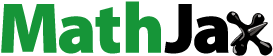
ABSTRACT
In this study, we investigated the effect of microwave-assisted extraction (MAE) on the yield, structure, and properties of rabbit skin gelatin. Compared to water bath extraction gelatin, short-term (5–30 min) MAE gelatin had better gel properties, which formed gels at a slower rate due to destruction of more hydrogen bonds, but had more powerful triple helix-like structures after a sufficiently long maturity (16–18 h) since more high-molecular-weight subunits were retained. Scanning electron microscope showed that MAE could form numerous holes on the surface of gelatin. This study will be of great significance for high efficiency of gelatin extraction.
KEYWORDS:
Introduction
Gelatin is a kind of biopolymer obtained by partial hydrolysis of animal collagen derived from skins and bones, which is generally regarded as wastes during animal slaughtering and processing.[Citation1] The principal structure of collagen is a rod-shape triple helix composed of three polypeptide chains. The main chains are more than 1,000 residues and the molecular weights are more than 100 kDa.[Citation2] By virtue of attractive advantages such as biocompatibility, hydrophilicity, nontoxicity, gelation, and low cost,[Citation3] gelatin is widely used in food,[Citation4] pharmaceutical,[Citation5] and photographic industries[Citation6] as a gelling agent, stabilizer, thickener, and emulsifier.[Citation7,Citation8] However, the production of gelatin is hampered by the issue of high pollution and long extraction time. Muralidharan et al.[Citation9] extracted gelatin from squid skin using a temperature-controlled water bath for 12 h. Badii and Howell[Citation10] extracted gelatin from horse mackerel skin at 45°C overnight. Our laboratory extracted gelatin from rabbit skin with distilled water at 60°C for 6 h.[Citation11] Hence, effective reduction of the extraction time is of great significance during gelatin production.
Microwaves are electromagnetic waves with a frequency in the range of 300 MHz–300 GHz.[Citation12] Microwave heating is caused by the ability of materials to absorb microwave energy and convert it into heat. Due to the dipolar nature of water, in the case of high-frequency electric field, the permanently polarized dipolar molecules realign in the direction of the electric field a million times per second, causing internal friction of the molecules, thus generating volumetric heat.[Citation13] Therefore, microwave can be applied to heat materials instantaneously. At the present stage, microwave has been reported to extract natural products such as polysaccharides,[Citation14] phenolic compounds,[Citation15] and green coffee oil.[Citation16] Up to now, only a few researches focused on gelatin extraction using microwave. Park et al.[Citation17] investigated the effects of three extraction methods on quality characteristics of duck feet gelatin, including water bath, pressure cooker, and microwave oven extraction. The results indicated that microwave oven was conducive to obtain good properties including the highest gel strength, viscosity, and melting point. Binsi et al.[Citation18] evaluated the gelation and thermal characteristics of microwave-extracted fish scale gelatin blended with natural gums, but specific conditions for MAE were not mentioned in this article. So there is still lack of data on the mechanism of MAE of gelatin.
Currently, the main sources of gelatins are pig skins, bovine hides, and cattle bones.[Citation19] However, due to religious beliefs[Citation10] and some zoonosis, such as foot-and-mouth disease,[Citation20] the application of gelatin from these sources is limited. To overcome this weakness, fish gelatin appears on the market as a substitute,[Citation21,Citation22] but its inferior rheological properties restrict its industrial applications.[Citation23] Hence, it is of great significance to develop better raw materials for gelatin extraction. China is the biggest rabbit meat producer globally.[Citation24] During meat processing, rabbit skin is generally discarded as a low-value by-product. Actually, it possesses an amino acid composition similar to pig and cattle skins and can be processed without BSE threat and religious restriction,[Citation11] demonstrating a great potential as a promising raw material for gelatin production.
In order to utilize by-products, rabbit skins were used to extract gelatin in this study. To investigate the mechanism of MAE, we used MAE method to obtain gelatin with different extraction time and further compared with water bath extraction (WBE) by some indicators, including yield, gel strength, amino acid composition, molecular weight distribution, rheological properties, Fourier transform infrared spectroscopy (FTIR), and scanning electron microscope (SEM).
Materials and methods
Materials and reagents
Fresh Hyla rabbit skins were generously provided by A Xing Ji Industry Co., Ltd. (Chongqing, China). L-Hydroxyproline was purchased from Hefei Bomei Biotechnology Co., Ltd (Hefei, China). β-Mercaptoethanol (2-ME), Tris, ammonium persulfate, N,N,N′,N′-tetramethylethylenediamine, and Coomassie Brilliant Blue R-250 were supplied by Bio-Rad Laboratories (Hercules, CA, USA). Glycine and bromophenol blue (BPB) were obtained from Sangon Biotech Co., Ltd. (Shanghai, China), and acrylamide 30% (w/v) were purchased from Solaibao Technology Co., Ltd. (Beijing, China). PageRuler™ Unstained Protein Ladder (10–200 kDa) was obtained from the Thermo Scientific. All reagents used in this study were of analytical grade.
