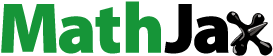
ABSTRACT
Peptides derived from food proteins have exhibited significant antihypertensive effects without side effects. In this study, the cottonseed protein was hydrolyzed by papain for preparing peptide with angiotensin I converting enzyme (ACE) inhibitory activity. The influence of hydrolysis temperature (33°C, 40°C, and 47°C), pH (6.5, 7.0, and 7.5), and enzyme to substrate (E/S) ratio (0.9%, 1.2%, and 1.5%) on the degree of hydrolysis (DH) and ACE-inhibitory activity were analyzed. The hydrolysis conditions were further optimized by central composite design (CCD) and response surface methodology (RSM). The DH of cottonseed protein and ACE inhibitory rate of hydrolysates reached 25.7% and 88.2%, respectively, under the optimal hydrolysis conditions (hydrolysis temperature 39°C, pH 7.5, and E/S ratio 1.04%). We further separated the cottonseed protein hydrolysates (CPH) using a combined strategy of ultrafiltration membrane bioreactor system, sephadex G-25 gel filtration chromatography and reversed-phase high-performance liquid chromatography (RP-HPLC). An ACE inhibitory peptide, named as FII-2-P, with ACE inhibitory IC50 value of 46.7 μg/mL, was obtained. MALDI-TOF-TOF mass spectrometry analysis revealed that the molecular weight of FII-2-P was 763.4 Da and its amino acids sequence was Phe-Pro-Ala-Ile-Gly-Met-Lys. These results demonstrated that the cottonseed protein is a potential source of ACE inhibitory ingredients to be used in the development of functional foods.
Introduction
Hypertension is a common chronic disease, which affects about 26.4% of the world’s adult population, and increases mortality risks.[1] Angiotensin-I converting enzyme is a key member of rennin–angiotensin system. It plays a vital role in regulating blood pressure by converting the inactive decapeptide angiotensin-I to a potent vasoconstrictor octapeptide angiotensin-II meanwhile inactivating the vasodilating nonapeptide, consequently constricting blood vessels and increasing blood pressure.[Citation2] Currently, different synthesized ACE inhibitors have been used as drugs for antihypertensive therapy including lisinopril, captopril, and enalapril. However, these drugs could result in undesirable side effects and risks, such as persistent dry coughs, angioneurotic edema, loss of taste and renal impairment.[Citation3] Therefore, natural ACE inhibitors derived from food proteins have been regarded as a safe alternative and have gained more and more attention. Oshima et al.[Citation4] initially isolated peptides inhibitors of ACE from gelatin digested with bacterial collagenase in 1979. Since then, many other ACE inhibitory peptides have been produced from enzymatic hydrolysates of different food proteins, such as casein, whey protein, mushroom, walnuts meal, wheat protein, fish protein, soybean protein, rapeseed protein, and marine organisms protein etc.[Citation5–Citation21]
Cottonseed protein is the second-best potential source of plant proteins.[Citation22] Six proteases including flavourzyme, neutrase, alcalase, papain, pepsin, and trypsin have been used to digest cottonseed protein for the production of ACE inhibitory peptides. The cottonseed protein hydrolysates (CPH) prepared by papain showed the strongest inhibitory ability of ACE in our previous study.[Citation23] Therefore, papain was applied to hydrolyze cottonseed protein for the production of ACE inhibitory peptides in the present study. Enzymatic hydrolysis of protein is a complicated process being influenced by several factors and their potential interactions, such as enzyme/substrate (E/S) ratio, hydrolysis time, temperature and pH. This work aims to obtain the optimal hydrolysis conditions of CPH prepared by papain using response surface methodology (RSM), isolate and purify the ACE inhibitory peptides by ultrafiltration, gel filtration chromatography (Sephadex G-25) and reversed-phase high performance liquid chromatography (RP-HPLC), and determine the molecular weight and amino acid sequences of the peptide with best ACE inhibitory activity by MALDI-TOF-TOF mass spectrometry.
Materials and methods
Materials
Dephenol and defatted cottonseed meal were obtained from China Cotton-Unis Biotechnology Co. Ltd. (Beijing, China), the composition of the meal was as following: protein (62.32%), carbohydrate (4.71%), mineral (2.83%), fat (0.01%) and moisture (5.04%). Papain enzyme from Carica papaya (10000 U/g) was purchased from Pombo Biological Engineering Co., Ltd. (Nanning, China). Hippuric acid (HA), ACE enzyme powder (2 U/mg protein), glycine and hippuryl -L-histidyl -L-leucine (HHL) were purchased from Sigma-Aldrich Chemical Co. (St. Louis, MO, USA). All other reagents used were of analytical grade.
Preparation of cottonseed protein
Cottonseed protein was extracted with alkaline water (1:15 ratio w/v) at 50°C for 2 h while stirring at a speed of 0.1 rpm with a magnetic stirrer, the pH of solution was adjusted to 10.5 by 1 M NaOH solution. The resultant suspension was clarified by centrifugation at 8,000 rpm and 4°C for 15 min (J-301, Beckman, USA) and the supernatant was collected. Protein precipitate was obtained from the supernatant by adjusting pH to 4.3 with 1 M HCl and collected by centrifugation at 10,000 rpm, 10 min, 4°C. It was then subjected to dialysis to remove salts using a dialysis tube and the resultant material was lyophilized before further use.
