ABSTRACT
The total phenolic, flavonoid, coumarin, and volatile contents and antioxidant activities in three tissues of six Citrus grandis ‘Tomentosa’ (CGT) fruit varieties were investigated. Two flavones, 3 coumarins and 147 volatiles were identified. The exocarp contained 3 coumarins, however, no coumarins were identified in the mesocarp, and only isoimperatorin was found in the pulp. In the exocarp, mesocarp and pulp, 104, 79 and 71 volatiles were identified, respectively. FuMao (FM) contained the highest total phenolic, naringin and rhoifolin amounts; HouYeZhengMao (HY-ZM) contained the highest meranzin hydrate and bergapten amounts; and XiYangZhengMao (XY-ZM) contained the highest isoimperatorin amount. The ABTS radical scavenging power of HuangLongZhengMao (HL-ZM) was the strongest, and FM had the strongest DPPH radical scavenging effect and ferric ion reducing antioxidant power. According to overall performance, FM and HY-ZM are the top two varieties with the highest total phenolic, flavonoid, and coumarin contents and antioxidant capacities.
Introduction
Due to its rich flavors and nutritional benefits, the Citrus genus is now widely cultivated worldwide, and certain ancient resources have also been identified and exploited.[Citation1] C. grandis (L.) Osbeck (pomelo) is one of the three original citrus species that are usually served as fresh fruits. The sugar and juice contents of the pomelo are not high enough for processing, which hinders its further expansion in developed countries. However, in the last decade, one famous species of C. grandis (L.) Osbeck, C. grandis ‘Tomentosa’ (CGT), has received a great deal of attention for its distinguished medicinal effect of curing chronic cough.[Citation2,Citation3] CGT originated in Huazhou town in Guangdong Province of southern China and is also called Huajuhong in Chinese by locals.[Citation4] CGT received China’s GI protection and was officially recorded in the Chinese Pharmacopoeia in 2007.[Citation2] CGT has also been recognized as one of the edible and medical plant resources by the Chinese Ministry of Health.[Citation5] In recent years, the bioactivities of CGT, such as its antioxidant, anticancer, antimicrobial and anti-inflammatory properties, have also been discovered and investigated.[Citation6–Citation9]
Figure 1. The six varieties of Citrus grandis ‘Tomentosa’ fruits investigated in this study: (a): MiYeZhengMao, (b): ‘HouYeZhengMao’, (c): ‘HuangLongZhengMao’, (d): XiYangZhengMao, (e): FuMao, (f): GuangQing
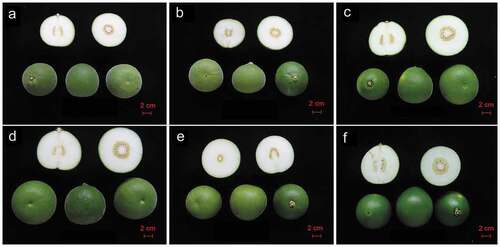
The exocarp of CGT is densely pubescent, which is a trait of ancient species.[Citation10] The dense, short and firm trichomes on the surface of the fruits and leaves can reduce the chance of being infected by Candidatus Liberibacter, which causes the citrus greening disease (Huanglongbing), a fatal disease for the citrus industry.[Citation11] CGT has been divided into six varieties according to the biological characteristics of the tree body and the density of trichomes on the fruit surface by local farmers, namely, HuangLongZhengMao (HL-ZM), HouYeZhengMao (HY-ZM), MiYeZhengMao (MY-ZM), XiYangZhengMao (XY-ZM), FuMao (FM) and GuangQing (GQ). The density of trichomes is ranked as ZhengMao>FuMao>GuangQing. It is commonly believed that the density of trichomes indicates the bioactivity of the fruit; namely, the denser its trichomes are, the higher the bioactivity of the fruit is. However, to the best of our knowledge, there is no scientific evidence to support this traditional viewpoint. In traditional medicine, CGT is primarily utilized in the form of whole fruits or separated pericarp, and those in the former form have a larger market share.[Citation2] Moreover, it has been verified that the contents of flavonoids in whole fruits are significantly higher than that in just the epicarp.[Citation2] Since the fruits of CGT are composed of the exocarp, mesocarp, and pulp, it is necessary to systematically investigate the compositions of the active ingredients in the different parts of the fruits. Meanwhile, the accumulative patterns of these bioactive secondary metabolites in CGT fruits could be revealed.
