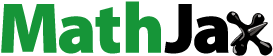
ABSTRACT
Crystalline pattern, granular morphology, physicochemical and pasting properties of banana starch and flour from three cooking banana cultivars (Kapas, Kepok and Nangka) and one dessert banana cultivar (Ambon) were compared. The result showed that cooking banana had a B-type crystalline pattern, while the dessert banana exhibited a C-type crystalline pattern. The flour exhibited higher crystallinity than the extracted starch. Nangka and Ambon starches possessed similar crystallinity (33–34%) while the crystallinity of Kapas starch (38.6%) was close to that of Kepok starch (39.4%). Elongated shape was typical of the starch granules of Kapas while spheroidal shape was seen in the rest of the cultivars. Cultivar did not significantly affect the swelling volume (SV) and solubility (except in Ambon cultivar) (p > .05) but determined water absorption capacity (WAC) and freeze-thaw stability (FTS) (p > .05). Ambon cultivar was the most stable to retrogradation while Kepok was the least stable which may be attributed to the different contents of amylose. Pasting properties, color and texture characteristics of starch were dependent on their cultivars and the presence of non-starch components. Non-starch contents increased the functional properties, pasting point and breakdown viscosity but decreased texture characteristics and setback viscosity. Kepok was the most resistant to gelatinization. Overall granule shape and starch crystalline type did not play any role in the different responses to the properties examined in the study but amylose content may have contributed to the freeze-thaw stability while crystallinity may have affected the starch pasting point.
Introduction
Starch plays an important role in the human diet. Apart from the commonly used starch sources such as cassava, wheat, rice, sweet potato, corn and potato, banana is an alternative source of starch which has the potential to be a major source due to its abundance in tropical countries. India is considered to be a leading country in producing banana.[Citation1] In Indonesia, according to BPS-Statistics Indonesia,[Citation2] banana production in the country increased from 6.13 million tons (2011) to 7.01 million tons (2016) with the West and East Java as the centers of banana production. Such high volume of production will have a potentially huge economic impact. Starch content of unripe banana is known to be as much as 60% (dry basis),[Citation3] with a solids content of around 40–70%.[Citation4,Citation5]
Several studies have reported that cultivar, regional climatic conditions and harvesting periods of banana affect physicochemical and pasting properties of banana starch.[Citation6–Citation8] Several previous studies have reported banana starch characteristics from different cultivars grown in several countries, such as South Africa,[Citation9] Vietnam,[Citation10] Mexico,[Citation11] Thailand,[Citation8] Brazil,[Citation6,Citation12,Citation13] and China.[Citation14] Based on the studies mentioned above, banana starch from different countries have different characteristics. Given that there are a number of cultivars grown in Indonesia with negligible literature available on the indigenous banana varieties, it is important to explore different cultivars of banana commonly consumed in the country. The information obtained in the study described here is expected to contribute to the existing data available worldwide, hence more choices for food industrial applications.
In this study, the role of non-starch components present in different cultivars on physicochemical and pasting properties of banana flour was also examined. Non-starch components include protein, fat, ash, fiber, pectin, cellulose, phenolic acids, etc.[Citation15,Citation16] These components may differ in quantity from variety to variety thereby showing different physicochemical and pasting properties.[Citation12,Citation13,Citation15] Certain compounds may even be present in certain cultivars but absent in the others. It is thus interesting to examine the role of non-starch components in different cultivars on the physicochemical and pasting properties of starch.
In the banana family, there are two types of bananas, namely, cooking banana (plantain) and dessert banana. Dessert banana is usually consumed when it is fully ripe while the other is intended only for cooking. Here, in this study, we used two types of banana with three genomic groups consisting of three cooking bananas and one dessert banana. The physicochemical and pasting properties were evaluated in the form of both starch and flour. As such, we were able to reveal the effect of not only cultivar but also the role of starch granules along with their inhern structure as well as non-starch contents on the properties.
Materials and methods
Materials
Two triploid genotypes Musa AAB (Kapas and Nangka), one triploid genotype Musa ABB (Kepok) (also known as cooking banana) and one triploid Musa AAA (Ambon) (also known as dessert banana) in a green ripeness state (ripening stage 1) harvested between 8 and 10 weeks after flowering were purchased from a local farmer (Jati Gede, Indonesia). The purchase of bananas from local farmers in the same region ensured that the climate conditions were similar. Fruit fingers were selected for uniformity of shape, size and color. Starch and flour production was carried out immediately after the purchase of the fresh samples.
Banana starch isolation
Starch from unripe banana from four cultivars were isolated using the procedure of Chávez-Salazar et al.[Citation17] with a slight modification. The fruits were peeled and sliced into 2 cm pieces; they were then immersed in water at a pulp/water ratio of 1 kg/3 L for 15 min. The pulp was homogenized in a Waring blender (Phillips HR2116/01, Indonesia) at medium speed for 2 min; the homogenate was sieved through muslin cloth until the wash water was clear. Then, the homogenate was allowed to precipitate for 24 h. After decanting, the starch was washed and precipitated again which was then dried in a drying oven at 50°C for 24 h; it was ground and sieved (100 mesh) and finally stored in a polypropylene plastic bag as primary packaging. This plastic was placed into a secondary packaging of aluminum foil along with silica gel using ziplock bags and stored at room temperature (26 ± 2°C) for later analysis.
