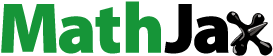
ABSTRACT
The ability to readily determine the composition of brown rice vinegar is important for quality assurance purposes. To that end, solid–phase microextraction (SPME)–Arrow method was optimized for the determination of volatile organic compounds (VOCs) from brown rice vinegar. Compared to traditional SPME fibers, SPME–Arrow has a larger sorbent-phase volume and is more durable. The SPME–Arrow conditions, including coating material, extraction temperature, extraction time, and NaCl concentration, were examined, which led to optimum extraction conditions that include: carboxen/polydimethylsiloxane (CAR/PDMS) fiber, 50°C, 30 min of SPME–Arrow, and 0% NaCl (i.e., no added NaCl). The developed method was compared to an optimized method based on conventional SPME. Twelve major VOCs, including acetates, alcohols, aldehydes, acids, and furan derivatives, were extracted by SPME–Arrow, while only seven VOCs were extracted using the traditional SPME. Moreover, SPME–Arrow extracted more 1.3–2 times more VOCs than SPME. Hence, the CAR/PDMS SPME–Arrow fiber is suitable for acquiring VOC profiles from brown rice vinegar.
Introduction
Vinegar, which is an acetic acid solution, is produced in complex fermentation procedures from a variety of raw materials such as fruits and grains.[Citation1,Citation2] In Korea, brown rice vinegar is typically obtained from bran-containing unpolished brown rice mixed with a Korean fermentation starter (nuruk), and is used as a flavor enhancer and preserving agent. Brown rice vinegar has attracted much attention due to its possible anti-aging role,[Citation3] and its antioxidant,[Citation4] antihypertensive,[Citation5] and immune-enhancing properties.[Citation6] These beneficial effects may be due to bioactive substances derived from the raw materials used to produce the vinegar.
In addition to bioactive substances, the volatile organic compounds (VOCs) in vinegar are crucial components that impact on product quality and consumer acceptance. The VOCs and sensory quality of vinegar are directly influenced by the raw materials, fermentation procedure, and aging.[Citation1,Citation2,Citation7] With this in mind, it is reasonable to suppose that VOC characterization using analytical techniques can play a crucial role in determining the quality of vinegar.
As a methodology for evaluating VOCs, the solid-phase microextraction (SPME) technique developed by Pawliszyn[Citation8] has been widely used to extract VOCs from liquid foods.[Citation7,Citation9] Sample preparation for SPME is simple, which minimizes the introduction of errors during analysis, resulting in more accurate and reliable analytical results.[Citation10] Analytes are absorbed on sorbent-coated small fibers in SPME. Recently, a novel technology that uses the SPME–Arrow device was introduced for the microextractions of larger volumes of sorbent material compared to those of traditional SPME fiber.[Citation11] The SPME–Arrow device has been designed for improved mechanical robustness, and consists of an inner metal rod coated with a sorbent material protected by an outer metal tube, which together lead to an arrow-shaped tip. The larger volume (6–20 times that of conventional SPME) of the sorption phase used with SPME–Arrow provides improved sensitivities and extraction efficacies based on the merits of conventional SPME.[Citation11]
A considerable number of headspace-SPME-based (HS-SPME-based) studies that analyze VOCs in vinegar derived from a variety of fruits and grains have been reported in recent years.[Citation7,Citation12,Citation13] However, the key VOCs in brown rice vinegars have been reported to a lesser extent. Moreover, the extraction efficacy of HS-SPME–Arrow for VOCs in brown rice vinegar has not been studied as this novel technique has only appeared recently. Herein, we propose that HS-SPME–Arrow combined with GC-MS/MS can be used to more-efficiently determine VOCs in brown rice vinegar than conventional techniques. In this study, we established such a sensitive HS-SPME–Arrow method for the detection of major VOCs, including acids, acetates, alcohols, aldehydes, and furan derivatives, in brown rice vinegar. We optimized the extraction conditions, including the type of fiber, NaCl concentration, extraction time, and extraction temperature. In addition, the extraction efficiencies and VOC profiles of the HS-SPME–Arrow-based method were directly compared with those of the traditional HS-SPME method.
Materials and methods
Materials
SPME–Arrow fibers, including polydimethylsiloxane (PDMS) (100 μm 20 mm), divinylbenzene (DVB)/PDMS (120 μm
20 mm), and carboxen/PDMS (CAR/PDMS) (120 μm
20 mm) were acquired from CTC Analytics AG (Zwingen, Switzerland). Traditional SPME fibers, including PDMS/DVB (65 μm
10 mm, 0.6 μL), PDMS (100 μm
10 mm, 0.6 μL), DVB/CAR/PDMS (50/30 μm
10 mm, 0.6 μL), and CAR/PDMS (75 μm
10 mm, 0.6 μL) were purchased from Supelco (Bellefonte, PA, USA). The 4-methyl-2-pentanol internal standard was purchased from Sigma-Aldrich (St. Louis, MO, USA). All reagents were of analytical grade.