Flavonoids and volatiles are two kinds of bioactive components universally acknowledged in the fruit of CGT.[Citation3,Citation12,Citation13] Additionally, two kinds of coumarins, meranzin hydrate and isoimperatorin, were also found in CGT fruits.[Citation8] Naringin was identified as the major compound accounting for the antitussive and expectorant activities.[Citation14,Citation15] Recent studies have also noted that coumarins have multiple biological activities, such as antitumoral, anticoagulant, anti-inflammatory, antimicrobial and antioxidant activities.[Citation16–Citation19] The volatiles in CGT fruits not only exert antibacterial, anti-inflammatory and expectorant functions but also play an important role in the quality control of the crude drug.[Citation20] Xie et al. extracted 56 volatile compounds from the peels of CGT fruits by headspace solid-phase microextraction (HS-SPME) and compared the compounds with the extracts from steam distillation and solvent extraction, and the results obtained by HS-SPME showed satisfactory repeatability.[Citation21] In the present study, six varieties (HL-ZM, HY-ZM, MY-ZM, XY-ZM, FM, and GQ) of CGT fruits were collected, and each fruit was divided into three biological parts (exocarp, mesocarp, and pulp) to compare the differences among the varieties and tissues. The flavonoids and coumarins were qualitatively measured by high-performance liquid chromatography diode-array detection (HPLC-DAD). The volatiles were extracted and identified by HS-SPME coupled with gas chromatography-mass spectrometry (GC-MS). The total phenolic contents (TPCs) and antioxidant activities were assayed by colorimetric methods. The objective of this study was to systematically evaluate and compare the chemical profiles and antioxidant activities in the different tissues of current varieties of CGT.
Materials and methods
Chemicals and reagents
Folin-Ciocalteu reagent, gallic acid, 2,20-azino-bis(3-ethylbenzthiazoline-6-sulfonic acid) (ABTS), 1,1-diphenyl-2-picrylhydrazyl (DPPH), 2,4,6-tripyridyl-s-triazine (TPTZ) and Trolox were purchased from Sigma-Aldrich, Inc. (St. Louis, MO, USA). HPLC-grade naringin, rhoifolin, meranzin hydrate, bergapten, and isoimperatorin were purchased from Aladdin Reagent Co., Ltd., China. HPLC-grade acetonitrile and methanol were purchased from Thermo Fisher Scientific Inc. (Shanghai, China). All other chemicals were analytical grade and used as received.
Plant material and sample treatment
The CGT fruits were collected from a standard nursery regulated by Huazhou Huajuhong Medicinal Materials Development Co., Ltd. (with a Good Manufacturing Practice (GMP) certificate, Huazhou, Guangdong, China) in 2018. Six phenotypes of CGT were named according to the morphologies of the trichomes on the surface of the fruits. Their names were as follows: HuangLongZhengMao (HL-ZM or HL), HouYeZhengMao (HY-ZM or HY), MiYeZhengMao (MY-ZM or MY), XiYangZhengMao (XY-ZM or XY), FuMao (FM), and GuangQing (GQ), and the photos of their fruits were presented in . The fruits were picked in May according to the local harvesting and selling period. The exocarp, mesocarp and pulp of the fruits were separated carefully, lyophilized, and ground to 60 mesh. The phytochemicals were extracted according to previously published literature.[Citation8] In summary, 0.5 g of the sample was extracted in 25 mL of methanol by an ultrasonicator for 30 min at 100 Hz. After centrifugation, the supernatant was filtrated and stored at −20°C before analysis.