Preparation of cottonseed protein hydrolysates
Cottonseed protein hydrolysates were prepared following the method described by Gao et al.[Citation23] with slight modification. Five percent of an aqueous dispersion of cottonseed protein was boiled in a water bath for 15 min prior to enzymatic hydrolysis and then hydrolyzed with papain under varying conditions of hydrolysis time, temperature, pH value and E/S ratio (w/w) that were determined by the experimental design. The hydrolysis pH value was maintained constant by the addition of 0.5 M NaOH continuously. The hydrolysates were further boiled for 10 min in order to inactivate the enzyme and then centrifuged at 8,000 rpm for 5 min. The supernatants were lyophilized and stored in a desiccator for further use.
Determination of DH
The DH of cottonseed protein was determined using the ninhydrin colorimetric method with minor modifications.[Citation24] In this study, glycine was used as the standard instead of leucine. After hydrolysis, 1 mL fresh ninhydrin reagent (ninhydrin reagent was made by dissolving 1.5 g ninhydrin in 10 mL acetate buffer (0.05 M, pH = 4.5) then adding 60 mL ethylene glycol,15 mL n-propanol and 15 mL n-butyl alcohol.) was mixed with 3 mL cottonseed protein hydrolysate solution followed by heating for 20 min, the mixture was cooled in an ice bath and 1 mL ethanol solution (40%, v/v) was then supplemented. The absorbance at 570 nm was measured in a UV-2600 spectrophotometer (Shimadzu Ltd, Japan). The DH value was calculated as follows:
where, AN2 is the concentration of free amino nitrogen of hydrolysate (µM/g); AN1 is the concentration of free amino nitrogen of protein (µM/g); AN0 is the concentration of total amino nitrogen of protein (µM/g).This formula was used for all subsequent calculations of the DH of the samples.
Determination of ACE inhibitory activity
The ACE inhibitory ability of cottonseed protein hydrolysates were determined by the method of Cushman et al.[Citation25] with slight modification. The reaction mixture contained100 μL of 5 M HHL (dissolved in 0.05 M sodium borate buffer containing 0.3 M NaCl, pH 8.3) as a substrate, 50 μL of sample solution, and 150 μL of ACE solution (0.025 U/mL). The mixture was then incubated at 37°C for 60 min, and the reaction was terminated by addition of 250 μL of 1 M HCl. The hippuric acid (HA) released was extracted by 2.0 mL of ethyl acetate, redissolved in 3 mL of 1 M NaCl after removal of ethyl acetate by heat evaporation, and the absorbance was measured at 228 nm by UV-2600 spectrophotometer (Shimadzu Ltd, Japan). The ACE inhibitory activity was calculated as follows:
where AS is the absorbance of the sample, AC represents the absorbance of the control, A0 is the absorbance of the blank. The concentration of peptide in mg/mL required to inhibit 50% of ACE activity was defined as the IC50 value, which was calculated by regression analysis of ACE inhibitory rate (%) versus peptide concentration.
Experimental design for optimization of hydrolysis conditions
In single factor experiments, the effects of the individual factors including hydrolysis time (1.0, 2.0, 3.0, 4.0, 5.0, 6.0, 7.0 and 8.0 h), temperature (20°C, 30°C, 40°C, 50°C, and 60°C), pH value (5.0, 6.0, 7.0, 8.0 and 9.0) and E/S ratio (0.3%, 0.6%, 0.9%, 1.2%, and 1.5%, w/w) on the DH and ACE inhibitory rate were investigated individually. Central composite design (CCD) was then used to predict the effects of three independent variables, i.e., temperature (A), pH value (B), and E/S ratio (C), on DH (Y1) and ACE inhibitory activity (Y2). The variables and their levels are shown in . Each variable was set five levels, and coded as -a, −1, 0, +1, +a. A total of 20 runs were performed in triplicate according to the CCD in , and the average values were used in data analysis. The response variables (Y1, Y2) were related to the coded variables (A, B, C) by the following quadratic equation:
Table 1. Coded and uncoded settings of the process parameters for cottonseed protein hydrolysis, according to a central composite design
Table 2. Central composite design of the independent variables and experimental values of DH (Y1) and ACE inhibitory activity (Y2)
where Y is the predicted response variables (DH and ACEI in real value), β0 is the constant coefficient (intercept), β1, β2, β3 are linear regression coefficients, β11, β22, β33 are squared regression coefficients, and β12, β13, β23 are interaction regression coefficients.
Purification of ACE inhibitory peptide
Cottonseed protein hydrolysates were filtered by sequential filtration using the MSC300 ultrafiltration unit equipped with three molecular weight cut-off membranes of 30, 10 and 5 kDa, respectively. Four fractions were obtained: fraction U-I with molecular weight < 5 kDa, U-II with molecular weight 5–10 kDa, U-III with molecular weight 10–30 kDa, and U-IV with molecular weight > 30 kDa. ACE inhibitory activity of each fraction was evaluated. The fraction with the highest ACE inhibitory activity was then loaded onto the Sephadex G-25 gel filtration chromatography column which was equilibrated with 0.1 M phosphate buffer solution (pH 7.2) under a flow rate of 1.0 mL/min, and monitored at 210 nm. The eluents were collected and the fraction with the highest ACE inhibitory activity was concentrated by freeze-drying, and passed through a RP-HPLC equipped with a semi-preparative C18 column (Zorbax, 9.4 × 250 mm, Agient Technologies, USA) using a linear gradient of 5% acetonitrile containing 0.1% TFA to 60% acetonitrile containing 0.1% TFA in 60 min at a flow rate of 2.0 mL/min. Peaks at 210 nm were identified and the eluents were collected and lyophilized. The purity of fractions with high ACE inhibitory activity was analyzed using a column (Zorbax, C18, 4.6 × 250 mm, Agient Technologies, USA) with a linear gradient of acetonitrile containing 0.1% TFA (5–40%, in 25 min) under a flow rate of 1.0 mL/min. The fraction was collected, lyophilized, and used to identify the amino acid sequence.