Gc-ms/ms
VOCs in brown rice vinegar were analyzed using a GC-MS instrument composed of a Triplus RSH Autosampler coupled with a TRACE 1310 GC system, and an ISQ LT single-quadrupole mass-detector (Thermo Scientific, West Palm Beach, FL, USA). The injector temperature was 250°C and split mode (5:1) was used. Chromatographic separation was achieved on a DB-WAX column (60 m × 0.25 mm i.d. × 0.25 μm) with helium as the carrier gas at a constant flow rate of 1.0 mL min−1. The initial oven temperature of 50°C was held for 2 min, and then raised to 210°C at a rate of 2.5°C min−1 and held there for 2 min. The temperatures of the MS transfer line, source, and quadrupole were 280, 230, and 150°C, respectively. A mass scan range of 50–450 amu was used, and the mass spectrometer was operated at 70 eV in the 40–400 amu range. All data were analyzed with the Xcalibur software. The volatile compounds were identified by comparing their MS fragments with a library of mass spectra (Wiley/NIST 2008) using the Kovats retention index based on the retention times of standard n-alkanes. Quantitative analyses are expressed as relative ratios of the areas of individual VOCs extracted with different fibers against the highest volatile-compound area among the fibers used.
Sample preparation
Commercial brown rice vinegars were obtained from a local supermarket (Seongnam, Korea). A sample (1 mL) was transferred into a 20-mL headspace vial equipped with a PTFE/silicone septum screw-cap. 4-Methyl-2-pentanol was added as the internal standard prior to extraction. The vials were placed in the autosampler tray for GC-MS analysis. The VOCs were extracted from the brown rice vinegar using conventional HS–SPME or HS–SPME–Arrow.
SPME–arrow and conventional SPME conditions
The VOC extraction efficacies of three sorbents (100 μm PDMS, 120 μm CAR/PDMS, and 120 μm DVB/PDMS) were compared for HS-SPME–Arrow. Based on the normalized peak area of each VOC, we compared extraction time (10, 30, and 60 min), temperature (40, 50, and 60°C), and the NaCl concentration (0, 4, and 8%, w/v) in order to optimize the extraction conditions.
Four conventional SPME fibers, including PDMS/DVB (65 μm), PDMS (100 μm), DVB/CAR/PDMS (50/30 μm), and CAR/PDMS (75 μm), were examined for the conventional SPME method. In addition to providing the best fiber coating, extraction time and temperature were also optimized. Finally, the extraction efficiencies and VOC profiles of the selected HS-SPME–Arrow and traditional HS-SPME fibers were compared under optimum extraction conditions.
Sensitivities and reproducibility of SPME–arrow and conventional SPME
The sensitivities and reproducibilities of selected SPME–Arrow and conventional SPME fibers were comprehensively evaluated. The sensitivities toward selected classes of VOC were evaluated based on the “cumulative area normalization value” parameter, as follows:
where K is the number of each category of VOC; X represents a selected fiber, AVK is the average peak area of compound K determined by two fibers, AK(X) is the absolute area of compound K determined by fiber X, NAK(X) is the normalized area of compound K determined by fiber X, and CA K(X) is the cumulative area of a particular category of VOC (from 1 to K) determined by fiber X. Reproducibilities were determined by calculating the relative standard deviations (RSDs) of selected fiber-adsorbed VOCs; the average RSD provides an indicator of repeatability, while the standard deviation of the RSD provides an indicator of variation.
Statistical analysis
All data are expressed as means with standard deviations from three replicate experiments. Data were analyzed using the students t-test or one-way analysis of variance followed by Duncan’s multiple range tests at the p < .05 level. The IBM SPSS statistics software (version 20, SPSS Inc., Chicago, IL, USA), was used for these statistical analyses.
Results and discussion
Optimizing the extraction conditions using spme–arrow
The main factors affecting VOC extraction efficacy (i.e., fiber coating, extraction temperature, exposure time, and NaCl concentration) were optimized. In recent years, the headspace analysis such as SPME, SPME-Arrow, dynamic headspace (DHS) and stir bar sorptive extraction (SBSE) is widely used for volatile extraction. These headspace techniques are optimized in terms of several extraction parameters including fiber type, extraction temperature, extraction time, desorption time, salt content, pH, and the possible interactions between independent variables, which can significantly affect the extraction efficacy.[Citation9–Citation16] All experiments were performed in triplicate and the experimental conditions were optimized on the basis of normalized peak areas.