Determination of the TPC
The TPC was determined by the Folin-Ciocalteau colorimetric method.[Citation22] First, 10 µL of the extract was added to 990 µL of ultrapure water, and then 50 µL of Folin-Ciocalteu reagent was added to the diluted samples. Finally, the reaction was neutralized by a 30% (wt%) sodium carbonate solution. After standing at room temperature for 1 h, the absorbance at 725 nm was determined using a spectrophotometer (TECAN, Spark 10M). A standard curve of gallic acid was prepared under identical conditions with concentrations of 0, 0.2, 0.4, 0.6, 0.8, and 1.0 mg mL−1. All tests were repeated at least three times, and the mean values with standard deviation were presented.
Ultra-performance liquid chromatography diode-array detection (UPLC-DAD) analysis
UPLC-DAD analysis was performed on an Agilent 1290 series UPLC system (Agilent, USA). The samples were separated on a reversed-phase ZORBAX RRHD Eclipse Plus C18 column (2.1 mm×50 mm, 1.8 μm). The flow rate was 0.2 mL min−1, and the gradient conditions were as follows: solvent A (H2O-HCOOH, 99/1, v/v) and solvent B (CH3CN-HCOOH, 99/1, v/v); initially, 5% B; 0–0.6 min 15% B linear; 0.6–2 min 40% B linear; 2–5 min 70% B linear; 5–7 min 90% B linear; 7–9 min 5% B linear. The postrun was kept at 5% for 5 min to allow reequilibration of the column. The injection sample volume was 1 µL, and the DAD was set to monitor at 283 nm for naringin, 310 nm for isoimperatorin, 320 nm for bergapten, and 330 nm for rhoifolin and meranzin hydrate.
HS-SPME-GC-MS analysis
An amount of 0.2 g of CGT powder was weighed and transferred to a 20 mL flat bottom headspace vial, which was sealed with a headspace stopper and water bathed at 60°C for 5 min. The carboxen/divinylbenzene/polydimethylsiloxane fiber (CAR/DVB/PDMS, 50/30 μm) attached in a solid-phase microextraction (SPME) holder was used to extract the volatile components of CGT from the vial. After adsorption for 30 min, the fiber was removed from the vial, and the volatiles were desorbed by exposing the fiber in the injection port of a GC/MS at 250°C for 3 min under 1 mL/min of gas flow.
The analysis of the volatiles in CGT was performed using a GC-MS instrument (Agilent 7890B-5977A) equipped with an electron impact (EI) ion source. The volatiles were separated on an HP-5MS capillary column (30 m × 0.25 mm × 0.25 μm; Agilent, USA). The oven temperature program was set as follows: 50°C initially for 1 min, increased to 145°C at 5°C/min, increased to 175°C at 7°C/min, increased to 195°C at 5°C/min, and then ramped to 250°C at 3°C/min; 250°C was maintained for 10 min. High purity helium (99.999%) was the carrier gas set at a constant flow rate of 1.0 mL min−1. The injection port, transfer line, and ion source temperatures were all set at 250°C. An EI of 70 eV was adopted, and the mass scanning range was set from 30 to 400 amu in full scan. The injection was performed in split mode with a split ratio of 10:1. The solvent delay time was set for 1 min for all samples. An Agilent MassHunter workstation was used to process the data.
Antioxidant activity
ABTS assay
The ABTS free radical scavenging activities of the CGT extracts were assessed as described in a previous method[Citation23] with minor modifications. Briefly, a stock solution was prepared by dissolving 0.384 g and 0.134 g of ABTS and potassium peroxodisulfate into 200 ml of distilled water and then kept in the dark at room temperature for 16 h. The stock solution was diluted with 0.1 mmol L−1 PBS (pH 7.2–7.4) to absorbance values of 0.70 ± 0.02 at 734 nm. Then, 1 mL of the ABTS working solution was mixed thoroughly with 50 µl of the sample, and after 30 min of incubation at room temperature in the dark, the absorbance was recorded at 734 nm. Equivalent amounts of various concentrations of Trolox were reacted with the ABTS working solution to draw a standard curve for calculating the ABTS antioxidant activity. The data were presented as milligrams of Trolox equivalents per 100 g of dry weight (mg TE/100 g DW).