Banana flour preparation
Banana flour preparation method was followed according to Yangılar[Citation18] with a slight modification. The unripe banana fruit was peeled and the pulp was sliced into pieces approx. 2 mm thick, then dipped in water for 15 min, drained and dried at 50°C for 24 h in a drying oven. After that, the banana slices were milled using miller machine FCT Z-300 to produce flour and passed through 100 mesh screens. Banana flour was stored in a polypropylene plastic bag as primary packaging using aluminum foil along with silica gel as secondary packaging and stored at room temperature (26 ± 2°C) for later analysis.
Chemical composition of banana starch and flour
Moisture content was determined using a modified version of the AOAC 925.04[Citation19] by drying 2 g of sample at 105°C in an air oven for 24 h. The water content was then gravimetrically determined. Protein content was determined using the Kjeldahl method, AOAC 981.10[Citation19] while fat content using a Soxhlet extractor. Starch content was determined by direct acid hydrolysis method[Citation20] while amylose content by International Rice Research Institute (IRRI) method.[Citation21]
X-ray diffraction (XRD)
X-ray diffraction pattern of banana starch and flour was measured using PANalytical X’Pert PRO series PW3040/x0 that operated using Cu-K alpha radiation with a wavelength of 1.5406 nm as X-ray source at 30 mA and 40 kV. The diffraction angle (2θ) scanning was from 3.0084° to 49.9734° with a scanning step time of 2.905 s. Crystallinity was calculated using a Sigma plot program as reported in the literature.[Citation22]
Granule morphology determined
Granule morphology of banana starch and flour was determined using scanning electron microscopy (JEOL JSM-6360 LA at 15kV). The sample was sprayed onto an aluminum plate and coated with gold at 8–10 mA for 10–15 min. Representative digital images of starch granules were obtained at 500× magnification.
Functional properties
Swelling volume and solubility measurement: Swelling volume and solubility was measured using the method of Marta et al.[Citation23] First of all 0.35 g (dry basis) of sample was placed into a centrifuge tube and 12.5 mL of distilled water was added. Sample was mixed using a vortex mixer for 20 s, heated in a water bath at 92.5°C and stirred regularly for 30 min. Then, the sample was cooled for 1 min in ice water and centrifuged at 2050 g for 15 min. The supernatant was separated, and its volume was measured. The supernatant was dried in a drying oven to measure the percentage of solubility. Swelling volume and solubility are calculated using EquationEquations 1(1)
(1) and Equation2
(2)
(2) .
Water absorption capacity (WAC) measurement: Water absorption capacity was measured using the method by Marta et al.[Citation23] 10 mL of distilled water was added to 1 g of sample in a centrifuge tube, then mixed using a vortex mixer. The sample was conditioned at room temperature (26 ± 2°C) for 1 h and then centrifuged at 2050 g for 30 min. The volume of supernatant was measured and WAC is calculated using EquationEquation 3(3)
(3) .
Freeze-thawing stability (syneresis) measurement: Distilled water was added to starch in centrifugal tubes (5% w/v). The starch solution was placed in a water bath and heated to 95°C for 30 min with constant agitation, then cooled to room temperature (26 ± 2°C) in ice water. After that 10 g of starch paste was taken and placed in a centrifuge tube. Next, the sample was stored at 4°C for 24 h, then frozen at −20°C for 48 h. The frozen starch was thawed at room temperature for 3 h and separated by centrifugation at 2050 g for 15 min. Then, the separated water was weighed and syneresis is calculated using EquationEquation 4(4)
(4) .[Citation24]
Texture and color measurement
The sample paste formed from RVA analysis was used to analyze gel texture profile of banana starch and flour. The paste was poured into a pipe container (2.5 cm diameter, 3 cm depth) and stored in a refrigerator at 4°C for 24 h. The gel formed was firstly removed from the container and then tested using TA-XT2 texture analyzer (Stable Micro System, Surrey, UK) using the texture profile analysis (TPA) project and exponent lite express software for data collection and calculation. The gel was pressed using an aluminum cylinder probe P36R with a 2 kg load cell at a speed of 5 mm/s to a distance of 10 mm. The gel texture profile including hardness, cohesiveness, springiness and gumminess was determined from exponent lite express software.
The color of banana starch and flour samples were measured using a Spectrophotometer CM 5 (Konica Minolta Co., Osaka, Japan) with Spectra Magic software. The color measurement includes L* (lightness, 0 = black/100 = white), a* (+a* = redness/-a* = greenness), b* (+b* = yellowness/-a* = blueness) and hue. The calibration was performed with a zero-calibration plate (CM-A124) and white calibration plate (CM-A120) with a large target mask (CM-A203). EquationEquation 5(5)
(5) is used to calculate the whiteness value.[Citation25]
Pasting properties analysis
Rapid Visco Analyzer (RVA StarchMaster 2, Warriewood, Australia) using the standard heating program 1 was used to analyze pasting properties of banana starch and flour samples. A portion amount of distilled water and sample was added into an RVA canister and then stirred to homogenize the mixture before running the test. Sample and water weights were corrected for the sample moisture content (reference moisture content of 14%) with the standard sample and water weight being 3 g and 25 g, respectively. The correction was applied to give a constant dry weight according to the manufacturer’s guide for the instrument. The correction formulae (EquationEquations 6(6)
(6) and 7) are
where S0 = standard sample weight (3 g), S1 = corrected sample weight (g), W0 = standard water weight (25 g), W1 = corrected sample weight (g), M0 = reference moisture content of sample (14%),
M1 = actual moisture content of sample (%). The test was started by equilibrating the starch at 50°C for 1 min., then heated to 95°C for 3.7 min., held at 95°C for 2.5 min., cooled to 50°C in 3.8 min. and kept at 50°C for 2 min. At first, after 10 s, the paddle was rotated at 960 rpm to disperse the starch. After that, the paddle was rotated at 160 rpm. The viscosity was expressed as centipoise (cP). From the graph, following were evaluated: pasting temperature (PT), peak viscosity (PV), holding viscosity (HV), breakdown (BD), final viscosity (FV) and setback (SB).