Selecting the appropriate spme–arrow fiber
Headspace VOCs were extracted using either the 100-μm PDMS, 120-μm CAR/PDMS, or 120-μm DVB/PDMS SPME–Arrow fiber. The main VOCs in vinegar can be classified into several groups, including aldehydes, acids, alcohols, acetates, and furan derivatives.[Citation6,Citation12] Accordingly, we selected seven major VOCs and determined their extraction yields using the above-mentioned SPME–Arrow fibers; these seven VOCs were tentatively identified and the normalized peak areas obtained using each tested fiber are shown in . The identified compounds belong to the following chemical groups: acetate (ethyl acetate), alcohol (ethanol, 1-hexanol), aldehyde (2-hexenal), acid (acetic acid), and furan derivatives (furfural and 2-furanmethanol). The results in indicate that the 120-μm CAR/PDMS SPME–Arrow fiber significantly (p < .05) extracted several VOCs, including ethyl acetate, 2-hexenal,1-hexanol, and the furan derivatives (furfural and 2-furanmethanol) from brown rice vinegar, compared to the 100-μm PDMS, and 120-μm DVB/PDMS SPME–Arrow fibers. The efficacies of the 120-μm CAR/PDMS, 100-μm PDMS, and 120-μm DVB/PDMS SPME–Arrow fibers toward ethanol and acetic acid extraction were not significantly (p > .05) different.
Figure 1. Relative peak areas as functions of extraction conditions: (a) SPME–Arrow fiber, (b) extraction time, (c) extraction temperature, and (d) concentration of added NaCl
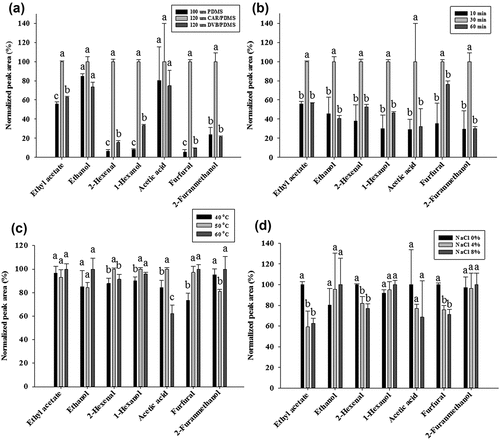
The selection of the appropriate coating material is a crucial step in the optimization and development of the SPME method. The suitability of a particular fiber coating for a specific analyte is related to the polarity of the extraction phase and the overall selectivity toward the analyte over other matrix components.[Citation17] A considerable number of VOC-analysis studies using CAR/PDMS fibers have been reported with the aim of assessing vinegar quality. Mejıas et al.[Citation12] reported that the CAR/PDMS fiber exhibited better performance for determining acetates, alcohols, and aldehydes in Chinese vinegars compared to DVB/PDMS or PDMS fibers. Moreover, Zhang et al.[Citation7] reported that furfural, acetic acid, ethyl acetate, 3-methyl-1-butanol, and phenylethyl alcohol are major VOCs of rice vinegar, as determined using silica fiber coated with CAR/PDMS for VOC extraction. A CAR/PDMS fiber, which was produced with a cross-linked coating, is bipolar and can be used for matrices containing polar and non-polar VOCs. On the other hand, a PDMS fiber (non-polar) is poorly sensitive to highly volatile and polar compounds. A CAR/PDMS fiber was found to exhibit high affinity for the extraction of aldehydes, alcohols, and acids[Citation12,Citation18]; this fiber was suitable for extracting VOCs with low molecular weights, in the 30–225 amu range, due to its porosity and characteristic pores.[Citation19] These results are consistent with our results ()), in which the CAR/PDMS coating was shown to exhibit the best extraction efficacy for compounds of low molecular weight. Moreover, CAR-coated fibers, such as CAR/PDMS, facilitate the adsorption of a wider range of molecular weights due to their similar volumes of micro-, meso- and macropores, compared to DVB-coated fibers, such as DVB/PDMS that contain relatively few micropores.[Citation20] In addition, a CAR/PDMS fiber was effective for extracting low-boiling compounds.[Citation20] The higher peak areas of the VOCs extracted with the bipolar porous CAR/PDMS coating, compared to those obtained using the PDMS and DVB/PDMS coatings is shown in ). Accordingly, the CAR/PDMS SPME–Arrow fiber was chosen for further use, and the HS-SPME–Arrow conditions for the extraction of VOCs from brown rice vinegar were optimized.
Extraction time
The performance of the CAR/PDMS SPME–Arrow fiber for the extraction of VOCs from brown rice vinegar was further investigated for extraction times of 10, 30, and 60 min ()). The normalized peak areas of selected VOCs were observed to significantly (p < .05) increase as the extraction time was increased from 10 to 30 min; however the extracted amounts of all VOCs were significantly (p < .05) lower at an extraction time of 60 min. The optimum time required for the SPME fiber to reach equilibrium needs to be determined in order to produce reproducible quantitative-analysis data. Therefore, based on the results presented in ), 30 min was selected as optimum for the extraction of VOCs from brown rice vinegar in subsequent experiments.