DPPH assay
The free radical scavenging activities of the CGT extracts were also determined by the modified DPPH method.[Citation24] First, 3.5 mg of DPPH was dissolved in 10 ml ethanol, and then, 2 ml of the stock solution was diluted with 98 ml ethanol to make the final DPPH working solution. Second, 0.2 ml of the sample was rapidly added to 1.8 ml of the DPPH working solution and kept in the dark at room temperature for 30 min; then, the absorbance of the mixture was measured at 517 nm. Equivalent amounts of various concentrations of Trolox were reacted with the DPPH working solution under identical conditions to generate a standard curve. The DPPH antioxidant activities were calculated and expressed as milligrams of Trolox equivalents per 100 g of dry weight (mg TE/100 g DW).
Ferric reducing antioxidant power (FRAP) assay
The FRAP assay of the CGT extracts was performed according to a previously published method[Citation25] with minor modifications. In summary, a 300 mmol L−1 acetate buffer (pH 3.6), 10 mmol L−1 TPTZ (in 40 mmol L−1 HCl) and 20 mmol L−1 FeCl3 · 6H2O were mixed in a ratio of 10:1:1 and then warmed at 37°C before utilization. Then, 50 µL of the sample was reacted with 950 µL of the TPTZ working solution at 37°C for 30 min out of light. Finally, the absorbance at 593 nm was recorded, and a Trolox standard curve was generated. The FRAP antioxidant activities were calculated and expressed as milligrams of Trolox equivalents per 100 g of dry weight (mg TEAC/100 g DW).
Statistical analysis
Data are shown as the mean ± standard deviation of the three replicates. Significant differences among the samples were calculated using one-way analysis of variance (ANOVA), followed by LSD (L) multiple-range test at the 5% level (p < .05). The Pearson correlation coefficients were calculated in a bivariate correlation fashion.[Citation26] The statistical analysis was performed using SPSS software (SPSS for Windows, Release 19.0, SPSS Inc.).
Results and discussion
Determination of the TPC
As shown in , the TPCs of the six CGT varieties in the exocarp, mesocarp and pulp varied from 14.28 ± 0.34 to 27.67 ± 0.81 mg gallic acid equivalents (GAE) g−1 DW, 25.77 ± 1.22 to 38.59 ± 0.82 mg GAE g−1 DW and 25.75 ± 1.35 to 48.36 ± 1.23 mg GAE g−1 DW, respectively. The TPCs in the exocarp, mesocarp and pulp ranked as FuMao (FM) ~ MiYeZhengMao (MY-ZM) > HouYeZhengMao (HY-ZM) ~ HuangLongZhengMao (HL-ZM) > GuangQing (GQ) > XiYangZhengMao (XY-ZM), FM > MY-ZM > XY-ZM > GQ > HL-ZM ~ HY-ZM and FM > XY-ZM > HY-ZM ~ HL-ZM > MY-ZM > GQ, respectively. The highest TPC was found in the pulp of FM, and the lowest TPC was found in the exocarp of GQ. There was a distinct difference in the phenolic contents between the different fruit tissues. On average, the pulp (42.75 mg GAE g−1 DW, ignorance of the error for all the mean values) contained the highest amounts of phenolic compounds, followed by the mesocarp (31.26 mg GAE g−1 DW) and exocarp (21.54 mg GAE g−1 DW). The highest TPCs of the whole fruits were found in the FM variety, with an average value of 38.19 ± 0.56 mg GAE g−1 DW. The different contents of total phenolic were observed in both the different varieties and the fruits parts. Nevertheless, it is difficult to compare the TPCs of CGT with that of other fruits reported previously since other factors including geographical origin, cultivation model and extraction methods might affect the contents of secondary metabolites beside the different varieties and fruit tissues.[Citation27,Citation28]
Table 1. The total phenolic content in three different fruits tissues of six Citrus grandis ‘Tomentosa’ varietiesa.
Quantification of the flavones and coumarins
In the present study, 2 flavones (naringin and rhoifolin) and 3 coumarins (meranzin hydrate, bergapten and isoimperatorin) were identified in CGT. Naringin and rhoifolin were detected in all of the samples. However, no coumarins were detected in the mesocarp tissue, and isoimperatorin was only found in the pulp.