Determination the molecular weight and amino acid sequence of the purified ACE inhibitory peptide
A model 4700 Plus MALDI TOF/TOF analyzer (Applied Biosystems, Inc., Foster City, CA, USA) equipped with a nitrogen laser (λ = 337 nm) was applied to determine the molecular mass and amino acid sequence of the purified ACE inhibitory peptide. Mass spectra were obtained for the major peptide ions in MS mode and peptide sequencing were obtained when the spectrometers automatically reverted to MS/MS mode. MS/MS was achieved by 2 kV collision-induced dissociation in positive ion mode. The primary sequence structure of the peptide was determined by the Mascot search algorithm and the De novo sequencing analysis.[Citation26]
Statistical analysis
The results were presented as the mean ± standard deviation derived from three replications. Significant differences between the means of samples were analyzed by one-way analysis of variance (ANOVA) with Duncan’s multiple comparison test. RSM was analyzed using the Design-Expert Software, version 8.06. SPSS software version 13.0 was used to analyze or report the data. All the tests were considered statistically significantly at P< .05.
Results and discussion
Effects of hydrolysis temperature, time, pH and E/S ratio on DH and ACE inhibitory activity
The effects of hydrolysis time on DH and ACE activity of the cottonseed protein hydrolysate were determined at 40°C, pH 7.0 and E/S ratio 0.9%. shows that the DH of cottonseed protein increased linearly (P < .05) from 1 to 4 h, and then reached a plateau with DH of 26.2–27.5% during 4–8 h. The ACE inhibitory rate increased during 0–6 h, then dropped a little during 6–8 h which might be related to the degradation of ACE inhibitory peptides. Therefore, 6 h was selected as the optimal hydrolysis time, which was consistent with the result reported by Mullally et al.[Citation27] Mao et al.[Citation28] also found that when yak milk casein was hydrolyzed for 6 h, the hydrolysate showed the most ACE inhibitory activity of 79.5%, but longer hydrolysis time led the peptides to lost their ability to inhibit ACE. In sum, further treatment may hydrolyze an ACE inhibitory peptides into amino acids and thus destroy its ACE inhibitory activity. As a result, 6 h was ensured as the optimum hydrolysis time.
Figure 1. Effects of hydrolysis time (a), temperature (b), pH (c) and E/S ratio (d) on the DH and ACE inhibitory activity of hydrolysate
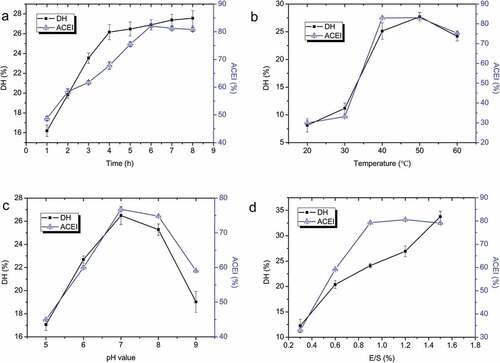
The effects of hydrolysis temperature on DH and ACE inhibitory ability were determined with the hydrolysis time 6 h, initial pH 7.0 and E/S ratio 0.9%. As shown in , the DH of cottonseed protein hydrolysate increased with the temperature (20–50°C), however, both the DH and ACE inhibitory activity were decreased with the further increment of temperature. The lowering ACE inhibitory activity at higher and lower temperatures may be, respectively, explained by protein denaturation and incomplete enzymatic hydrolysis,[Citation29] which indicated that temperature is an important factor in the hydrolysis of cottonseed protein. In this study, the central point was found at 40°C.
Papain is sensitive to alkaline or acidic environments and could only work and reach maximum activity in a specific range of pH. The effects of initial pHs from 5.0 to 9.0 on ACE inhibitory ability and DH were investigated. The hydrolysis temperature, E/S ratio, and hydrolysis time were set at 40°C, 0.9%, and 6 h, respectively. The DH and ACE inhibitory rate of CPH increased when initial pH was from 5.0 to 7.0 and peaked at initial pH of 7.0 (); afterwards, the values of DH and ACE inhibitory activity decreased. Ren et al.[Citation30] verified that papain with pH 7.0 showed the most efficient hydrolysis ability on grass carp sarcoplasmic protein. Our results indicated that pH had a significant influence (P < .05) on the cottonseed protein hydrolysis. Based on our findings, a pH of 7.0 was chosen as the RSM central point ().
reveals the effects of E/S ratio on DH and ACE inhibitory ability of cottonseed protein hydrolysate varied from 0.3% to 1.5%. The DH increased with the increment of E/S ratio and reached 33.76 ± 1.08% at E/S ratio of 1.5%. Differently, ACE inhibitory ability reached a maximum when E/S ratio was 1.2%, and the further increase of E/S caused the decrease of the value of ACE inhibitory rate. The results could be due to greater hydrolysis of the protein when more proteases were added. This is also consistent with earlier findings on the hydrolysis of other kinds of a protein substrate, such as whey protein[Citation31] and dogfish muscle protein.[Citation32] Considering the cost, E/S ratio of 1.2% was selected as the center point ().