Statistical analysis
Data were expressed as mean ± SD quadruplicate. The data were analyzed by one-way analysis of variance (ANOVA) to compare physicochemical and pasting properties between four banana cultivars in the same form (flour or extracted starch) and submitted to the mean comparison test by Duncan, at a significance level of 5% (P < .05). Whereas independent-samples T-test (two-sample T-test) was used to compare physicochemical and pasting properties between different forms (starch or flour form) in the same cultivars (Kapas, Kepok, Ambon or Nangka). One-way ANOVA and independent-samples T-test were analyzed using SPSS 19.0 Statistical Software Program.
Results and discussion
Chemical composition of banana starch and flour
The composition of banana starch and flour is presented in . Moisture content of both flour and extracted starch ranged from 6.8% to 8.9%. The flour tended to have slightly lower moisture content compared to the extracted starch. Protein content was reduced from 2% to 3% in the flour to 1–1.6% in the starch. Fat content in flour which was approximately 0.5–0.6% was much lower than protein content. The fat content in the starch ranged from 0.1% to 0.2%. The much lower content of fat suggested that protein might play a more significant role in the starch or flour properties although for certain properties attributed to starch crystallinity, fat has been reported to form a complex with amylose following heat treatment contributing to the slight increase in crystallinity.[Citation26] The contents of protein and fat of banana flour in this study (varieties cultivated in Indonesia) are similar to those found in Brazil.[Citation13]
Table 1. Chemical composition of banana flour and starch from four banana cultivars
Starch content in the flour and extracted starch were 72–81% and 81.5–90%, respectively. Kapas cultivar contained the highest starch content while the rest of the cultivars had similar starch contents. Amongst the four cultivars, Ambon had the lowest amylose content (32.6%), while the amylose content of Kepok was approximately 40.9%. The amylose content of Nangka and Kapas cultivars were 37.8% and 38.6%, respectively. Amylose content in flours was not measured directly. It is however possible to calculate the content in the flour by taking into account the amylose content in the extracted starch.
X-ray diffraction patterns and relative crystallinity
X-ray diffraction patterns of banana starch and flour are shown in . All cultivars of banana starch and flour except Ambon cultivar showed a B-type crystalline structure characterized by the presence of a small peak at 5°, strong peaks at 15° and 17° and a broad peak at 22–24° 2θ. Meanwhile, the peak at 5° was absent in the x-ray diffraction pattern of Ambon flour and starch suggesting that the Ambon cultivar had a C-type crystalline structure. Several previous studies have reported that native banana starch exhibited different types of crystalline structures such as B-type,[Citation6,Citation8,Citation10,Citation22,Citation27,Citation28] C-type,[Citation29,Citation30] and A-type.[Citation31] The type of crystalline structure depends on the cultivar of banana, plant growth condition, isolation technique and other factors.[Citation6,Citation27] In the study described here, we found that the three cultivars from the cooking banana type displayed a B type crystalline structure while the dessert banana had a C-type crystalline structure.
Figure 1. XRD diffractograms of flour and starch from four cultivars: KaS = Kapas starch, KeS = Kepok starch, AmS = Ambon starch, NaS = Nangka starch, KaF = Kapas flour, KeF = Kepok flour, AmF = Ambon flour, NaF = Nangka flour
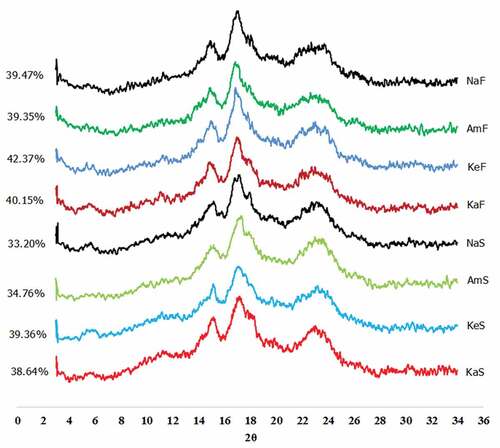
In terms of crystallinity, Nangka cultivar exhibited the lowest crystallinity (33.2%). The crystallinity of Nangka was close to that of Ambon which was approximately 34.8%. Kepok cultivar displayed the highest crystallinity (39.4%) and showed a similar crystallinity to Kapas cultivar which was approximately 38.6%. In the flour forms, slight increase in the crystallinity was observed compared to the starch forms suggesting the contribution of non-starch content to the crystallinity as observed in another study.[Citation22]
Granule morphology
Granule morphology of banana flour and starch observed by Scanning Electron Microscope (SEM) are presented in . The granules of the four banana cultivars had dense surfaces with a small thin layer, which consisted of non-starch components on the flour granules while it was smoother on the starch granules. Pelissari et al.[Citation29] reported that fiber could be seen in the microstructure of banana flour observed by SEM. No evidence of cracks was observed but sizes of the granules varied ranging from 5.5 to 59 μm. Our observation was in agreement with previously reported studies that the granule size ranged from 3.33 to 60 μm.[Citation3,Citation7,Citation10,Citation26,Citation27]
All of the granules exhibited an irregular shape and appeared as elongated and spheroidal shapes, which were in line with several previous studies.[Citation6,Citation10,Citation29,Citation27] Elongated shape was however mainly found in the granule of Kapas cultivar while spheroidal shape was predominantly present in the granules of the rest of the cultivars.