Rabbit skin preparation
After removing fat layers and noncollagenous proteins,[Citation25] the rabbit skins were washed thoroughly with distilled water and then stored at −20°C for further use. The rabbit skin contained 76.75% moisture, 14.56% protein, 5.84% fat, and 1.35% ash according to our published reports.[Citation11]
Gelatin extraction
The rabbit skins were pretreated by soaking in 1% (w/v) hydrochloric acid solution at a solid–liquid ratio of 1:6 (w/v) under stirring for 10 min at room temperature. Immediately after being washed with distilled water until pH reached to 4.0, rabbit skins were mixed with distilled water at a solid–liquid ratio of 1:3 (w/v), and then placed in a microwave extractor (SINEO MAS-II plus, Shanghai, China) at 65°C, 500 W, for gelatin extraction. To investigate the effect of microwave time, the extraction processes were carried out with different microwave times of 5, 15, 30, 60, and 90 min, respectively. After being filtered and dried at 60°C hot air atmosphere, the final products were now available as rabbit skin gelatin and named according to their corresponding microwave time (MAE-5, MAE-15, MAE-30, MAE-60, and MAE-90, respectively). Furthermore, a conventional WBE was also processed at conditions of solid–liquid ratio of 1:3 (w/v), 65°C, and 6 h for comparison study. The yield of gelatin was calculated as follows:
Gel strength
Gel strength was determined three times according to the previously reported method.[Citation26] A gelatin solution (6.67% w/v) was prepared by heating dry gelatin dispersed in distilled water at 60°C. The gelatin solution was poured into flat-bottom glass measuring bottles (54 mm in diameter, 60 mm in height) and then cooled in a water bath at 10 ± 0.1°C for 16–18 h. The gel strength was measured using a TA. XT2i Texture Analyzer (Stable Micro Systems, Godalming, Surrey, UK) featuring a 5-kN load cell and equipped with a probe measuring 1.27 cm in diameter. The gel strength, or maximum force (in grams), was obtained when the probe had penetrated 4 mm into the gelatin gels at a rate of 1 mm/s. The data represented the averages (SD) of three measurement trials.
Amino acid analysis
Gelatin samples were hydrolyzed with 6 M hydrochloric acid at 110°C for 24 h in the hydrolysis tubes. Subsequently, the hydrolyzates were filtered and diluted with distilled water before taking 1 mL of hydrolyzates to evaporate and then mixed with 1 mL of citrate buffer solution. After filtration through 0.22-μm syringe filters, the final hydrolysis solutions were analyzed by an automatic amino acid analyzer (Hitachi L-8900, Tokyo, Japan).
Molecular weight distribution
The molecular weight distribution of gelatins was investigated by sodium dodecyl sulfate–polyacrylamide gel electrophoresis (SDS-PAGE) according to our previous method.[Citation26] The concentration of the stacking gel and the resolving gel were 5% and 6%, respectively. The protein pattern was visualized by staining with Coomassie Brilliant Blue R-250. The molecular weight was estimated from resolving gels by comparison with a protein calibration mixture (50–200 kDa).
Rheological properties
Rheological properties of gelatin solution (6.67% w/v) were evaluated using a DHR-1 rheometer (TA Instruments, Surrey, UK). A 40-mm parallel steel plate geometry with a 1,000 μm gap was used. The elastic modulus (G’) and loss modulus (G″) were recorded over a temperature sweep in the range of 5–40°C at a rate of 1°C/min. The oscillation frequency was 1 Hz, and the oscillation strain was 5%. The gelling temperature and melting temperature were expressed by the temperature corresponding to the cross-over point of G’ and G″ in the process of cooling and heating.
FTIR analysis
FTIR spectra of samples were measured and recorded using Spectrum 100 infrared spectrophotometer (Perkin-Elmer, Boston, MA, USA). Prior to measurement, 1 mg of gelatin powder was mixed with 400 mg of dry potassium bromide (KBr) powder and then pressed as discs for FTIR spectrometer analysis in the range of 4,000–400 cm–1 at a data acquisition rate of 4 cm–1.[Citation27]
Surface morphology
A scanning electron microscopy (Phenom Pro, Phenom World, Holland) was used to observe the surface morphology. The prepared gelatin gels were cut into sheets with uniform size of 4 mm × 4 mm, and then rapidly frozen using liquid nitrogen followed by vacuum freeze-drying to remove moisture. Prior to the test, the surface of the samples was coated with a thin film of gold on the magnetron sputtering system (KEJING GSL-1100X-SPC-16M, Shenyang, China).[Citation28]
Statistical analysis
The statistical analysis was performed using SPSS 17.0 for Windows (SPSS Inc., Chicago, IL, USA). Significant differences between means (α = 0.05) were obtained through Duncan’s multiple range test and one-way ANOVA.
Results and discussion
Yield of rabbit skin gelatin
The yield of rabbit skin gelatin obtained by WBE and MAE is shown in . As could be clearly observed from the figure, the yield of gelatin extracted by MAE increased with the increase in microwave extraction time, showing a positive effect of microwave. The yield increased obviously (p < .05) with the prolongation of microwave time from 5 to 60 min until a yield of 6.44% for MAE-60, then increased slightly with a further increase in microwave extraction time (60–90 min) (p > .05). However, in the experimental range of microwave extraction time, the yield of gelatin extracted by MAE was relatively lower than that of WBE (7.50%) (p < .05). In the published articles, the yield of gelatin extracted by microwave is relatively low, and the values are 0.75%[Citation17]and 1.27%,[Citation29] respectively. Generally, a longer extraction time was beneficial for obtaining a higher yield because more collagen was hydrolyzed and converted into gelatin, which could be revealed by the results of . However, more extraction time (6 h) during the process of WBE would hamper its further development and application.