Central composite design and response surface analysis modeling
In this experiment, the hydrolysis conditions for the production of ACE inhibitory peptides derived from cottonseed protein were optimized by RSM. The data indicated that the experimental DH and ACE inhibitory rate values fall in the ranges of 15.11–37.73% and 38.04–84.76%, respectively (), which were similar to the results reported by Guo et al.[Citation31] The analyses of variance (ANOVA) for the regression models were summarized using Design-Expert 8.06 software (). The linear terms (A, B and C), quadratic terms (A2, B2, and C2), and interaction terms (AB and BC) had significant effects (P <.05), as shown by the regression coefficients, while the interaction term (AC) had no significant effect on DH values. The linear terms (A, B and C), the quadratic terms (A2, and B2), and the interaction terms (AC and BC) had significant effects (P <.05) on the ACE inhibitory ability. The P value of the two regression models were less than 0.01, implying that the models were extremely significant. Meanwhile, the lack-of-fit values for the two models were no significant (P >.05), indicating that the two models were well adapted to the responses. The coefficient of determination R2 was used to check the fit of the model, which was calculated to be 0.9657, indicating that 96.57% of the variability in the response on DH model could be sufficiently explained. The Adj R-Squared of ACE inhibitory activity was 0.9816, indicating that 98.16% of the variability in the response on the ACE inhibitory activity model could be explained. R-Squared value was closer to 1.00, indicating that the models were well adapted to the responses and had high precision. In sum, the statistical model was built, and the relationships between responses and the test variables were determined to be shown as follows:
Table 3. Analysis of variance for DH (Y1) and ACE inhibitory activity (Y2)
where Y1 is the predicted DH in real value, Y2 is the predicted ACE inhibitory activity in real value, A, B and C are the temperature, E/S ratio and pH, respectively. To determine the optimal levels of each variable for maximum ACE-inhibitory peptide production, three-dimensional response surface plots were constructed by plotting the responses (DH and ACE inhibitory activity) on the Z-axis against any two independent variables, while maintaining other variables at their center values (). The center values for temperature, E/S ratio and pH were 40°C, 1.2%, and 7.0, respectively. presents the interaction effects of temperature and of E/S ratio on DH, with the fixed pH 7.0. DH was enhanced with temperature and E/S ratio. Lower pH (6.5) and higher temperature (47°C) led to a higher DH when E/S ratio was maintained at 1.2% (). Both pH and E/S ratio exerted linear effects on DH at temperature 40°C, and the maximal DH was obtained at E/S ratio of 1.2 and pH 6.0 (). Under the fixed-pH condition, ACE inhibitory ability was firstly increased and then gradually decreased with temperature but constantly increased with E/S ratio (). When E/S ratio was fixed, ACE inhibitory ability increased until temperature reached an optimum point and then decreased with temperature; in addition, a linear effect of pH on the ACE inhibitory ability was observed (). E/S ratio exerted a quadratic effect on the ACE inhibitory ability, yielding the maximum ACE inhibitory ability at 84.8%, whereas the effect of pH was linear at temperature 40°C ().
Figure 2. Response surface three-dimensional plots of the effects of (a, d) temperature and E/S ratio; temperature and pH (b, e); pH and E/S ratio on the DH and ACE inhibitory activity of CPH (c, f)
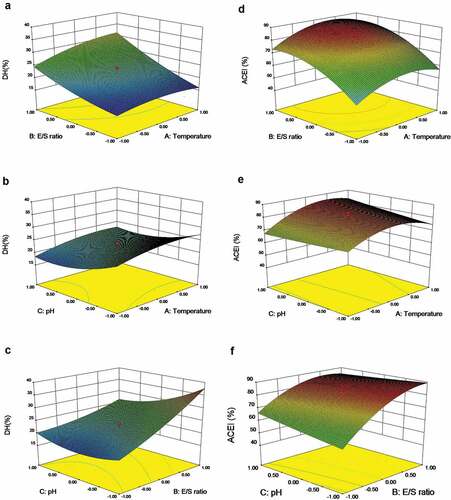
Although the DH values of 25.6% produced the maximal ACE inhibition values (84.8%), the lowest DH values of 15.1% leaded to comparatively lower ACE inhibitory activity (47.8%). The highest DH of 37.7% resulted in an ACE inhibitory activity of 82.1%, a value lower than most of those obtained at lower DH values (). This finding was consistent with the results of Guo et al.[Citation31], who found that higher DH could not lead to higher ACE inhibitory activity. Initially, the hydrolysis may be releasing the ACE inhibitory peptides resulting in an increase in ACE inhibitory activity of the hydrolysates. However, further hydrolysis treatment may result in the decrease of ACE inhibitory ability due to the degradation of ACE inhibitory peptides. Our results were different from the reports of Baharuddin et al.[Citation33] Who found that the highest DH levels induced the highest ACE inhibition and lowest peptide molecular weights (150–800Da). Up to date, no evidence proved any relationship between the DH and ACE inhibitory activity.
Optimization and model validation
In order to obtain the highest ACE inhibitory ability of CPH, the optimization of the model was performed using Design-Expert 8.06 software by setting the maximum ACE inhibitory rate value (Y2) as the goal. For cottonseed protein, optimum hydrolysis conditions were temperature (A) at 38.9°C, E/S ratio (B) of 1.04% and pH (C) of 7.5, under this condition, the predicted values of ACE inhibitory activity and DH were 88.2% and 26.7%, respectively. The experimentally obtained mean ACE inhibitory activity was 87.8 ± 0.23% and DH was 25.5 ± 0.12%. These results were slightly lower than the predicted values, similar to reports on salmon skin.[Citation34] The results confirmed that the RSM approach was appropriate for optimizing the hydrolysis conditions of cottonseed proteins by papain for production of ACE inhibitory activity peptides.