Functional properties
Effect of cultivars on functional properties: Functional properties of banana flour and starch are presented in . Functional properties of banana starch in this study included swelling volume, solubility, water absorption capacity and freeze-thaw stability (measured by the extent of syneresis). To understand the role of cultivar on functional properties it is easier to examine the difference in the properties of the starch than the flour. As such the difference in the response of the starch could be linked to the difference in starch structure and its morphology while the difference in the flour suggested the influence of non-starch components. Therefore, we may conclude that the different properties of different cultivars may be attributed to the difference in the starch granule morphology or starch structure.
Table 2. Functional properties of banana flour and starch from four banana cultivars
The result showed that cultivars did not significantly affect the swelling volume (ranging from 15.33 to 16.07 mL/gdb) and solubility (except in Ambon cultivar) suggesting that the difference in starch granule morphology or starch structure did not play any role in determining such properties. Furthermore, water absorption capacity (WAC) and particularly freeze-thaw stability (FTS) were dependent on the banana cultivar. It means that the starch morphology or starch structure affected WAC and FTS.
It can be concluded based on SEM results that it was not granular morphology that affected WAC and FTS from different cultivars, neither was starch crystalline type as according to XRD results it was only the Ambon cultivar that had C-type while the others exhibited B-type. Therefore, the difference in the functional properties might be related to the starch structure or amylose content as reported in a study where molecular weight distribution, length of branches, degree of branching and structure of starch molecules affected the functional properties of starch.[Citation32]
Amongst the cultivars, Ambon was the most stable to retrogradation with the lowest syneresis (approximately 0.6%). The high stability of Ambon cultivar might be attributed to the low amylose content. This is confirmed by the lowest stability to retrogradation of Kepok cultivar which contained the highest amylose content. The effect of low amylose on increasing banana starch stability to retrogradation was also observed in another study.[Citation13] Retrogradation has been reported to occur more rapidly with amylose than with amylopectin due to the smaller size of unbranched amylose.[Citation33]
Effect of non-starch components on functional properties: Most of the banana flour samples had higher swelling volume, solubility, water absorption capacity and syneresis than banana starch samples. It indicated that non-starch components affected functional properties. These results were in agreement with another study, which found that swelling volume, solubility and WAC of banana flour were higher than in banana starch.[Citation22,Citation34] Swelling volume is the ability of starch granule to hydrate under excess water condition.[Citation32] The increase in the swelling volume was however very small. Meanwhile, twice the increase in the solubility and WAC was observed. The increase of WAC in the flour forms might be related to the presence of pectin. The pectin content was however not measured in this study. Literature reported that banana flour contained pectin of approximately 3.29–5.61% db.[Citation35] Furthermore, Parimalavalli and Babu[Citation34] have reported that protein content might be one of the responsible factors contributing to the high WAC of banana flour. In this study, the protein content in banana flours ranged from 2% to 3% while it was 1–1.6% in the extracted starches. The presence of polar amino acids in the protein could be attributed to the hydrophilicity hence higher affinity to water.
In the starch, solubility indicated the amount of amylose leaching out from the starch granule. In the flours, however, solubility might reflect not only amylose leaching but also non-starch components which were removed from the granule surface and suspended into water during heating treatment and centrifugation. This may explain why the solubility of the banana flour in this study was higher than that of its counterpart. In terms of freeze-thaw stability, it was observed that the presence of non-starch components decreased the stability of starch to retrogradation indicated by the higher syneresis. The non-starch components therefore apparently facilitated the starch recrystallization to take place more easily. Fat content may not be the non-starch component responsible for the increase in syneresis because fat has been reported to form a complex with amylose[Citation36] which then retards the syneresis.