According to , the naringin contents of the exocarp, mesocarp and pulp of the six CGT varieties varied from 12.35 ± 0.14 to 33.94 ± 0.69 mg g−1 DW, 54.51 ± 0.62 to 80.40 ± 0.75 mg g−1 DW and 157.78 ± 0.81 to 251.56 ± 1.03 mg g−1 DW, respectively. The difference in the naringin contents of the different tissues was significant, with rankings of HY-ZM > GQ > FM > MY-ZM > XY-ZM > HL-ZM, FM > MY-ZM > GQ > XY-ZM > HY-ZM > HL-ZM and XY-ZM > FM > HY-ZM > HL-ZM > GQ > MY-ZM in the exocarp, mesocarp and pulp, respectively. Similar to the contents of phenolic compounds, the pulp (192.26 mg g−1 DW) contained the largest amount of naringin, followed by the mesocarp (66.51 mg g−1 DW) and exocarp (26.78 mg g−1 DW). The highest content of naringin was in the pulp of XY-ZM (251.56 ± 1.03 mg g−1 DW), and the lowest content was found in the exocarp of XY-ZM (12.35 ± 0.14 mg g−1 DW). The rhoifolin content was in the following order HY-ZM > GQ > FM > XY-ZM > MY-ZM > HL-ZM, FM > MY-ZM > HY-ZM ~ GQ > XY-ZM > HL-ZM and XY-ZM > FM > HY-ZM > HL-ZM ~ GQ > MY-ZM in the tissues of the exocarp, mesocarp and pulp, respectively. In contrast to the results of the naringin content, the average content of rhoifolin in the exocarp (41.40 mg g−1 DW) was higher than that in the mesocarp (21.22 mg g−1 DW), and the content in the pulp (43.40 mg g−1 DW) was slightly higher than that in the exocarp. The highest content of rhoifolin was in the exocarp of HY-ZM (65.21 ± 1.55 mg g−1 DW), and the lowest was found in the exocarp of HL-ZM (14.31 ± 0.12 mg g−1 DW). In addition, the whole fruit of FM contained the highest average amounts of naringin (77.65 mg g−1 DW) and rhoifolin (36.56 mg g−1 DW). The contents of naringin and rhoifolin were comparable to that of Citrus wilsonii.[Citation29]
Table 2. Narignin and rhoifolin contents in three different fruits tissues of six Citrus grandis ‘Tomentosa’ varietiesa.
As presented in , the contents of meranzin hydrate, bergapten and isoimperatorin of the exocarp of the six CGT varieties varied from 0.82 ± 0.02 to 2.67 ± 0.08 mg g−1 DW, 5.85 ± 0.04 to 19.87 ± 0.35 mg g−1 DW and 2.54 ± 0.01 to 17.35 ± 0.33 mg g−1 DW, respectively. The content of isoimperatorin in the pulp varied from 1.80 ± 0.07 to 9.67 ± 0.26 mg g−1 DW. The meranzin hydrate, bergapten and isoimperatorin contents in the exocarp were ranked as HY-ZM > XY-ZM ~ HL-ZM > FM > MY-ZM > GQ, HL-ZM > HY-ZM > XY-ZM ~ FM > MY-ZM ~ GQ and XY-ZM > HY-ZM ~ MY-ZM > HL-ZM > FM > GQ, respectively. For the isoimperatorin content in the pulp, the ranking was HY-ZM ~ MY-ZM > FM > XY-ZM > GQ > HL-ZM. The highest contents of meranzin hydrate, bergapten and isoimperatorin existed in the exocarp of HY-ZM, HL-ZM and XY-ZM, respectively. Moreover, HY-ZM contained the largest amounts of meranzin hydrate (0.80 mg g−1 DW) and bergapten (4.91 mg g−1 DW) as a whole, and the fruit of XY-ZM contained the highest content of isoimperatorin (5.35 mg g−1 DW).
Table 3. Coumarins contents in three different fruits tissues of six Citrus grandis ‘Tomentosa’ varietiesa.