Purification of the ACE inhibitory peptides
In our previous work, the CPH was fractionated into four parts of different molecular weight (U-I, < 5 kD; U-II, 10–5 kD; U-III, 30–10 kD; U-IV, > 30 kD) by using an ultrafiltration membrane bioreactor system. U-I (< 5 kD) exhibited the best ACE inhibitory ability with IC50 value of 0.792 mg/mL, similar to the reports that peptides in the lowest molecular size fractions of protein hydrolysates from black soybean,[Citation14] gram flour[Citation35] and macroalgae[Citation36] had the highest ACE inhibitory activities. Then, the U-I was further separated with gel filtration chromatography (Sephadex G-25) into four fractions (FI, FII, FIII, and FIV). The results showed that FII exhibited the maximum ACE inhibitory activity (IC50 = 160 μg/mL) with the yield of 41.63%.[Citation23] In the present research, as shown in , FII was loaded onto the semi-preparative C18 column with a gradient elution of acetonitrile (5–60% in 60 min) for isolating of peptides by RP-HPLC. Nine peptides, designated as FII-1–FII-9, were collected and their ACE inhibitory activities were individually tested (). The results showed that FII-2 exhibited the highest ACE inhibitory activity (91.4%) than the others at the same concentration (100 μg/mL). Then FII-2 was further purified by the semi-preparative C18 column with a linear gradient of acetonitrile (5–40% in 30 min). This step was repeated for several times until enough samples have been collected. At last an RP-HPLC analytical column was used to confirm the purity of the peptide. As shown in , the fraction with one single peak, named FII-2-P, was obtained with the purity of 95%, and the ACE inhibitory IC50 value of 46.7 μg/mL. The IC50 value of FII-2-P from cottonseed protein was found to be lower than other food protein hydrolysates like yeast (420 µg/mL),[Citation37] algal (255 µg/mL),[Citation38] and small red bean (67.2 µg/mL),[Citation39] but was higher than those of chlorella (15 µg/mL),[Citation40] fresh chickpeas (0.039 µg/mL and 0.307 µg/mL, respectively)[Citation41] and hard-to-cook bean (2.7 µg/mL).[Citation42] Thus, FII-2-P (46.7 μg/mL) could be a potential ACE inhibitor to be used in the development of functional foods.
Table 4. ACE inhibitory activity of FII fraction by semi-preparing RP-HPLC
Molecular weight and amino acid sequence analysis of FII-2-P
ACE inhibitory ability of a peptide is significantly dependent on molecular weight, amino acid composition, and their primary structure.[Citation43] The molecular mass and amino acid sequence of FII-2-P was determined using MALDI TOF/TOF mass spectrometry. As presented in , the molecular weight of FII-2-P was 763.4 Da, and the peptide was composed of seven amino acids with the sequence FPAIGMK (Phe-Pro-Ala-Ile-Gly-Met-Lys). Bhaskar et al.[Citation35] identified two ACE inhibitory peptides derived from horse gram flour after hydrolysis with alcalase. The amino acid sequences of the peptides were TVGMTAKF and QLLLQQ, with IC50 value of 75.0 ± 4.2 µM and 30.3 ± 2.3 µM, respectively, similar to our results. Even though the structure–activity relationship of ACE inhibitory peptides derived from food protein has not been fully identified, several studies have suggested that the binding ability to ACE of these peptides were related to the first three amino acids at C-terminus.[Citation44] The positively charged amino acids (Arg and Lys) and the hydrophobic amino acid residues (Phe, Pro, Try, Val, Tyr, Ala, Leu, and Ile) have been revealed to increase ACE inhibitory activity.[Citation45] Structure–activity relationship analysis also implied that amino acids Ile, Leu, Ala, and Val were preferred at the N-terminus, whereas Phe, Trp, and Tyr were preferred at the C-terminus had a positive effect on potency of ACE inhibitory peptides.[Citation46] This could explain the high ACE inhibitory ability of peptide FPAIGMK (Phe-Pro-Ala-Ile-Gly-Met-Lys) which consists of an aliphatic amino acid (alanine, A) and an aromatic amino acid (phenylalanine, F) at the N-terminus, a positively charged amino acid (lysine, K) at the C-terminal . Ashok and Aparna[Citation47] reported that some longer peptides showed high ACE inhibitory activity while some more hydrophilic ones with possessed low inhibitory activities. Thus, the long peptide FPAIGMK (763.4Da) consisting of seven amino acids without hydrophilic amino acid residues had the high ACE inhibitory ability, possibly because the hydrophilic amino acid residues affected the ACE inhibitory activity by restricting the entry of the peptide into the enzyme active site. However, the structure–activity relationship for the various ACE inhibitory peptides remains inconclusive, and the structure–activity correlations deserve further work.
Conclusion
The enzymatic conditions of papain for cottonseed protein were optimized by RSM. The highest ACE inhibitory activity of 88.2% and DH of 25.7% were obtained under the optimal proteolysis parameters (hydrolysis temperature 39℃, E/S ratio 1.0%, and pH 7.5). The ACE inhibitory peptide (FII-2-P) was isolated and identified as Phe-Pro-Ala-Ile-Gly-Met-Lys (763.4 Da). FII-2-P displayed powerful ACE inhibitory activity with an IC50 value of 46.7 μg/mL, implying that cottonseed protein has the potential in the development of new functional foods.
Declaration of interest
The authors declare that there is no conflict of interest.
Additional information
Funding
References
- Kearney, P. M.; Whelton, M.; Reynolds, K.; Muntner, P.; Whelton, P. K.; He, J. Global Burden of Hypertension: Analysis of Worldwide Data. Lancet. 2005, 365(9455), 217–223. DOI: 10.1016/s0140-6736(05)17741-1.