Gel texture and color properties
Effect of cultivars on gel texture and color properties: The textural properties of gel and color characteristics from banana starch and flour are presented in . Similar to the previous section, to understand the role of cultivar on texture characteristics it is easier to examine the difference in the properties in both flour and starch. The role of non-starch content is discussed in the later section. The textural properties of the gel from banana starch investigated in this study included hardness, springiness, cohesiveness and gumminess. According to definitions of mechanical parameters, texture hardness, cohesiveness and springiness were classified as primary properties while gumminess is a secondary property. Hardness is the force necessary to attain a given deformation. Springiness is the rate at which a deformed material goes back to its undeformed condition after the deforming force is removed. Whereas gumminess is the energy required to disintegrate a semi-solid food to a state ready for swallowing. This parameter is dependent on hardness and cohesiveness.[Citation37]
Table 3. Texture and color characteristics of banana flour and starch from four banana cultivars
It was clear from the result that the values of hardness and gumminess of various cultivars differed significantly. Meanwhile, springiness of Kapas and Kepok was not significantly different (p > .05). Although the difference in the cohesiveness was small, the cohesiveness may be classified into two groups in which Ambon and Nangka had similar cohesiveness to Kapas and Kepok in the other group. Overall the difference in the cultivar affected the gumminess and hardness remarkably but not cohesiveness or springiness. In other words, the hardness and gumminess of starch were more sensitive to the difference in the starch characteristics while springiness and cohesiveness were less sensitive.
Looking at the SEM result it was obvious that in terms of granule shape Kapas starch granule was the only cultivar which had a different shape while the granule shape of the other cultivars was similar. It leads to a conclusion that the difference in the hardness and gumminess was not related to the granule morphology. XRD results further confirmed that the difference in the hardness and gumminess exhibited positive correlation with relative crystallinity but it might not be attributed to the crystalline type. Kepok with the highest relative crystallinity exhibited the hardest gel. Similar correlation was observed for the other cultivars. Furthermore given that, gumminess value is a secondary parameter whose value was dependent on the hardness and cohesiveness value, it can be concluded that the different gumminess values were due to the difference in the hardness and not cohesiveness. Furthermore comparing the extent of syneresis, it was evident that the lowest syneresis was exhibited by Ambon cultivar, not Nangka, suggesting that the hardness value did not correlate with the syneresis.
Regarding color characteristics, it was obvious that all banana cultivars in both flour and starch forms were significantly different. Kepok flour and starch have the highest whiteness, L*, and hue value amongst the other cultivars. The highest whiteness and L* value might be due to the lower phenolic content in Kepok cultivar. According to Fatemeh,[Citation38] phenolic content in banana varied depending on the cultivar. The phenolic content was however not measured in this study.
Effect of the non-starch component on gel texture and color properties: Comparing the values of hardness, springiness, cohesiveness and gumminess of starch gel with those of flour gel it was noted that the values tended to decrease in the presence of non-starch components. The gels from all cultivars showed a decrease in gumminess and became less cohesive when non-starch components were present. The cohesive property of starch was apparently shielded by non-starch components present on the surface of the granule. Meanwhile, the hardness of Nangka and Kepok cultivar was not affected by the presence of non-starch components. In addition, the springiness of Kepok flour was independent of the non-starch components.
Comparing the parameters between cultivars in the flour form, there was no role played by the cultivar in the springiness and cohesiveness of flour gels as the values were not significantly different (p > .05). There was however a difference which could be noted in the starch form. It suggested that it is more difficult to distinguish between one cultivar and another by measuring springiness and cohesiveness in the flour form. Therefore, starch form is more reliable to distinguish one cultivar from another.
In terms of color parameters, it was observed that the whiteness and lightness of Kepok and Kapas cultivars were lower in the flour form than in the starch. These values (except the lightness of Ambon) were however higher in the flour of Ambon and Nangka than in their starches. Results suggested that non-starch components contributing to the color characteristics present in the flours of Ambon and Nangka were quantitatively different from those in Kapas and Kepok. It is not clear what component contributes significantly to this characteristic although fat, protein and phenolic content may partly contribute.
Pasting properties
Effect of cultivars on pasting properties: Pasting properties of banana flour and starch from four cultivars are presented in and . The results showed that cultivars determined the pasting properties. Our finding was in line with a previous study on banana starches from different cultivars grown in Brazil.[Citation6,Citation9]
Table 4. Pasting properties of flour and starch from four banana cultivars
Pasting temperature of banana flour and starch ranged from 78.66°C to 81.47°C and 76.58°C to 80.70°C, respectively. Kepok cultivar in the form of both starch and flour had the highest pasting temperature compared to the other cultivars. Pasting point has been reported to be affected by crystallinity.[Citation26] In this study, particularly in starch form, Kepok which had a similar pasting point to Kapas starch exhibited similar crystallinity. Similar trend was also observed in Ambon and Nangka starch.
Peak viscosity of banana starches ranged from 3913.25 to 5182.5 cP. Ambon and Nangka starches had the highest peak viscosity among banana starch samples, indicating Ambon and Nangka starches had higher ability to form a paste on cooking. Peak viscosity also described the extent of granule swelling. A study has reported the role of swelling volume on peak viscosity.[Citation26] Comparing the data on swelling volumes, there was no significant difference (p > .05) in the swelling volume of starches from different cultivars suggesting that there was no significant role of swelling volume on the peak viscosity.
Furthermore, Kapas in both starch and flour forms had the lowest breakdown but the highest setback viscosity amongst the samples studied. Breakdown viscosity reflected the stability of starch during heating and stirring. It suggested that the most stable starch to the heating process was from Kapas cultivar. The trend in the increase of setback viscosity in the starch form was correlated to the trend in the increase of solubility which reflected an increase in the amylose leaching. It is thus likely that amylose amount dissolved in water affected the viscosity.