Gel strength
The gel strength of rabbit skin gelatin obtained by WBE and MAE is presented in . All gelatins obtained by the two methods were higher than bovine gelatin (259 g) and porcine skin gelatin (295 g),[Citation11] implying a good gel strength for rabbit skin gelatin. The gelatins obtained by MAE-5, MAE-15 and MAE-30 showed significantly higher values than that of WBE (P<0.05), indicating a short MAE time could obtain a better gel strength . A similar phenomenon was also found in a previously published study that gelatin extracted by microwave oven had the highest gel strength.[Citation17] It might be ascribed that a short microwave extraction time could release subunit components properly to achieve higher gel strength. With the prolongation of MAE time (60–90 min), the gel strength decreased significantly (p < .05). The decrease in gelatin gel strength might be due to the reduction in imino acid contents or degradation of high-molecular-weight subunits in gelatin.[Citation30,Citation31] It would be verified from these two aspects for the phenomenon caused by prolonged microwave time. There was no significant difference between the gelatins obtained by WBE and MAE-60 (p > .05), indicating that MAE-60 could achieve an efficient gelatin extraction in terms of extraction time, yield, and gel strength.
Amino acid composition
The amino acid composition of rabbit skin gelatin obtained by WBE and MAE was investigated and compared, and the results are summarized in . Glycine (Gly), proline (Pro), and hydroxyproline (Hyp) were the major constituent amino acids of obtained gelatins. The Gly content was the highest, reaching a high amount in the range of 230.45–235.94 g/1,000 g. However, there existed almost no difference among gelatins obtained by WBE and MAE in amino acid composition. In general, Gly-X-Y sequence could be found in collagen molecule, where X was usually Pro and Y was usually Hyp.[Citation1] Imino acids in gelatin were composed of Pro and Hyp, whose total amount reached 204.08–213.48 g/1,000 g in the obtained gelatins. The stability of the triple helical structure in renatured gelatins had been reported to be positively proportional to the total content of imino acids.[Citation9] In this study, with the increase in MAE time, the content of imino acids did not change obviously even contrasted to WBE, which indicated that the imino acid contents remained relatively stable regardless of the changes of microwave extraction time or extraction method. Therefore, the difference in gel strength in this study was not related to the content of imino acids.
Table 1. Amino acid composition of rabbit skin gelatin extracted by WBE and MAE. WBE: gelatin obtained by water bath extraction; MAE-5, MAE-15, MAE-30, MAE-60, and MAE-90: gelatin obtained by microwave-assisted extraction with extraction time of 5, 15, 30, 60, and 90 min, respectively
Molecular weight distribution
SDS-PAGE could be applied to explore the molecular weight distribution of gelatin, and the results are shown in . The high-molecular-weight subunits of gelatin (α, β, γ) were closely related to gel strength because the aggregation of subunit molecules and formation of triple helix-like structures were the key factors to affect gel properties during gelation process.[Citation32]
Figure 3. SDS-PAGE patterns of rabbit skin gelatin extracted by WBE and MAE. M: marker; 5, 15, 30, 60, and 90: gelatin obtained by microwave-assisted extraction with an extraction time of 5, 15, 30, 60, and 90 min, respectively. WBE: gelatin obtained by water bath extraction
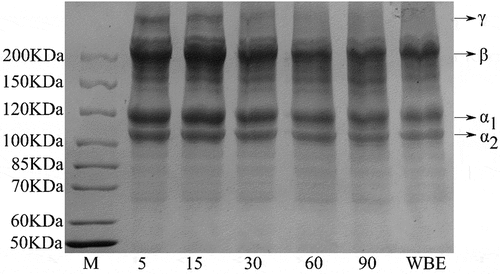
The γ components could only be seen in MAE-5, MAE-15, and MAE-30 gelatins and disappeared with the extension of MAE time. Similarly, there was no trace of γ components in WBE gelatin. All obtained gelatins showed α chains and β components. During the extraction of microwave, these two bands gradually became shallower except for MAE-5 to MAE-15, where β components appeared deeper. Moreover, the situation of gelatin obtained by WBE was very similar to that of MAE-60.
The heating of materials by microwave was mainly due to the dipolar nature of water. When an oscillating electric field was incident on the water molecules, the permanently polarized dipolar molecules rearranged in the direction of the electric field and occurred one million times per second, which caused internal friction of molecules, resulting in volumetric heating.[Citation13] Such a heating mechanism tended to extract more complex molecules together. During the MAE process, instantaneous cleavage of covalent bonds might generate the dissolution of collagen due to vibrational force of water molecules coupled with heat energy supplemented during extraction.[Citation18] Therefore, the polymer subunits of collagen could be released quickly, but long-term MAE led to excessive degradation of polymer subunits. Thus, high-molecular-weight subunits of gelatins obtained by MAE could be rapidly dissolved but also degraded with the extension of MAE time. Gelatins obtained by MAE-5 to MAE-30 showed a large amount of high-molecular-weight subunits, suggesting the polymer subunits reached an optimal release during this period, corresponding to the highest gel strength of these periods. With the degradation of high-molecular-weight subunits (MAE-60 to MAE-90), gel strength became weaker. The highest β component accumulation occurred at MAE-15. However, excessive β component content would lead to a decrease in the proportion of α-chain content. It was reported that the ratio of α-chain components (α1 and α2) was positively correlated with gel strength[Citation33]; thus the gel strength of MAE-15 exhibited a slight decrease. As the degradation of high-molecular-weight subunits of WBE gelatin was similar to MAE-60, the gel strength results of both showed no significant difference.