- Guang, C.; Phillips, R. D.; Jiang, B.; Milani, F. Three Key Proteases – Angiotensin-I-converting Enzyme (ACE), ACE2 and Renin – Within and beyond the Renin-angiotensin System. Arch. Cardiovasc. Dis. 2012, 105(6–7), 373–385. DOI: 10.1016/j.acvd.2012.02.010.
- Antonios, T. F. T.; MacGregor, G. A. Angiotensin Converting Enzyme Inhibitors in Hypertension: Potential Problems. J. Hypertens. 1995, 13(3), 11–16. DOI: 10.1097/00004872-199509003-00003.
- Oshima, G.; Shimabukuro, H.; Nagasawa, K. Peptide Inhibitors of Angiotensin I-converting Enzyme in Digests of Gelatin by Bacterial Collagenase. Biochim. Biophys. Acta. 1979, 566(1), 128–137. DOI: 10.1016/0005-2744(79)90255-9.
- Wu, S.; Feng, X.; Lu, Y.; Lu, Y.; Liu, S.; Tian, Y. Purification of Angiotensin I-converting Enzyme (ACE) Inhibitory Peptides from Casein Hydrolysate by IMAC-Ni2+. Amino Acids. 2017, 49(10), 1787–1791. DOI: 10.1007/s00726-017-2475-5.
- Van der Ven, C.; Gruppen, H.; de Bont, D. B. A.; Voragen, A. G. J. Optimisation of the Angiotensin Converting Enzyme Inhibition by Whey Protein Hydrolysates Using Response Surface Methodology. Int. Dairy. J. 2002, 12(10), 813–820. DOI: 10.1016/s0958-6946(02)00077-8.
- Zhang, P.; Roytrakul, S.; Sutheerawattananonda, M. Production and Purification of Glucosamine and angiotensin-I Converting Enzyme (ACE) Inhibitory Peptides from Mushroom Hydrolysates. J. Funct. Foods. 2017, 36, 72–83. DOI: 10.1016/j.jff.2017.06.049.
- Wang, F. J.; Yin, X. Y.; Regenstein, J. M.; Wang, J. Z. Separation and Purification of angiotensin-I-converting Enzyme (ACE) Inhibitory Peptides from Walnuts (juglans Regia L.) Meal. Eur. Food Res. Technol. 2015, 242(6), 911–918. DOI: 10.1007/s00217-015-2597-5.
- Dadzie, R. G.; Ma, H.; Abano, E. E.; Qu, W.; Mao, S. Optimization of Process Conditions for Production of Angiotensin I-converting Enzyme (ACE) Inhibitory Peptides from Vital Wheat Gluten Using Response Surface Methodology. Food Sci. Biotechnol. 2013, 22(6), 1531–1537. DOI: 10.1007/s10068-013-0248-9.
- Thewissen, B. G.; Pauly, A.; Celus, I.; Brijs, K.; Delcour, J. A. Inhibition of Angiotensin I-converting Enzyme by Wheat Gliadin Hydrolysates. Food Chem. 2011, 127(4), 1653–1658. DOI: 10.1016/j.foodchem.2010.11.171.
- Liu, X.; Zhang, M.; Shi, Y.; Qiao, R.; Tang, W.; Sun, Z. Production of the Angiotensin I Converting Enzyme Inhibitory Peptides and Isolation of Four Novel Peptides from Jellyfish (rhopilema Esculentum) Protein Hydrolysate. J. Agric. Food Chem. 2015, 96(9), 3240–3248. DOI: 10.1002/jsfa.7507.
- Ko, J. Y.; Kang, N.; Lee, J. H.; Kim, J.-S.; Kim, W. S.; Park, S. J.; Kim, Y. T.; Jeona, Y. J. Angiotensin I-converting Enzyme Inhibitory Peptides from an Enzymatic Hydrolysate of Flounder Fish (paralichthys Olivaceus) Muscle as a Potent Anti-hypertensive Agent. Process Biochem. 2016, 51(4), 535–541. DOI: 10.1016/j.procbio.2016.01.009.
- Zhang, Y.; Ma, L.; Otte, J. Optimization of Hydrolysis Conditions for Production of Angiotensin-Converting Enzyme Inhibitory Peptides from Basa Fish Skin Using Response Surface Methodology. J. Aquat. Food Prod. Technol. 2015, 25(5), 684–693. DOI: 10.1080/10498850.2014.919049.
- Li, M.; Xia, S.; Zhang, Y.; Li, X. Optimization of ACE Inhibitory Peptides from Black Soybean by Microwave-assisted Enzymatic Method and Study on Its Stability. LWT - Food Sci. Technol. 2018, 98, 358–365. DOI: 10.1016/j.lwt.2018.08.045.
- Hanafi, M. A.; Hashim, S. N.; Chay, S. Y.; Ebrahimpour, A.; Zarei, M.; Muhammad, K.; Abdul-Hamida, A.; Saari, N. High angiotensin-I Converting Enzyme (ACE) Inhibitory Activity of Alcalase-digested Green Soybean (glycine Max) Hydrolysates. Food Res. Int. 2018, 106, 589–597. DOI: 10.1016/j.foodres.2018.01.030.
- He, R.; Alashi, A.; Malomo, S. A.; Girgih, A. T.; Chao, D.; Ju, X.; Aluko, R. E. Antihypertensive and Free Radical Scavenging Properties of Enzymatic Rapeseed Protein Hydrolysates. Food Chem. 2013, 141(1), 153–159. DOI: 10.1016/j.foodchem.2013.02.087.