Effect of non-starch components on pasting properties: The presence of non-starch components affected the pasting properties of banana starch. In the Kapas cultivar, the pasting temperature was higher in the flour form than in the starch form. This might be attributed to the presence of non-starch components serving as a heat barrier hence having less effective heat transfer into the starch granule. Furthermore, it could be due to the interaction between starch and non-starch components. Starch surrounded by protein matrix in the form of aggregates might have less access to water, resulting in higher pasting temperature. Dietary fiber might also interact with starch and water, altering pasting properties of banana flour.[Citation15] Li and Zhu[Citation15] have reported that lipid-amylose complexes increased gelatinization temperature and granule integrity. The lipid-amylose complex has been reported to increase following thermal treatment.[Citation26]
Ambon and Nangka cultivars exhibited higher peak viscosity while Kepok had lower peak viscosity in the presence of non-starch components. Sasaki et.al.[Citation39] has reported that interaction of starch with non-starch polysaccharide (pectin, xanthan gum, guar gum, konjac-glucomannan) increased peak viscosity. Banana flour has been reported to have a high pectin content of 3.3–5.6% db.[Citation35] Therefore, in this study, the higher peak viscosity in banana flour could be due to the pectin content. Meanwhile, all cultivars displayed higher breakdown viscosity in the presence of non-starch components suggesting that the components reduced the stability of starch to heating and shearing process.
Banana starch had higher setback viscosity (1212–2258 cP) than banana flour (1160–1424 cP), which was in line with a previous study.[Citation29] Li and Zhu[Citation15] have reported that lipid-amylose complexes increased gelatinization temperature and granule integrity while decreasing retrogradation. They suggested that non-starch components such as protein, lipids, dietary fiber and polyphenols could interact with starch during pasting. Furthermore, Fu et al. [Citation40] explained that amylose-lipid complexes could disturb the mobility of amylose molecule and reduced amylose dissolution in water hence reducing the setback viscosity.
Conclusion
Cooking banana cultivars of Kapas, Kepok and Nangka had a B-type crystalline pattern, while the dessert banana type (Ambon) exhibited a crystalline structure similar to C-type. Nangka and Ambon starches possessed similar crystallinity (33–34%) while the crystallinity of Kapas starch (38.6%) was closer to that of Kepok starch (39.4%). The banana flours exhibited higher crystallinity than the starches suggesting a contribution of non-starch content to the crystallinity. The granules of all cultivars varied in shape but elongated shape was mainly found in the granules of Kapas while spheroidal shape was predominantly present in the granules of rest of the cultivars. The granule size ranged from 5.5 to 59 μm. Cultivars did not significantly affect the swelling volume and solubility (except in Ambon cultivar) (p > .05) but determined water absorption capacity (WAC) and particularly freeze-thaw stability (FTS). The presence of non-starch components increased the functional properties. Amongst the functional properties studied, syneresis was remarkably affected by the difference in the cultivars amongst which Ambon cultivar had the lowest syneresis. The low syneresis reflected a stability to retrogradation which may be related to the lower amylose content. Therefore, in food application, Ambon may be the suitable starch as an ingredient in food expected to stable in low-temperature storage. Color and texture characteristics, particularly gumminess and hardness were determined by the cultivars. The presence of non-starch components decreased the texture characteristics. The gumminess values were dictated by the value of hardness but not by the cohesiveness. The hardness values may be affected by the crystallinity. Cultivar clearly played a role in pasting properties of starch. Kepok was the most resistant to gelatinization indicated by the highest pasting temperature reaching approximately 81°C. The non-starch components increased pasting point and breakdown viscosity but decreased setback viscosity.
Additional information
Funding
References
- FAO. Food and Agriculture Organization of the United Nations. Faostat. 2017. Retrieved from: http://www.fao.org/faostat/en/#rankings/countries_by_commodity (accessed May,9th 2019).
- Indonesia, B.-S. Statistics of Annual Fruit and Vegetable Plants; B.-S., Ed; BPS-Statistics Indonesia: Indonesia, Jakarta, 2017.
- Zhang, P.; Whistler, R. L.; BeMiller, J. N.; Hamaker, B. R. Banana Starch: Production, Physicochemical Properties, and Digestibility—a Review. Carbohydr. Polym. 2005, 59(4), 443–458. DOI: 10.1016/j.carbpol.2004.10.014.
- Nimsung, P.; Thongngam, M.; Naivikul, O. Compositions, Morphological and Thermal Properties of Green Banana Flour and Starch. Kasetsart J. (Nat. Sci.). 2007, 41, 324–330.
- Englyst, H. N.; Kingman, S. M.; Cummings, J. Classification and Measurement of Nutritionally Important Starch Fractions. Eur. J. Clin. Nutr. 1992, 46(Suppl 2), S33–50.
- de Barros Mesquita, C.; Leonel, M.; Franco, C. M. L.; Leonel, S.; Garcia, E. L.; Dos Santos, T. P. R. Characterization of Banana Starches Obtained from Cultivars Grown in Brazil. Int. J. Biol. Macromol. 2016, 89(Supplement C), 632–639. DOI: 10.1016/j.ijbiomac.2016.05.040.