Rheological properties
The development of the G′ and G″ during gel formation of the obtained gelatin was depicted by cooling and heating curves in . Generally, the elastic modulus represents the gel state of the material and the loss modulus represents the liquid state of the material.[Citation34] The larger values of G’ mean greater energy stored in the material and stronger network structure. The larger G″ demonstrates more energy dissipated by the flow.[Citation35] As shown in , during cooling process, the values of G’ and G″ increased sharply, showing gelation process was underway, which was a transition of the single strands to triple helix of gelatin chains.[Citation36] On the contrary, the values of G’ and G″ decreased during the heating process, and the system changed from solid to liquid. It has been reported that the values of G’ corresponded positively to the concentration of triple helical structures.[Citation34,Citation37] With the increase in MAE time, the G’ values of gelatin showed a slight downtrend, and G’ values of WBE gelatin were higher than all gelatins obtained by MAE. The gelling/melting temperature corresponded to the intersection of the G’ and G″ curves during the cooling and heating process[Citation38] and is shown in . High gelling temperature indicates that the gel network is easier to form accompanied by an easy transition from single chains to triple helix-like structures. High melting temperature indicates stronger interactions in the systems and higher thermal stability. As shown in , WBE gelatin had the highest gelling temperature (24.30°C) and melting temperature (32.60°C). With the increase in MAE time from 5 to 30 min, there was no obvious difference in gelling/melting temperature for these obtained gelatins. However, a slight decrease in gelling/melting temperature occurred with further increase in MAE time (30–90 min), which could be related to the degradation of polymer subunits.
Figure 4. Rheological properties of rabbit skin gelatin: (a) elastic modulus (G’) and loss modulus (G″) and (b) gelling and melting temperatures. The temperature was cooling from 5°C to 40°C and heating from 40°C to 5°C. WBE: gelatin obtained by water bath extraction; MAE-5, MAE-15, MAE-30, MAE-60, and MAE-90: gelatin obtained by microwave-assisted extraction with an extraction time of 5, 15, 30, 60, and 90 min, respectively
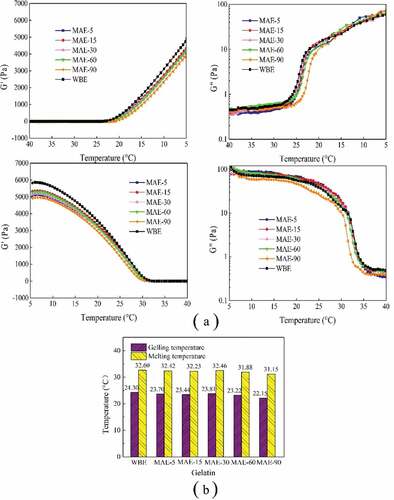
The rheology results indicated WBE gelatin had the highest G’ values, which was inconsistent with the results of gel strength. It was speculated that gelatin obtained by WBE could be transformed into triple helix-like structures more easily within a short time. Gel strength was determined after samples had undergone a longer maturation process (16–18 h), so the gelatin gel had sufficient time to form triple helix-like structures; the inconsistent behavior of rheological properties and gel strength demonstrated that gelatin obtained by short-term MAE (5–30 min) could form better triple helix-like structures but gel slower than WBE.
FTIR spectra analysis
FTIR spectra of rabbit skin gelatin obtained by WBE and MAE are shown in and the amide band positions are summarized in . Amide-A represents the N–H stretching coupled with hydrogen bonding. Generally, a free N–H stretching vibration is found in the range of 3,400–3,440 cm−1.[Citation39] When the N–H group of a peptide is involved in a hydrogen bond, the position shifts to lower frequencies.[Citation40] During MAE period, gelatin obtained by MAE-5 had the highest wavenumber (3,444.41 cm−1) and MAE-15 showed the lowest (3,435.15 cm−1); since then, amide-A shifted to higher position from 3,436.24 to 3,442.98 cm−1 (MAE-30, MAE-60, MAE-90), indicating the loss of hydrogen bonds. WBE gelatin showed the lowest position (3,433.91 cm−1), indicating the most hydrogen bonds compared with MAE samples. Conventional heating relies on heat conduction and convection.[Citation41] However, microwave heating is based on volumetric heating.[Citation42] Therefore, microwave effect could damage more hydrogen bonds during heating processing. At the beginning of the 5-min MAE, the original balance of hydrogen bonds was quickly broken, and new hydrogen bonds could not be formed in a short time, so MAE-5 gelatin was the most damaged. With the increase in MAE time, a new balance of hydrogen bonds was established with an increase in the amount (from MAE-5 to MAE-15); however, the hydrogen bonds inside gelatins were broken due to long period of both internal and external heating by microwave (from MAE-30 to MAE-90), and the destruction of hydrogen bonds showed an upward trend.