Extraction temperature
The effect of extraction temperature (40, 50, and 60°C) on VOC extraction from brown rice vinegar is shown in ). Ethyl acetate and ethanol, with low molecular weights and low boiling points, equilibrated at 40°C, whereas the optimum temperature for the higher-boiling 2-furanmethanol, was found to be 60°C. The peak areas of 2-hexenal and acetic acid were significantly (p < .05) higher at 50°C compared to those of 40°C, but were lower at 60°C. Except for 2-furanmethanol, the best extraction results for the VOCs were obtained at 50°C ()). Thermodynamically, higher temperatures result in higher amounts of VOC due to their enhanced partial vapor pressures and their Henry’s law constants. However, the ability of a fiber coating to adsorb an analyte decreases with increasing temperature. Furthermore, the partition coefficient is lower at high temperature, which results in lower extraction efficacy.[Citation21] Feng et al.[Citation9] also reported that VOCs with low molecular weights, including ethanol and ethyl acetate, exhibited lower peak areas at higher temperatures due to lower partition coefficients. On the basis of these experiments, we consider 50°C to be adequate for obtaining the best extraction efficiencies and this temperature was used in subsequent studies.
Salting effect
In general, the addition of salting-out agent increases VOC extraction efficacies, which is due to the higher ionic strength of the sample solution. The addition of NaCl usually decreases VOC solubility and facilitates the adsorption of higher amounts of analyte onto the fiber coating. Contrary to expectation, the addition of NaCl to the solutions in this study resulted in lower extraction efficiencies or no significant change compared to samples devoid of added NaCl, which indicates that higher ionic strength leads to lower extraction efficacies ()).[Citation22] These results are consistent with those of a previous study, in which NaCl was used in an attempt to improve the salting-out effect.[Citation23] To ensure optimal extraction performance, salt was not added in further studies. On the basis of the above-mentioned experimental results, the optimum extraction time, extraction temperature, NaCl concentration, and fiber type for the HS-SPME–Arrow analysis of brown rice vinegar were determined to be 30 min, 50°C, 0%, and the CAR/PDMS SPME–Arrow fiber, respectively.
Comparing the SPME–arrow and conventional SPME methods
Optimizing the conventional SPME method
The performance of four conventional SPME fibers, namely PDMS/DVB (65 μm), PDMS (100 μm), DVB/CAR/PDMS (50/30 μm), and CAR/PDMS (75 μm), were examined by extracting VOCs from brown rice vinegar (Supplementary Figure 1(A)). The CAR/PDMS fiber was determined to be the most suitable conventional SPME fiber, and was selected for conventional SPME. The optimum extraction time and temperature were determined in a preliminary study to be 30 min (Supplementary Figure 1(B)), and 50°C (Supplementary Figure 1(C)), respectively.
Comparing VOCsVOCs were analyzed by GC-MS following extraction with the CAR/PDMS SPME–Arrow and conventional CAR/PDMS SPME fibers (). A total of 12 VOCs, including acids, acetates, alcohols, aldehydes, and furan derivatives, were identified using SPME–Arrow, while seven VOCs were determined using the traditional SPME. A comparison of the extraction efficacies of the SPME–Arrow and conventional SPME fibers reveals that SPME–Arrow provides a more complete profile due to the wider range of VOCs detected; in particular, hexyl acetate, 1-hexanol, (Z)-3-hexen-1-ol, (E)-2-hexen-1-ol, and 2-furanmethanol were only detected by SPME–Arrow. The relative concentrations obtained by CAR/PDMS SPME–Arrow for ethyl acetate, 2-hexenal, acetic acid, furfural, and 2-acetylfuran were significantly (p < .05) higher than those obtained using the traditional CAR/PDMS fiber; however, they were nearly equal for ethanol and hexanal. These results demonstrate the advantages of SPME–Arrow over the conventional SPME fiber.