- Coulibaly, S.; Nemlin, J. G.; Amani, G. N. G. Isolation and Partial Characterisation of Native Starches of New Banana and Plantain Hybrids (musa Spp.) In Comparison with that of Plantain Variety Orishele. Starch - Stärke. 2006, 58(7), 360–370. DOI: 10.1002/star.200500476.
- Vatanasuchart, N.; Niyomwit, B.; Wongkrajang, K. Resistant Starch Content, in Vitro Starch Digestibility and Physico-Chemical Properties of Flour and Starch from Thai Bananas. Maejo Int. J. Sci. Technol. 2012, 6(2), 259–271. DOI: 10.14456/mijst.2012.19.
- Kongolo, J.; Suzanne Da Silva, L.; Wokadala, O.; Du Plessis, B.; Husselman, J.; Ngcobo, M. E. K.; Emmambux, M.; Daneel, M. Pasting, Thermal, Gel Texture, Resistant Starch and Colour Properties of Unripe Banana Flour from 10 Desert Banana Varieties Cultivated in South Africa. J. Food Meas. Charact. 2017, 11. DOI: 10.1007/s11694-017-9481-x.
- Hung, P. V.; Cham, N. T. M.; Truc, P. T. T. Characterization of Vietnamese Banana Starch and Its Resistant Starch Improvement. Int. Food Res J. 2013, 20(1), 205–211. DOI: 10.1002/(SICI)1521-379X(200004)52:2/3<68::AID-STAR68>3.0.CO;2-1.
- Bello-Perez, L. A.; Agama-Acevedo, E.; Sayago-Ayerdi, S. G.; Moreno-Damian, E.; Figueroa, J. D. C. Some Structural, Physicochemical and Functional Studies of Banana Starches from Two Varieties Growing in Guerrero, Mexico. Starch - Stärke. 2000, 52, 68–73. DOI: 10.1002/(SICI)1521-379X(200004)52:2/3<68::AID-STAR68>3.0.CO;2-1.
- Hoffmann Sardá, F. A.; de Lima, F. N. R.; Lopes, N. T. T.; Santos, A. D. O.; Tobaruela, E. D. C.; Kato, E. T. M.; Menezes, E. W. Identification of Carbohydrate Parameters in Commercial Unripe Banana Flour. Food Res. Int. 2016, 81(Supplement C), 203–209. DOI: 10.1016/j.foodres.2015.11.016.
- Da Mota, R. V.; Lajolo, F. M.; Cordenunsi, B. R.; Ciacco, C. Composition and Functional Properties of Banana Flour from Different Varieties. Starch - Stärke. 2000, 52(2‐3), 63–68. DOI: 10.1002/(SICI)1521-379X(200004)52:2/3<63::AID-STAR63>3.0.CO;2-V.
- Wang, J.; Huang, H. H.; Chen, P. S. Structural and Physicochemical Properties of Banana Resistant Starch from Four Cultivars. Int. J. Food Prop. 2017, 20(6), 1338–1347. DOI: 10.1080/10942912.2016.1209517.
- Li, G.; Zhu, F. Physicochemical Properties of Quinoa Flour as Affected by Starch Interactions. Food Chem. 2017, 221(Supplement C), 1560–1568. DOI: 10.1016/j.foodchem.2016.10.137.
- Sasaki, T.; Kohyama, K. Influence of Non-Starch Polysaccharides on the in Vitro Digestibility and Viscosity of Starch Suspensions. Food Chem. 2012, 133(4), 1420–1426. DOI: 10.1016/j.foodchem.2012.02.029.
- Chávez-Salazar, A.; Bello-Pérez, L. A.; Agama-Acevedo, E.; Castellanos-Galeano, F. J.; Álvarez-Barreto, C. I.; Pacheco-Vargas, G. Isolation and Partial Characterization of Starch from Banana Cultivars Grown in Colombia. Int. J. Biol. Macromol. 2017, 98(Supplement C), 240–246. DOI: 10.1016/j.ijbiomac.2017.01.024.
- Yangılar, F.;. Effects of Green Banana Flour on the Physical, Chemical and Sensory Properties of Ice Cream. Food Technol. Biotechnol. 2015, 53(3), 315–323. DOI: 10.17113/ftb.53.03.15.3851.
- AOAC. Official Method of Analysis; The Association of Official Analytical Chemistry: Washington DC, 1995.
- AOAC. Official Method of Analysis; The Association of Official Analytical Chemistry: Washington DC, 1970.
- Graham, R. A Proposal for Irri to Establish A Grain Quality and Nutrition Research Center. IRRI Discussion Paper Series No.44 2002, 15 p.
- Cahyana, Y.; Titipanillah, R.; Mardawati, E.; Sukarminah, E.; Rialita, T.; Andoyo, R.; Djali, M.; Hanidah, -I.-I.; Setiasih, I. S.; Handarini, K. Non-Starch Contents Affect the Susceptibility of Banana Starch and Flour to Ozonation. J. Food Sci. Technol. 2018, 55(5), 1726–1733. DOI: 10.1007/s13197-018-3085-2.
- Marta, H.; Cahyana, Y.; Arifin, H. R.; Khairani, L. Comparing the Effect of Four Different Thermal Modifications on Physicochemical and Pasting Properties of Breadfruit (artocarpus Altilis) Starch. Int. Food Res J. 2019, 26(1), 269–276.