Table 2. The major peaks in the amide region of rabbit skin gelatin extracted by WBE and MAE. WBE: gelatin obtained by water bath extraction; MAE-5, MAE-15, MAE-30, MAE-60, and MAE-90: gelatin obtained by microwave-assisted extraction with extraction time of 5, 15, 30, 60, and 90 min, respectively
Figure 5. FTIR results of rabbit skin gelatin extracted by WBE and MAE. WBE: gelatin obtained by water bath extraction; MAE-5, MAE-15, MAE-30, MAE-60, and MAE-90: gelatin obtained by microwave-assisted extraction with an extraction time of 5, 15, 30, 60, and 90 min, respectively
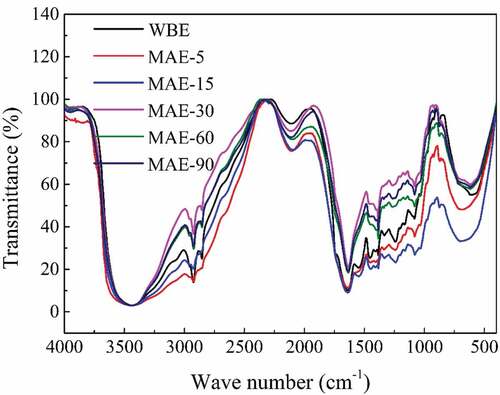
The IR absorption ratio of AIII/1454 cm−1 was a measure of the integrity of triple helical structure in dry gelatin, which was preserved when the value was bigger than or equal to 1 and damaged when the value was less than 1.[Citation43,Citation44,Citation45] As shown in , dry gelatins obtained by all extraction methods showed a comparatively high ratio from 0.77 to 0.91, indicating a relatively complete preservation of triple helical structures. In MAE group, this value showed a downward trend generally with the increase in time, illustrating thata long-term action of microwave could destroy subunit components and triple helical structures. WBE gelatin had the same value as MAE-60 (0.80), suggesting a similar preservation of triple helical structures.
Compared with MAE, the preservation of triple helical structures of gelatin obtained by WBE was not the most complete, which did not fully correspond to the rheology results that WBE gelatin gelled faster than MAE. A published study indicated that at the molecular level of native collagen structure, the hydrogen bonds relevant to water were crucial for stabilizing the triple helix structure.[Citation46] Due to the highest hydrogen bond content, WBE gelatin could form triple helix-like structures at the fastest speed in short-term gelation process. Shot-term MAE (5–30 min) gelatin had a lower content of hydrogen bonds, which was disadvantageous for the rapid formation of the triple helix-like structures, but its large amount of high-molecular-weight subunits made the gel strength higher than that of WBE gelatin.
SEM
The SEM images of the rabbit skin gelatin obtained by WBE and MAE are presented in at a magnification of 2,500 times. As clearly observed, WBE had many sheet-like structures on the surface. Unlike WBE, the surfaces of all gelatins obtained by MAE, including MAE-5, MAE-15, MAE-30, MAE-60, and MAE-90, showed numerous holes. When MAE time was short (5–30 min), the surface of gelatin remained relatively flat. However, the holes on the surface became denser with time increasing (60–90 min). In the process of WBE, the hydrolysis of intermolecular cross-links in the triple helical structure of collagen made collagen convert to gelatin.[Citation47] Microwave heating was based on volumetric heating, and the simultaneous heating of both inside and outside of materials caused more holes on the surface of gelatin obtained by MAE. Volumetric heat occurred because of the internal friction of the polarized dipole molecules,[Citation48,Citation49] thus making materials heated instantaneously. For these reasons, the surface morphology of gelatin extracted by short-term MAE (5–30 min) was not damaged so much.
Conclusion
In this study, effects of MAE on the yield, structure, and properties of rabbit skin gelatin were studied and compared with WBE. Gelatin with higher gel strength could be obtained by short-term MAE (5–30 min), but its yield was lower than WBE. With the prolongation of MAE, yield showed an increase and gel strength decreased due to the degradation of high-molecular-weight subunits.
Rheological results showed that WBE had the highest G’ under low-temperature condition and had the highest gelling and melting temperature during temperature decreasing and rising, indicating that gelatins obtained by WBE gelatin gelled faster than MAE, but MAE had more powerful triple helix-like structures after a full length of maturity (16–18 h). FTIR results showed that the short-term MAE (5–30 min) had a lower content of hydrogen bonds which could not facilitate the rapid formation of triple helix-like structures, but its gel strength was higher due to the large amount of high-molecular-weight subunits compared to WBE gelatin. SEM showed numerous holes on the surfaces of MAE gelatins; as time went on, the holes became denser. This study explored the possibility of replacing traditional water bath with microwave, which was of great significance in terms of energy conservation and environmental friendly.
Disclosure statement
The authors declare no conflict of interest.
Additional information
Funding
References
- Dille, M. J.; Hattrem, M. N.; Draget, K. I. Bioactively Filled Gelatin Gels; Challenges and Opportunities. Food Hydrocoll. 2018, 76, 17–29. DOI: 10.1016/j.foodhyd.2016.12.028.