- Sun, Z.; Qin, H.; Cao, D.; Yan, X.; Li, H.; Huang, L.; Qu, X.; Kong, C.; Wang, M. Optimization of Hydrolysis Conditions for the Isolation of Angiotensin-I Converting Enzyme (ACE) Inhibitory Peptides from Rhopilema Hispidum. J. Ocean U China. 2018, 17(6), 1458–1464. DOI: 10.1007/s11802-018-3666-8.
- Ko, S. C.; Jang, J.; Ye, B. R.; Kim, M. S.; Choi, I. W.; Park, W. S.; Jung, W. K. Purification and Molecular Docking Study of Angiotensin I-converting Enzyme (ACE) Inhibitory Peptides from Hydrolysates of Marine Sponge Stylotella Aurantium. Process Biochem. 2017, 54, 180–187. DOI: 10.1016/j.procbio.2016.12.023.
- Sun, L.; Wu, S.; Zhou, L.; Wang, F.; Lan, X.; Sun, J.; Tong, Z.; Liao, D. Separation and Characterization of Angiotensin I Converting Enzyme (ACE) Inhibitory Peptides from Saurida Elongata Proteins Hydrolysate by IMAC-Ni2+. Mar. Drugs. 2017, 15(2), 29–39. DOI: 10.3390/md15020029.
- Rasli, H. I.; Sarbon, N. M. Optimization of Enzymatic Hydrolysis Conditions and Characterization of Shortfn Scad (decapterus Macrosoma) Skin Gelatin Hydrolysate Using Response Surface Methodology. Int. Food Res. J. 2018, 25(4), 1541–1549.
- Ishak, N. H.; Sarbon, N. M. Optimization of the Enzymatic Hydrolysis Conditions of Waste from Shortfin Scad (decapterus Macrosoma) for the Production of Angiotensin I-converting Enzyme (ACE) Inhibitory Peptide Using Response Surface Methodology. Int. Food Res. J. 2017, 24(4), 1735–1743.
- Ahmad, S.; Anwar, F.; Hussain, A. I.; Ashraf, M.; Awan, A. R. Dose Soil Salinity Affect Yield and Composition of Cottonseed Oil. J. Am. Oil Chem. Soc. 2007, 84, 845–851. DOI: 10.1007/s11746-007-1115-8.
- Gao, D.; Chang, T.; Li, H.; Cao, Y. Angiotensin I-converting Enzyme Inhibitor Derived from Cottonseed Protein Hydrolysate. Afr. J. Biotechnol. 2010, 9(53), 8977–8983. DOI: 10.5897/AJB10.119.
- Pearce, K. E. N.; Karahalios, D. A.; Friedman, M. E. Ninhydrin Assay for Proteolysis in Ripening Cheese. J. Food Sci. 1988, 53(2), 432–435. DOI: 10.1111/j.1365-2621.1988.tb07723.x.
- Cushman, D. W.; Cheung, H. S. Spectrophotometric Assay and Properties of the Angiotensin-converting Enzyme of Rabbit Lung. Biochem. Pharmacol. 1971, 20(7), 1637–1648. DOI: 10.1016/0006-2952(71)90292-9.
- Rajeswari Prabha, M.; Ramachandramurty, B. Sequence Determination of a Novel Tripeptide Isolated from the Young Leaves of Azadirachta Indica A. Juss. Int. J. Pept. 2013, 2013, 1–6. DOI: 10.1155/2013/629549.
- Mullally, M. M.; Meisel, H.; FitzGerald, R. J. Angiotensin-I-converting Enzyme Inhibitory Activities of Gastric and Pancreatic Proteinase Digests of Whey Proteins. Int. Dairy. J. 1997, 7(5), 299–303. DOI: 10.1016/s0958-6946(97)00018-6.
- Mao, X. Y.; Ni, J. R.; Sun, W. L.; Hao, P. P.; Fan, L. Value-added Utilization of Yak Milk Casein for the Production of angiotensin-I-converting Enzyme Inhibitory Peptides. Food Chem. 2007, 103(4), 1282–1287. DOI: 10.1016/j.foodchem.2006.10.041.
- Roslan, J.; Kamal, S. M. M.; Yunos, K. F. M.; Abdullah, N. Optimization of Enzymatic Hydrolysis of Tilapia Muscle (oreochromis Niloticus) Using Response Surface Methodology (RSM). Sains Malays. 2014, 43, 1715–1723.
- Ren, J.; Zhao, M.; Shi, J.; Wang, J.; Jiang, Y.; Cui, C.; Kakuda, Y.; Xue, S. J. Optimization of Antioxidant Peptide Production from Grass Carp Sarcoplasmic Protein Using Response Surface Methodology. LWT - Food Sci. Technol. 2008, 41(9), 1624–1632. DOI: 10.1016/j.lwt.2007.11.005.
- Guo, Y.; Pan, D.; Tanokura, M. Optimisation of Hydrolysis Conditions for the Production of the angiotensin-I Converting Enzyme (ACE) Inhibitory Peptides from Whey Protein Using Response Surface Methodology. Food Chem. 2009, 114(1), 328–333. DOI: 10.1016/j.foodchem.2008.09.041.
- Diniz, F. M.; Martin, A. M. Use of Response Surface Methodology to Describe the Combined Effects of pH, Temperature and E/S Ratio on the Hydrolysis of Dogfish (squalus Acanthias) Muscle. Int. J. Food Sci. Technol. 1996, 31(5), 419–426. DOI: 10.1046/j.1365-2621.1996.00351.x.