Table 1. Volatile components of brown rice vinegar identified using SPME–Arrow and conventional SPME fibers
Evaluating VOCs in vinegar is useful for ascertaining product quality, which is influenced by aging time, storage conditions, fermentation procedures, and the raw materials used in its production. Chung et al.[Citation13] reported that ethyl acetate, acetic acid, ethanol, and furfural were major VOCs in brown rice vinegar by conventional SPME, which is in agreement with our results using both SPME–Arrow and conventional SPME fibers (). Ethanol and acetic acid in vinegar are representative VOCs for the alcoholization or vinegarization stages, respectively; hence, these VOCs can be used as biomarkers for improving vinegar quality.[Citation24] Furfural, a furan derivative formed through Maillard reactions, is responsible for the caramel-like flavor of vinegar. 2-Acetylfuran, a furfural-related compound, is a characteristic VOC that distinguishes aged vinegars from less-matured products.[Citation25] Aldehydes are generated by the oxidation of alcohols or the decarboxylation of acids during fermentation. Previous study reported that the proportions of aldehydes, including 2-hexenal and hexanal, depended significantly on aging time and the raw materials used to produce the vinegar.[Citation26] Some VOCs in brown rice vinegar, including (Z)-3-hexen-1-ol, (E)-2-hexen-1-ol, 2-furanmethanol, hexyl acetate, and 1-hexanol, were detected only by SPME–Arrow (). In previous studies, (Z)-3-hexen-1-ol, also known as a green leaf volatile, was considered to be exclusive to wine, tarragon, and apple vinegars.[Citation27] Furthermore, (E)-2-hexen-1-ol has only been previously found in specific vinegars.[Citation28] Ishihara et al.[Citation29] reported that these VOCs are derived from raw materials since no significant changes in their concentrations were observed during the fermentation stage. 2-Furanmethanol, also known as furfuryl alcohol, is a flavoring ingredient. Hexyl acetate (apple flavor) is produced by the esterification of acids with alcohols,[Citation30] and Lee et al.[Citation31] reported that the alcohol contents depended on acidity, with the majority being ethanol and 1-hexanol. Therefore, these SPME–Arrow-extracted VOCs contribute to the assessment and characterization of brown rice vinegar quality.
Evaluating sensitivity and reproducibility
Cumulative VOC areas were used to evaluate the sensitivities of the CAR/PDMS SPME–Arrow and traditional CAR/PDMS SPME fibers (); the higher the cumulative area, the higher the fiber sensitivity toward the same number of accumulated analytes. As shown in , the CAR/PDMS SPME–Arrow fiber exhibited a higher sensitivity to each category of VOC. Among the various categories of VOC, the CAR/PDMS SPME–Arrow fiber exhibited the highest increase in normalized cumulative area for alcohols, which is apparently related to the number of accumulated compounds.
Figure 2. Cumulative areas as functions of the number of accumulated analytes: depicting the sensitivities of CAR/PDMS SPME-Arrow and conventional CAR/PDMS fibers to different categories of VOC
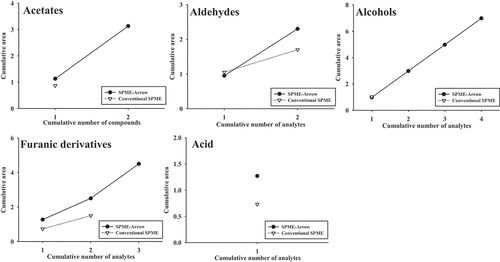
The reproducibility of the CAR/PDMS SPME–Arrow and traditional CAR/PDMS SPME fibers were compared on the basis of average RSD and the standard deviation of the RSD obtained from the VOC peak areas listed in . The results, shown in , indicate that the CAR/PDMS SPME–Arrow fiber provides more reproducible results, with a lower average RSD and standard deviation of the RSD than the traditional CAR/PDMS SPME fiber.
Figure 3. Reproducibilities of the CAR/PDMS SPME–Arrow and conventional CAR/PDMS SPME fibers: standard deviation of the average relative standard deviation (RSD) as a function of the average RSD for brown rice vinegar VOCs
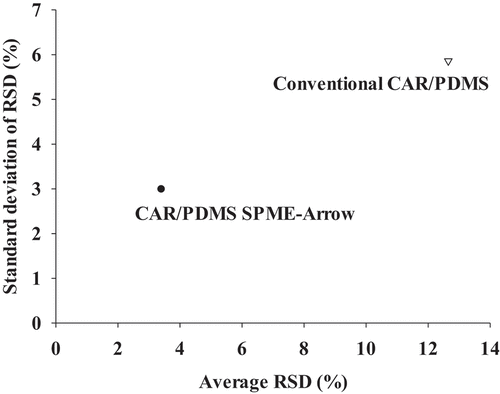
The SPME–Arrow SPME-based device provides a greater sorption-phase volume while maintaining the advantages of conventional SPME. Hence, SPME–Arrow exhibits an enhanced extraction efficacy and higher sensitivity over the detection range compared to conventional SPME. The use of SPME–Arrow resulted in an improved extraction capacity of aroma compounds in wine due to the increase of sorbent phase volume.[Citation32] In addition to food matrices, HS-SPME–Arrow extraction has been applied to determine volatile compounds in Korean salt-fermented fish sauce, providing better sensitivity and robustness.[Citation11] Barreira et al.[Citation33] reported that SPME-Arrow improved the extraction efficacy of VOCs in the atmosphere with an up to 2-fold increase. The SBSE, one of microextraction technique, can also provide high sensitivity of analysis.[Citation15] However, direct contact with the matrix may cause physical damage and contamination of stir bar. SMPE–Arrow has been shown to overcome the disadvantages of SBSE, which is possibly due to headspace extraction.[Citation34] Our results ( and ) reveal that CAR/PDMS SMPE–Arrow performs well for VOC extraction; hence it was used comprehensively to analyze VOCs in brown rice vinegar. The performance of CAR/PDMS SMPE–Arrow may be due to the intrinsic SMPE–Arrow design that is capable of extracting a wide range of VOC with high sensitivity.