- Fonkwe, L. G.; Narsimhan, G.; Cha, A. S. Characterization of Gelation Time and Texture of Gelatin and Gelatin–Polysaccharide Mixed Gels. Food Hydrocoll. 2003, 17, 871–883. DOI: 10.1016/S0268-005X(03)00108-5.
- Dai, H.; Ou, S.; Huang, Y.; Liu, Z.; Huang, H. Enhanced Swelling and Multiple-responsive Properties of Gelatin/sodium Alginate Hydrogels by the Addition of Carboxymethyl Cellulose Isolated from Pineapple Peel. Cellulose. 2018, 25, 593–606. DOI: 10.1007/s10570-017-1557-6.
- Mutlu, C.; Tontul, S. A.; Erbaş, M. Production of a Minimally Processed Jelly Candy for Children Using Honey Instead of Sugar. LWT. 2018, 93, 499–505. DOI: 10.1016/j.lwt.2018.03.064.
- Sahoo, N.; Sahoo, R. K.; Biswas, N.; Guha, A.; Kuotsu, K. Recent Advancement of Gelatin Nanoparticles in Drug and Vaccine Delivery. Int. J. Biol. Macromol. 2015, 81, 317–331. DOI: 10.1016/j.ijbiomac.2015.08.006.
- Bkhairia, I.; Mhamdi, S.; Jridi, M.; Nasri, M. New Acidic Proteases from Liza Aurata Viscera: Characterization and Application in Gelatin Production. Int. J. Biol. Macromol. 2016, 92, 533–542. DOI: 10.1016/j.ijbiomac.2016.07.063.
- Ali, A. M. M.; Kishimura H.; Benjakul, S. Physicochemical and Molecular Properties of Gelatin from Skin of Golden Carp (probarbus Jullieni) as Influenced by Acid Pretreatment and Prior-ultrasonication. Food Hydrocoll. 2018, 82, 164–172. DOI: 10.1016/j.foodhyd.2018.03.052.
- Erge, A.; Zorba, Ö. Optimization of Gelatin Extraction from Chicken Mechanically Deboned Meat Residue Using Alkaline Pre-treatment. LWT. 2018, 97, 205–212. DOI: 10.1016/j.lwt.2018.06.057.
- Muralidharan, N.; Soottawat, B.; Thummanoon, P.; Ponusa, S.; Hideki, K. Characteristics and Functional Properties of Gelatin from Splendid Squid (loligo Formosana) Skin as Affected by Extraction Temperatures. Food Hydrocoll. 2012, 29, 389–397. DOI: 10.1016/j.foodhyd.2012.04.001.
- Badii, F.; Howell, N. K. Fish Gelatin: Structuregelling Properties and Interaction with Egg Albumen Proteins. Food Hydrocoll. 2006, 20, 630–640. DOI: 10.1016/j.foodhyd.2005.06.006.
- Yu, W.; Wang, X.; Ma, L.; Li, H.; He, Z.; Zhang, Y. Preparationcharacterisation and Structure of Rabbit (Hyla rabbit) Skin Gelatine. Int. J. Food Sci. Technol. 2016, 51, 574–580. DOI: 10.1111/ijfs.13012.
- Asomaning, J.; Haupt, S.; Chae, M.; Bressler, D. C. Recent Developments in Microwave-assisted Thermal Conversion of Biomass for Fuels and Chemicals. Renew. Sust. Energ. Rev. 2018, 92, 642–657. DOI: 10.1016/j.rser.2018.04.084.
- Chandrasekaran, S.; Ramanathan, S.; Basak, T. Microwave Food Processing—A Review. Food Res. Int. 2013, 52, 243–261. DOI: 10.1016/j.foodres.2013.02.033.
- Hu, C.; Hu, W.; Zhao, Y.; Yang, Y.; Zhang, Z.; Zhang, H.; Ding, C.; Zhou, L.; Yuan, S.; Yuan, M.; et al. Microwave-assisted Extractionphysicochemical Characterization and Bioactivity of Polysaccharides from Camptotheca Acuminata Fruits. Int. J. Biol. Macromol. 2019, 133, 127–136.
- Cassol, L.; Rodrigues, E.; Zapata Noreña, C. P. Extracting Phenolic Compounds from Hibiscus Sabdariffa L. Calyx Using Microwave Assisted Extraction. Ind. Crops Prod. 2019, 133, 168–177. DOI: 10.1016/j.indcrop.2019.03.023.
- Tsukui, A.; Júnior, H. M. S.; Oigman, S. S.; Souza, R. O. M. A. D.; Bizzo, H. R.; Rezende, C. M. Microwave-assisted Extraction of Green Coffee Oil and Quantification of Diterpenes by Hplc. Food Chem. 2014, 164, 266–271. DOI: 10.1016/j.foodchem.2014.05.039.
- Park, J. H.; Choe, J. H.; Kim, H. W.; Hwang, K. E.; Song, D. H.; Yeo, E. J.; Kim, H. Y.; Choi, Y. S; Lee, S. H.; Kim, C. J. Effects of Various Extraction Methods on Quality Characteristics of Duck Feet Gelatin. Korean J. Food Sci. Animal Resour. 2013, 33, 162–169. DOI: 10.5851/kosfa.2013.33.2.162.
