369.1989.10869621.
- Cheung, H. S.; Wang, F. L.; Ondetti, M. A.; Sabo, E. F.; Cushman, D. W. Binding of Peptide Substrates and Inhibitors of Angiotensin-Converting Enzyme. Importance of the COOH-terminal Dipeptide Sequence. J. Biol. Chem. 1980, 255, 401–407.
- Pan, S.; Wang, S.; Jing, L.; Yao, D. Purification and Characterization of a Novel angiotensin-I Converting Enzyme (Ace)-Inhibitory Peptide Derived from Enzymatic Hydrolysate of Enteromorpha Clathrate Protein. Food Chem. 2016, 211, 423–430. DOI: 10.1016/j.foodchem.2016.05.087.
- Matsumura, N.; Fugii, M.; Takeda, Y.; Sugita, K.; Shimizu, T. Angiotensin I Converting Enzyme Inhibitory Peptides Derived from Dried Bonito Bowels Autolysate. Biosci. Biotechnol. Biochem. 1993, 57, 695–697. DOI: 10.1271/bbb.57.695.