Conclusion
In this study, we developed a sensitive and reproducible method for determining VOCs in brown rice vinegar based on HS-SPME–Arrow coupled with GC-MS/MS. Like conventional HS–SPME, HS-SPME–Arrow is a very fast, simple, and solventless technique. The optimized vinegar-VOC extraction conditions were determined to involve a CAR/PDMS SPME–Arrow fiber absorbing for 30 min at 50°C without the addition of NaCl. However, further study is needed to examine the possible interactions between independent variables. Among the examined fiber coatings, the CAR/PDMS SPME–Arrow fiber extracted acid, acetate, alcohol, aldehyde, and furan-based VOCs most efficiently. In addition, we compared the extraction efficacies of SPME–Arrow and conventional SPME on the basis of extraction yield. The CAR/PDMS SPME–Arrow fiber was found to extract VOCs more sensitively and reproducibly than the conventional CAR/PDMS SPME fiber. We conclude that CAR/PDMS SPME–Arrow is a promising and powerful extraction technique for the analysis of VOCs in brown rice vinegar.
Supplemental Material
Download MS Word (296 KB)Supplementary material
Supplemental data for this article can be accessed here.
Additional information
Funding
References
- Koyama, M.; Ogasawara, Y.; Endou, K.; Akano, H.; Nakajima, T.; Aoyama, T.; Nakamura, K. Fermentation-Induced Changes in the Concentrations of Organic Acids, Amino Acids, Sugars, and Minerals and Superoxide Dismutase-Like Activity in Tomato Vinegar. Int. J. Food Prop. 2017, 20(4), 888–898. DOI: 10.1080/10942912.2016.1188309.
- Zhang, Q.; Huo, N.; Wang, Y.; Zhang, Y.; Wang, R.; Hou, H. Aroma-Enhancing Role of Pichia Manshurica Isolated from Daqu in the Brewing of Shanxi Aged Vinegar. Int. J. Food Prop. 2017, 20(9), 2169–2179. DOI: 10.1080/10942912.2017.1297823.
- Chen, J.; Tian, J.; Ge, H.; Liu, R.; Xiao, J. Effects of Tetramethylpyrazine from Chinese Black Vinegar on Antioxidant and Hypolipidemia Activities in HepG2 Cells. Food Chem. Toxicol. 2017, 109(2), 930–940.
- Chou, C. H.; Liu, C. W.; Yang, D. J.; Wu, Y. H. S.; Chen, Y. C. Amino Acid, Mineral, and Polyphenolic Profiles of Black Vinegar, and Its Lipid Lowering and Antioxidant Effect in Vivo. Food Chem. 2015, 168(1), 63–69. DOI: 10.1016/j.foodchem.2014.07.035.
- Park, K. M.; Lee, S. H. Anti-Hyperlipidemic Activity of Rhynchosia Nulubilis Seeds Pickled with Brown Rice Vinegar in Mice Fed a High-Fat Diet. Nutr. Res. Pract. 2013, 7(6), 453–459. DOI: 10.4162/nrp.2013.7.6.453.
- Kim, H.; Lee, H.; Shin, K. S. Intestinal Immunostimulatory Activity of Neutral Polysaccharide Isolated from Traditionally Fermented Korean Brown Rice Vinegar. Biosci. Biotechnol. Biochem. 2016, 80(12), 2383–2390. DOI: 10.1080/09168451.2016.1217149.
- Zhang, X.; Wang, P.; Xu, D.; Wang, W.; Zhao, Y. Aroma Patterns of Beijing Rice Vinegar and Their Potential Biomarker for Traditional Chinese Cereal Vinegars. Food Res. Int. 2019, 119, 398–410.
- Arthur, C. L.; Pawliszyn, J. Solid Phase Microextraction with Thermal Desorption Using Fused Silica Optical Fibers. Anal. Chem. 1990, 62(19), 2145–2148. DOI: 10.1021/ac00218a019.
- Feng, Y.; Su, G.; Sun-Waterhouse, D.; Cai, Y.; Zhao, H.; Cui, C.; Zhao, M. Optimization of Headspace Solid-Phase Micro-Extraction (HS-SPME) for Analyzing Soy Sauce Aroma Compounds via Coupling with Direct GC-Olfactometry (D-GC-O) and Gas Chromatography-Mass Spectrometry (GC-MS). Food Anal. Methods. 2017, 10(3), 713–726. DOI: 10.1007/s12161-016-0612-5.