- Binsi, P. K.; Nayak, N.; Sarkar, P. C.; Joshy, C. G.; Ninan, G.; Ravishankar, C. N. Gelation and Thermal Characteristics of Microwave Extracted Fish Gelatin-natural Gum Composite Gels. J. Food Sci. Technol. 2017, 54, 518–530. DOI: 10.1007/s13197-017-2496-9.
- Lin, L.; Regenstein, J. M.; Lv, S.; Lu, J.; Jiang, S. An Overview of Gelatin Derived from Aquatic Animals: Properties and Modification. Trends Food Sci. Technol. 2017, 68, 102–112. DOI: 10.1016/j.tifs.2017.08.012.
- Jongjareonrak, A.; Benjakul, S.; Visessanguan, W.; Nagai, T.; Tanaka, M. Isolation and Characterisation of Acid and Pepsin-solubilised Collagens from the Skin of Brownstripe Red Snapper (Lutjanus vitta). Food Chem. 2005, 93, 475–484. DOI: 10.1016/j.foodchem.2004.10.026.
- Duan, R.; Zhang, J.; Liu, L.; Cui, W.; Regenstein, J. M. The Functional Properties and Application of Gelatin Derived from the Skin of Channel Catfish (Ictalurus punctatus). Food Chem. 2018, 239, 464–469. DOI: 10.1016/j.foodchem.2017.06.145.
- Joanna, T.; Małgorzata, M.; Piotr, K.; Zając, M. Characterization of Carp (Cyprinus carpio) Skin Gelatin Extracted Using Different Pretreatments Method. Food Hydrocoll. 2018, 81, 169–179. DOI: 10.1016/j.foodhyd.2018.02.048.
- Karim, A. A.; Bhat, R. Fish Gelatin: Properties, Challenges, and Prospects as an Alternative to Mammalian Gelatins. Food Hydrocolloids. 2009, 23, 563–576. DOI: 10.1016/j.foodhyd.2008.07.002.
- Li, S.; Zeng, W.; Li, R.; Hoffman, L. C.; He, Z.; Sun, Q.; Li, H. Rabbit Meat Production and Processing in Chinav.Meat Sci. 2018, 145, 320–328. DOI: 10.1016/j.meatsci.2018.06.037..
- Ma, M.; Ma, L.; Yu, W.; Zhang, X.; Shen, Y.; Zhang, Y. Research on Rapid Gelatinization of Rabbit Skin Collagen as Effect of Acid Treatment. Food Hydrocoll. 2018, 77, 945–951. DOI: 10.1016/j.foodhyd.2017.11.042.
- Chen, L.; Liang, M.; Zhou, M.; Liu, Y.; Zhang, Y. Effects of Pressure on Gelatinization of Collagen and Properties of Extracted Gelatins. Food Hydrocoll. 2014, 36, 316–322. DOI: 10.1016/j.foodhyd.2013.10.012.
- Dai, H.; Zhang, Y.; Zhang, H.; Ma, L.; Huang, H. Synthesis and Response of Pineapple Peel Carboxymethyl Cellulose-g-Poly(Acrylic Acid-Co-Acrylamide)/Graphene Oxide Hydrogels. Carbohydr. Polym. 2019, 215, 366–376. DOI: 10.1016/j.carbpol.2019.03.090.
- Dai, H.; Zhang, H.; Zhang, Y.; Ma, L.; Zhou, H.; Yu, Y.; Guo, T.; Huang, H. Green pH/magnetic Sensitive Hydrogels Based on Pineapple Peel Cellulose and Polyvinyl Alcohol: Synthesischaracterization and Naringin Prolonged Release. Carbohydr. Polym. 2019, 209, 51–61. DOI: 10.1016/j.carbpol.2019.01.014.
- Mirzapour-Kouhdasht, A.; Sabzipour, F.; Taghizadeh, M. S.; Moosavi-Nasab, M. Physicochemical, Rheological, and Molecular Characterization of Colloidal Gelatin Produced from Common Carp By-products Using Microwave and Ultrasound-assisted Extraction. J. Texture Stud. 2019, 1–10. DOI: 10.1111/jtxs.12408.
- Ahmad, M.; Benjakul, S. Characteristics of Gelatin from the Skin of Unicorn Leatherjacket (Aluterus monoceros) as Influenced by Acid Pretreatment and Extraction Time. Food Hydrocolloids. 2011, 25, 381–388. DOI: 10.1016/j.foodhyd.2010.07.004.
- Balti, R.; Jridi, M.; Sila, A.; Souissi, N.; Nedjar-Arroume, N.; Guillochon, D.; Nasri, M. Extraction and Functional Properties of Gelatin from the Skin of Cuttlefish (sepia Officinalis) Using Smooth Hound Crude Acid Protease-aided Process. Food Hydrocolloids. 2011, 25, 943–950. DOI: 10.1016/j.foodhyd.2010.09.005.
- Mackie, A. R.; Gunning, A. P.; Ridout, M. J.; Morris, V. J. Gelation of Gelatin Observation in the Bulk and at the Air-water Interface. Biopolymers. 1998, 46, :245–252. DOI: 10.1002/(ISSN)1097-0282.