- Baharuddin, N. A.; Halim, N. R. A.; Sarbon, N. M. Effect of Degree of Hydrolysis (DH) on the Functional Properties and Angiotensin I-converting Enzyme (ACE) Inhibitory Activity of Eel (monopterus Sp.) Protein Hydrolysate. Int. Food Res. J. 2016, 23(4), 1424–1431.
- Gu, R. Z.; Li, C. Y.; Liu, W. Y.; Yi, W. X.; Cai, M. Y. Angiotensin I-converting Enzyme Inhibitory Activity of Low-molecular-weight Peptides from Atlantic Salmon (salmo Salar L.) Skin. Food Res. Int. 2011, 44(5), 1536–1540. DOI: 10.1016/j.foodres.2011.04.006.
- Bhaskar, B.; Ananthanarayan, L.; Jamdar, S. Purification, Identification, and Characterization of Novel Angiotensin I-converting Enzyme (ACE) Inhibitory Peptides from Alcalase Digested Horse Gram Flour. LWT - Food Sci. Technol. 2019, 103, 155–161. DOI: 10.1016/j.lwt.2018.12.059.
- Paiva, L.; Lima, E.; Neto, A. I.; Baptista, J. Isolation and Characterization of Angiotensin I-converting Enzyme (ACE) Inhibitory Peptides from Ulva Rigida C. Agardh Protein Hydrolysate. J. Funct. Foods. 2016, 26, 65–76. DOI: 10.1016/j.jff.2016.07.006.
- Mirzaei, M.; Mirdamadi, S.; Ehsani, M. R.; Aminlari, M.; Hosseini, E. Purification and Identification of Antioxidant and ACE-inhibitory Peptide from Saccharomyces Cerevisiae Protein Hydrolysate. J. Funct. Foods. 2015, 19, 259–268. DOI: 10.1016/j.jff.2015.09.031.
- Cao, D.; Lv, X.; Xu, X.; Yu, H.; Sun, X.; Xu, N. Purification and Identification of a Novel ACE Inhibitory Peptide from Marine Alga Gracilariopsis Lemaneiformis Protein Hydrolysate. Eur. Food Res. Technol. 2017, 243(10), 1829–1837. DOI: 10.1007/s00217-017-2886-2.
- Rui, X.; Boye, J. I.; Simpson, B. K.; Prasher, S. O. Purification and Characterization of Angiotensin I-converting Enzyme Inhibitory Peptides of Small Red Bean (phaseolus Vulgaris) Hydrolysates. J. Funct. Foods. 2013, 5(3), 1116–1124. DOI: 10.1016/j.jff.2013.03.008.
- Lin, Y. H.; Chen, G. W.; Yeh, C.; Song, H.; Tsai, J. S. Purification and Identification of Angiotensin I-converting Enzyme Inhibitory Peptides and the Antihypertensive Effect of Chlorella Sorokiniana Protein Hydrolysates. Nutrients. 2018, 10(10), 1397. DOI: 10.3390/nu10101397.
- Medina-Godoy, S.; Ambriz-Pérez, D. L.; Fuentes-Gutiérrez, C. I.; Germán-Báez, L. J.; Gutiérrez-Dorado, R.; Reyes-Moreno, C.; Valdez-Ortiz, A. Angiotensin-converting Enzyme Inhibitory and Antioxidative Activities and Functional Characterization of Protein Hydrolysates of Hard-to-cook Chickpeas. J. Sci. Food Agr. 2012, 92(9), 1974–1981. DOI: 10.1002/jsfa.5570.
- Ruiz-Ruiz, J.; Dávila-Ortíz, G.; Chel-Guerrero, L.; Betancur-Ancona, D. Angiotensin I -converting Enzyme Inhibitory and Antioxidant Peptide Fractions from Hard-to-cook Bean Enzymatic Hydrolysates. J. Food Biochem. 2011, 37(1), 26–35. DOI: 10.1111/j.1745-4514.2011.00594.x.
- Del M. Contreras, M.; Hernández-Ledesma, B.; Amigo, L.; Martín-Álvarez, P. J.; Recio, I. Production of Antioxidant Hydrolyzates from a Whey Protein Concentrate with Thermolysin: Optimization by Response Surface Methodology. LWT - Food Sci. Technol. 2011, 44(1), 9–15. DOI: 10.1016/j.lwt.2010.06.017.
- Iwaniak, A.; Minkiewicz, P.; Darewicz, M. Food-originating Ace Inhibitors, Including Antihypertensive Peptides, as Preventive Food Components in Blood Pressure Reduction. Compr. Rev. Food Sci. F. 2014, 13(2), 114–134. DOI: 10.1111/1541-4337.12051.
- Daskaya-Dikmen, C.; Yucetepe, A.; Karbancioglu-Guler, F.; Daskaya, H.; Ozcelik, B. Angiotensin-I-converting Enzyme (ace)-inhibitory Peptides from Plants. Nutrients. 2017, 9(4), 316. DOI: 10.3390/nu9040316.
- Toopcham, T.; Mes, J. J.; Wichers, H. J.; Roytrakul, S.; Yongsawatdigul, J. Bioavailability of Angiotensin I-converting Enzyme (ACE) Inhibitory Peptides Derived from Virgibacillus Halodenitrificans SK1-3-7 Proteinases Hydrolyzed Tilapia Muscle Proteins. Food Chem. 2017, 220, 190–197. DOI: 10.1016/j.foodchem.2016.09.183.
- Ashok, N. R.; Aparna, H. S. Empirical and Bioinformatic Characterization of Buffalo (bubalus Bubalis) Colostrum Whey Peptides & Their Angiotensin I-converting Enzyme Inhibition. Food Chem. 2017, 228, 582–594. DOI: 10.1016/j.foodchem.2017.02.032.