- Wattanachant, S.; Muhammad, K.; Mat Hashim, D.; Rahman, R. A. Effect of Crosslinking Reagents and Hydroxypropylation Levels on Dual-Modified Sago Starch Properties. Food Chem. 2003, 80(4), 463–471. DOI: 10.1016/S0308-8146(02)00314-X.
- Yang, Z.; Wang, W.; Wang, H.; Ye, Q. Effects of a Highly Resistant Rice Starch and Pre-Incubation Temperatures on the Physicochemical Properties of Surimi Gel from Grass Carp (ctenopharyn Odon Idellus). Food Chem. 2014, 145, 212–219. DOI: 10.1016/j.foodchem.2013.08.040.
- Cahyana, Y.; Wijaya, E.; Halimah, T. S.; Marta, H.; Suryadi, E.; Kurniati, D. The Effect of Different Thermal Modifications on Slowly Digestible Starch and Physicochemical Properties of Green Banana Flour (musa Acuminata Colla). Food Chem. 2019, 274, 274–280. DOI: 10.1016/j.foodchem.2018.09.004.
- Utrilla, R.; E Rodríguez-Huezo, M.; Carrillo-Navas, H.; Hernández-Jaimes, C.; Vernon-Carter, E. J.; Alvarez-Ramirez, J. In Vitro Digestibility, Physicochemical, Thermal and Rheological Properties of Banana Starches, Carbohydr.Polym. Vol. 101, 2014, 154–162.
- Faisant, N.; Buleon, A.; Colonna, P.; Molis, C.; Lartigue, S.; Galmiche, J. P.; Champ, M. Digestion of Raw Banana Starch in the Small Intestine of Healthy Humans: Structural Features of Resistant Starch. Br. J. Nutr. 1995, 73(1), 111–123. DOI: 10.1079/BJN19950013.
- Pelissari, F. M.; Andrade-Mahecha, M. M.; Sobral, P. J. D. A.; Menegalli, F. C. Isolation and Characterization of the Flour and Starch of Plantain Bananas (musa Paradisiaca). Starch - Stärke. 2012, 64(5), 382–391. DOI: 10.1002/star.201100133.
- Waliszewski, K. N.; Aparicio, M. A.; Bello, L. S. A.; Monroy, J. A. Changes of Banana Starch by Chemical and Physical Modification. Carbohydr. Polym. 2003, 52(3), 237–242. DOI: 10.1016/S0144-8617(02)00270-9.
- Bello-Pérez, L. A.; Romero-Manilla, R.; Paredes-López, O. Preparation and Properties of Physically Modified Banana Starch Prepared by Alcoholic-Alkaline Treatment. Starch - Stärke. 2000, 52(5), 154–159. DOI: 10.1002/1521-379X(200006)52:5<154::AID-STAR154>3.0.CO;2-#.
- Ratnayake, W. S.; Hoover, R.; Warkentin, T., Pea Starch: Composition, Structure and Properties — a Review. Starch - Stärke 2002, 54(6), 217–234. DOI:10.1002/1521-379X(200206)54:6< 217::AID-STAR217>3.0.CO;2-R.
- Wang, S.; Li, C.; Copeland, L.; Niu, Q.; Wang, S., Starch Retrogradation: A Comprehensive Review. Comprehensive Reviews in Food Science and Food Safety 2015, 14(5), 568–585. DOI: 10.1111/1541-4337.12143.
- Parimalavalli, R.; Babu, D., Comparative Study on Properties of Banana Flour, Starch and Autoclaved Starch. Trends Carbohydr Res. 2014, 6(1), 38–44.
- Bi, Y.; Zhang, Y.; Jiang, H.; Hong, Y.; Gu, Z.; Cheng, L.; Li, Z.; Li, C., Molecular Structure and Digestibility of Banana Flour and Starch. Food Hydrocoll. 2017, 72 (Supplement C), 219-227. DOI: 10.1016/j.foodhyd.2017.06.003.
- Tufvesson, F.; Wahlgren, M.; Eliasson, A.-C., Formation of Amylose-Lipid Complexes and Effects of Temperature Treatment. Part 2. Fatty Acids. Starch - Stärke 2003, 55 (3‐4), 138–149. DOI: 10.1002/star.200390028.
- Szczesniak, A. S., Texture Is a Sensory Property. Food Qual Prefer. 2002, 13(4), 215–225. DOI: 10.1016/S0950-3293(01)00039-8.
- Fatemeh, S. R.; Ramli, S.; Alkarkhi, A.; Easa, A., Total Phenolics, Flavonoids and Antioxidant Activity of Banana Pulp and Peel Flours: Influence of Variety and Stage of Ripeness. Int. Food Res J. 2012, 19(3), 1041–1046.
- Sasaki, T.; Sotome, I.; Okadome, H., In Vitro Starch Digestibility and in Vivo Glucose Response of Gelatinized Potato Starch in the Presence of Non-Starch Polysaccharides. Starch - Stärke 2015, 67 (5–6), 415–423. DOI: 10.1002/star.201400214.
- Fu, Z.; Chen, J.; Luo, S.-J.; Liu, C.-M.; Liu, W., Effect of Food Additives on Starch Retrogradation: A Review. Starch - Stärke 2015, 67 (1–2), 69–78. DOI: 10.1002/star.201300278.