- Gómez-Guillén, M. C.; Turnay, J.; Fernández-Dı́Az, M. D.; Ulmo, N.; Lizarbe, M. A.; Montero, P. Structural and Physical Properties of Gelatin Extracted from Different Marine Species: A Comparative Study. Food Hydrocolloids. 2002, 16, 25–34. DOI: 10.1016/S0268-005X(01)00035-2.
- xChiou, B. S.; Avena-Bustillos, R. J.; Shey, J.; Yee, E.; Bechtel, P. J.; Imam, S. H.; Glenn, G. M.; Orts, W. J. Rheological and Mechanical Properties of Cross-linked Fish Gelatins. Polymer. 2006, 47, 6379–6386. DOI: 10.1016/j.polymer.2006.07.004.
- Cruz, N.; Peng, Y. Rheology Measurements for Flotation Slurries with High Clay Contents – A Critical Review. Miner. Eng. 2016, 98, 137–150. DOI: 10.1016/j.mineng.2016.08.011.
- Da, S. M.; Bode, F.; Grillo, I.; Dreiss, C. A. Exploring the Kinetics of Gelation and Final Architecture of Enzymatically Cross-linked Chitosan/Gelatin Gels. Biomacromolecules. 2015, 16, 1401–1409. DOI: 10.1021/acs.biomac.5b00205.
- Simon, A.; Grohens, Y.; Vandanjon, L.; Bourseau, P.; Balnois, E.; Levesque, G. A Comparative Study of the Rheological and Structural Properties of Gelatin Gels of Mammalian and Fish Origins. Macromol. Symp. 2003, 203, 331–338. DOI: 10.1002/masy.200351337.
- Sila, A.; Martinez-Alvarez, O.; Krichen, F.; Gómez-Guillén, M. C.; Bougatef, A. Gelatin Prepared from European Eel (Anguilla anguilla) Skin: Physicochemical, Textural, Viscoelastic and Surface Properties. Colloids Surf. A Physicochem. Eng. Aspects. 2017, 529, 643–650. DOI: 10.1016/j.colsurfa.2017.06.032.
- Muyonga, J. H.; Cole, C. G. B.; Duodu, K. G. Fourier Transform Infrared (FTIR) Spectroscopic Study of Acid Soluble Collagen and Gelatin from Skins and Bones of Young and Adult Nile Perch (Lates niloticus). Food Chem. 2004, 86, 325–332. DOI: 10.1016/j.foodchem.2003.09.038.
- Doyle, B. B.; Bendit, E. G.; Blout, E. R. Infrared Spectroscopy of Collagen and Collagen-like Polypeptides. Biopolymers. 1975, 14, 937–957. DOI: 10.1002/bip.1975.360140505.
- Géczi, G.; Horváth, M.; Kaszab, T.; Alemany, G. G. No Major Differences Found between the Effects of Microwave-based and Conventional Heat Treatment Methods on Two Different Liquid Foods. PLoS One. 2013, 8, e53720. DOI: 10.1371/journal.pone.0053720.
- Guo, Q.; Sun, D. W.; Cheng, J. H.; Han, Z. Microwave Processing Techniques and Their Recent Applications in the Food Industry. Trends Food Sci. Technol. 2017, 67, 236–247. DOI: 10.1016/j.tifs.2017.07.007.
- Andrews, M. E.; Murali, J.; Muralidharan, C.; Madhulata, W.; Jayakumar, R. Interaction of Collagen with Corilagin. Colloid Polym. Sci. 2003, 281, 766–770. DOI: 10.1007/s00396-002-0843-4.
- Plepis, A. M. D. G.; Goissis, G.; Das-Gupta, D. K. Dielectric and Pyroelectric Characterization of Anionic and Native Collagen. Polym. Eng. Sci. 1996, 36, 2932–2938. DOI: 10.1002/pen.10694.
- Albu, M. G.; Ghica, M. V.; Leca, M.; Popa, L.; Borlescu, C.; Cremenescu, E.; Giurginca, M.; Trandafir, V. Doxycycline Delivery from Collagen Matrices Crosslinked with Tannic Acid. Mol. Cryst. Liq. Cryst. 2010, 523, 97–669. DOI: 10.1080/15421401003724159.
- Ramachandran, G. N.; Sasisekharan, V. Structure of Collagen. Nature. 1961, 190, 1004–1005. DOI: 10.1038/1901004a0.
- Karnjanapratum, S.; Sinthusamran, S.; Sae-leaw, T.; Benjakul, S.; Kishimura, H. Characteristics and Gel Properties of Gelatin from Skin of Asian Bullfrog (rana Tigerina). Food Biophys. 2017, 12, 289–298. DOI: 10.1007/s11483-017-9485-4.
- Shen, Q.; Zheng, C.; Zheng, Y.; Li, S.; Ding, H.; Xu, Y.; Thern, M.; Thermal, P. E.; Lund, U.; Kraftverksteknik, L. U. Optimize Process Parameters of Microwave-Assisted EDTA Method Using Orthogonal Experiment for Novel BaCoO3−δ Perovskite. J. Alloys Compd. 2016, 658, 125–131. DOI: 10.1016/j.jallcom.2015.10.194.
- Chandrasekaran, S.; Ramanathan, S.; Basak, T. Microwave Material Processing—A Review. AIChE J. 2012, 58, 330–363. DOI: 10.1002/aic.v58.2.