Identification of the volatile compounds
GC-MS is a powerful technique for the identification of volatile compound, and headspace solid-phase microextraction (HS-SPME) has a lot of advantages such as satisfactory extraction efficiency, good repeatability and less sample amount consuming.[Citation21] Hence, in this study, a combined method of HS-SPME-GC-MS was carried out to extract the volatiles from the exocarp, mesocarp and pulp of the various CGT varieties. Fig. S1 illustrates the total ion chromatograms (TICs) of all samples. The profiles exhibited significant differences in the TICs among the different tissues. Detailed information is presented in Table S2, Table S3 and Table S4. As shown in Table S2, a total of 104 volatiles were detected in the exocarp, including 47 terpenes, 25 alcohols, 17 aldehydes, 5 esters, and 3 alkanes. From Table S3, 82 compounds were identified in the mesocarp containing 41 terpenes, 18 alcohols, 10 alkanes, 8 aldehydes, 4 esters, and 1 oxide. As described in Table S4, 72 compounds were determined altogether in the pulp consisting of 47 terpenes, 10 alkanes, 8 alcohols, 3 aldehydes, 2 esters, 1 ketone, and 1 oxide. Consequently, terpenoid (34.05–81.45%) was the most dominant chemical group among the volatiles discovered in CGT across the different tissues, followed by the alcohols (4.21–32.20%), which is consistent with a previous report.[Citation21]
The major volatiles of the 27 compounds are shown in , consisting mainly of terpenes and alcohols. The most abundant compound in the exocarp on average was germacrene-D (27.40%), followed by nerolidol (7.89%), D-limonene (7.32%), α-terpineol (6.88%), β- caryophyllene (5.07%), geraniol (3.47%) and linalool (3.00%). However, in the mesocarp, D-limonene (15.28%) was the most abundant on average, followed by germacrene-D (12.02%), α-terpineol (7.22%), linalool (5.08%), β-caryophyllene (3.07%), β-myrcene (2.99%) and γ-terpinene (2.62%). In terms of the pulp, germacrene-D (20.72%) was the predominant compound, followed by β-caryophyllene (17.89), D-limonene (10.10%), γ-terpinene (4.64%), β-myrcene (4.21%), nerolidol (3.36%) and β-elemene (2.60%). Notably, γ-muurolene was not detected in all exocarps, and indole was unique in the exocarp samples, which was only described in C. grandis, C. bergamia and C. limon. (±)-δ-Elemene, neryl acetate, geranyl acetate and nerolidol were not detected in all mesocarps, and geraniol was not determined in all pulps. In contrast to a previous report on C. grandis[Citation30], linalool was determined with a percentage higher than 1.00% in almost all samples of CGT. Nerol, neral, geraniol, citral, indole, neryl acetate, β-caryophyllene, γ-elemene, α-caryophyllene, nerolidol, phytane were the major compounds detected in CGT for the first time. It is worth noting that thymol was identified in all exocarps and in the pulp of HY-ZM, which is different from a recent review that described that thymol had not been reported in C. grandis.[Citation31] Recently, thymol was shown to have antifungal, anticancer, antibacterial and hepatoprotective activities.[Citation32–Citation35] Thus, thymol can be used to inhibit oral bacteria and as an antioxidant in food preservation. Moreover, thymol has also been reported as a therapy for wound repair.[Citation36] In the samples of exocarp among the six varieties, the compositions of the main volatiles are almost identical. However, the amounts of volatiles in the mesocarp of FM and GQ are slightly larger than those in the ZhengMao series.