- Iranmanesh, M.; Ezzatpanah, H.; Akbari-Adergani, B.; Torshizi, M. A. K. SPME/GC-MS Characterization of Volatile Compounds of Iranian Traditional Dried Kashk. Int. J. Food Prop. 2018, 21(10), 1067–1079. DOI: 10.1080/10942912.2018.1466323.
- Song, N. E.; Lee, J. Y.; Lee, Y. Y.; Park, J. D.; Jang, H. W. Comparison of headspace-SPME and SPME-Arrow –GC-MS Methods for the Determination of Volatile Compounds in Korean Salt-Fermented Fish Sauce. Appl. Biol. Chem. 2019, 62(16), 1–8. DOI: 10.1186/s13765-019-0424-6.
- Mejías, R. C.; Marín, R. N.; Moreno, M. D. V. G.; Barroso, C. G. Optimisation of Headspace Solid-Phase Microextraction for Analysis of Aromatic Compounds in Vinegar. J. Chromatogr. A. 2002, 953(1–2), 7–15. DOI: 10.1016/S0021-9673(02)00122-X.
- Chung, N.; Jo, Y.; Joe, M. H.; Jeong, M. H.; Jeong, Y. J.; Kwon, J. H. Rice Vinegars of Different Origins: Discriminative Characteristics Based on Solid-Phase Microextraction and Gas Chromatography with Mass Spectrometry, an Electronic Nose, Electronic Tongue and Sensory Evaluation. J. Inst. Brew. 2017, 123(1), 159–166. DOI: 10.1002/jib.406.
- Ma, L.; Qiao, Y.; Du, L.; Li, Y.; Huang, S.; Liu, F.; Xiao, D. Evaluation and Optimization of a Superior Extraction Method for the Characterization of the Volatile Profile of Black Tea by HS-SPME/GC-MS. Food Anal. Methods. 2017, 10(7), 2481–2489. DOI: 10.1007/s12161-016-0785-y.
- Lee, J.; Shibamoto, T.; Ha, J.; Jang, H. W. Identification of Volatile Markers for the Detection of Adulterants in Red Ginseng (Panax Ginseng) Juice Using Headspace Stir-Bar Sorptive Extraction Coupled with Gas Chromatorgraphy and Mass Spectrometry. J. Sep. Sci. 2018, 41(14), 2903–2912. DOI: 10.1002/jssc.201800202.
- Manzini, S.; Durante, C.; Baschieri, C.; Cocchi, M.; Sighinolfi, S.; Totaro, S.; Marchetti, A. Optimization of a Dynamic Headspace-Thermal Desorption-Gas Chromatography/Mass Spectrometry Procedure for the Determination of Furfurals in Vinegars. Talanta. 2011, 85(2), 863–869. DOI: 10.1016/j.talanta.2011.04.018.
- Fan, Y.; Li, Z.; Xue, Y.; Hou, H.; Xue, C. Identification of Volatile Compounds in Antarctic Krill (Euphausia Superba) Using Headspace Solid-Phase Microextraction and GC-MS. Int. J. Food Prop. 2017, 20(sup1), S820–S829. DOI: 10.1080/10942912.2017.1315589.
- Ji, J.; Liu, H.; Chen, J.; Zeng, J.; Huang, J.; Gao, L.; Wang, Y.; Chen, X. ZnO Nanorod Coating for Solid Phase Microextraction and Its Applications for the Analysis of Aldehydes in Instant Noodle Samples. J. Chromatogr. A. 2012, 1246, 22–27. DOI: 10.1016/j.chroma.2012.01.080.
- Shirey, R. E.;. Optimization of Extraction Conditions and Fiber Selection for Semivolatile Analytes Using Solid-Phase Microextraction. J. Chromatogr. Sci. 2000, 38(7), 279–288. DOI: 10.1093/chromsci/38.7.279.
- Elmore, J. S.; Mottram, D. S.; Hierro, E. Two-Fibre Solid-Phase Microextraction Combined with Gas Chromatography–Mass Spectrometry for the Analysis of Volatile Aroma Compounds in Cooked Pork. J. Chromatogr. A. 2001, 905(1–2), 233–240. DOI: 10.1016/S0021-9673(00)00990-0.
- Hayward, D. G.; Wong, J. W.; Park, H. Y. Determinations for Pesticides on Black, Green, Oolong, and White Teas by Gas Chromatography Triple-Quadrupole Mass Spectrometry. J. Agric. Food Chem. 2015, 63(37), 8116–8124. DOI: 10.1021/acs.jafc.5b02860.
- Zheng, J.; Liu, B.; Ping, J.; Chen, B.; Wu, H.; Zhang, B. Vortex- and Shaker-Assisted Liquid–Liquid Microextraction (VSA-LLME) Coupled with Gas Chromatography and Mass Spectrometry (GC-MS) for Analysis of 16 Polycyclic Aromatic Hydrocarbons (Pahs) in Offshore Produced Water. Water Air Soil Pollut. 2015, 226, 318. DOI: 10.1007/s11270-015-2575-3.
- Lee, Y. Y.; Shibamoto, T.; Ha, S. D.; Ha, J.; Lee, J.; Jang, H. W. Determination of Glyoxal, Methylglyoxal, and Diacetyl in Red Ginseng Products Using Dispersive Liquid-Liquid Microextraction Coupled with GC-MS. J. Sep. Sci. 2019, 42(6), 1230–1239. DOI: 10.1002/jssc.201800841.
- Zhang, X.; Wang, P.; Xu, D.; Wang, W.; Zhao, Y. Aroma Patterns of Beijing Rice Vinegar and Their Potential Biomarker for Traditional Chinese Cereal Vinegars. Food Res. Int. 2019, 119, 398–410. DOI: 10.1016/j.foodres.2019.02.008.
- Cirlini, M.; Caligiani, A.; Palla, L.; Palla, G. HS-SPME/GC–MS and Chemometrics for the Classification of Balsamic Vinegars of Modena of Different Maturation and Ageing. Food Chem. 2011, 124(4), 1678–1683. DOI: 10.1016/j.foodchem.2010.07.065.
- Durán-Guerrero, E.; Chinnici, F.; Natali, N.; Riponi, C. Evaluation of Volatile Aldehydes as Discriminating Parameters in Quality Vinegars with Protected European Geographical Indication. J. Sci. Food Agric. 2014, 95(12), 23951403. DOI: 10.1002/jsfa.6958.
- Cejudo-Bastante, M. J.; Duràn-Guerrero, E.; Natera-Marìn, R.; Castro-Mejìas, R.; Garcìa-Barroso, C. Characterisation of Commercial Aromatised Vinegars: Phenolic Compounds, Volatile Composition and Antioxidant Activity. J. Sci. Food Agric. 2013, 93, 1284–1302. DOI: 10.1002/jsfa.5885.
- Guerrero, E. D.; Marín, R. N.; Mejías, R. C.; Barroso, C. G. Stir Bar Sorptive Extraction of Volatile Compounds in Vinegar: Validation Study and Comparison with Solid Phase Microextraction. J. Chromatogr. A. 2007, 1167(1), 18–26. DOI: 10.1016/j.chroma.2007.08.039.
- Ishihara, S.; Inaoka, T.; Nakamura, T.; Kimura, K.; Sekiyama, Y.; Tomita, S. Nuclear Magnetic Resonance- and Gas Chromatography/Mass Spectrometry-Based Metabolomic Characterization of Water-Soluble and Volatile Compound Profiles in Cabbage Vinegar. J. Biosci. Bioeng. 2018, 126(1), 53–62. DOI: 10.1016/j.jbiosc.2018.02.003.
- Lu, S.; Cao, Y.; Yang, Y.; Jin, Z.; Luo, X. Effect of Fermentation Modes on Nutritional and Volatile Compounds of Huyou Vinegar. J. Food Sci. Technol. 2018, 55(7), 2631–2640. DOI: 10.1007/s13197-018-3184-0.
- Lee, J.; Jang, H. W.; Jeong, M. C.; Yoo, S. R.; Ha, J. Analysis of Volatile Compounds as Quality Indicators for Fuji Apples after Cold Storage. J. Food Biochem. 2017, 41(6), 1–11. DOI: 10.1111/jfbc.12410.
- Ziegler, M.; Schmarr, H. G. Comparison of Solid-Phase Microextraction Using Classical Fibers versus Mini-Arrows Applying Multiple Headspace Extraction and Various Agitation Techniques. Chromatographia. 2019, 82, 635–640. DOI: 10.1007/s10337-018-3659-1.
- Barreira, L. M. F.; Duporté, G.; Rönkkö, T.; Parshintsev, J.; Hartonen, K.; Hyrsky, L.; Heikkinen, E.; Jussila, M.; Kulmala, M.; Riekkola, M. L. Field Measurements of Biogenic Volatile Organic Compounds in the Atmosphere Using Solid-Phase Microextraction Arrow. Atmos. Meas. Tech. 2018, 11, 881–893. DOI: 10.5194/amt-11-881-2018.
- Kremser, A.; Jochmann, M. A.; Schmidt, T. C. PAL SPME Arrow–Evaluation of a Novel Solid-Phase Microextraction Device for Freely Dissolved PAHs in Water. Anal. Bioanal. Chem. 2016, 408(3), 943–952. DOI: 10.1007/s00216-015-9187-z.