Table 4. Main volatiles compounds in three different fruits tissues of six Citrus grandis ‘Tomentosa’ varieties
Assay of the antioxidant activities
The antioxidative activities of all the samples were assayed by three methods of ABTS, DPPH and FRAP, and the results were expressed as the Trolox equivalent antioxidant capacity, as shown in . The ABTS value showed significant differences among the different varieties, and the highest value (6.14 ± 0.01 mmol TEAC/100 g DW) was found in the pulp of HY-ZM. In the exocarp, mesocarp and pulp, the ABTS radical scavenging ability was ranked as HL-ZM > HY-ZM > FM > MY-ZM > XY-ZM ~ GQ, HL-ZM > HY-ZM > FM > MY-ZM > XY-ZM ~ GQ, and HY-ZM ~ XY-ZM ~ HL-ZM > MY-ZM > FM > GQ, respectively. The rule of the order in the exocarp and the mesocarp was the same. Overall, the radical scavenging abilities by ABTS among the three tissues were ranked as pulp > mesocarp > exocarp, which were in agreement with the results of the phenolic contents. However, there was no such pattern in the results of the DPPH and FRAP methods. For the DPPH and FRAP assays, the highest values were observed in the mesocarp of FM (4.28 ± 0.02 mmol TEAC/100 g) and the pulp of HL-ZM (4.44 ± 0.06 mmol TEAC/100 g). There were three different levels of antioxidant activities measured by the DPPH and FRAP methods, except in the mesocarp sample determined by the DPPH method. The antioxidant activities of the whole fruits were also determined according to the weight ratios and are shown in . Notably, the results of the ABTS, DPPH and FRAP assays were different from each other, which indicated that the complex chemical compositions simultaneously contribute to the antioxidant activities of CGT. The ABTS radical scavenging abilities of CGT in all samples were higher than that of finger millet which is a common staple food for populations in developing countries.[Citation37]
Table 5. The antioxidant activities measured by ABTS, DPPH and FRAPa.
Correlation analysis
Correlation coefficients between antioxidant activity and the phytochemical contents measured in this study are shown in . The content of isoimperatorin is correlated (p < .05) with the antioxidant activity as determined by DPPH and FRAP methods. Remarkably, the contents of naringin, rhoifolin, meranzin hydrate, and bergaptan were strongly correlated (p < .01) with the total phenolic contents. The antioxidant activity of chemical compound was reported to be mainly correlated with their chemical structures. For example, the number and location of hydroxyl groups on the flavonoid ring system determined the antioxidant effect, although other factors also had effects.[Citation38] In our study, the content of isoimperatorin was confirmed as largely responsible for the antioxidant activity of CGT. However, it has been reported that flavonoids are powerful radical quenchers in various systems.[Citation39] Naringin, rhoifolin, meranzin hydrate, and bergapten were highly correlated with the total phenolic contents, and the content of naringin was the primary contributor to the total phenolic contents. Flavonoids, coumarins, volatiles, and polysaccharides are proved to be the main metabolites of CGT fruits. In our previous study, an active acidic polysaccharide was purified and structurally characterized with the ability to the anti-proliferation toward cancer cells.[Citation40] The present study focused on the comparison of main secondary metabolites in CGT fruits among different varieties and parts. The correlation analysis further verified that the antioxidant activities of CGT fruits were not determined solely by single or several metabolites, and complex chemical compositions simultaneously are playing a part to the bioactivity of CGT fruits. Therefore, further work of the global metabolites assay in CGT should be performed.
Table 6. Pearson’s correlation coefficient among total phenolic contents (TPC), flavonoids, coumarins and antioxidant activities
Ranking analysis
A ranking analysis of the TPCs, flavone and coumarin amounts, and antioxidant capacities among the varieties was carried out and is presented in . The rank refers to the whole fruit values calculated according to the weight ratios of the different tissues shown in Table S1. The overall performances of these varieties were examined using a previously reported method. The lowest summative rank was regarded as the highest overall performance of the fruits.[Citation41] Therefore, according to this standard, the best variety is supposed to be FM, which does not have the densest trichomes, thus contradicting the traditional view of the more pubescent the fruit is, the better. However, GQ, whose trichomes are the thinnest, performed the worst in the ranking analysis, which affirmed the traditional view. In addition, the best in the ZhengMao series is HY-ZM.
Table 7. Rank orders of total phenolic contents, flavones and coumarins amounts, and antioxidant capacities of six Citrus grandis ‘Tomentosa’ varities, and the overall performance
Conclusion
In summary, the difference in the phenolic contents among the different fruit tissues was distinct, and in general, the phenolic content could be ranked as pulp > mesocarp > exocarp. The exception was that the exocarp contained more kinds of coumarins and volatiles than did the mesocarp and pulp. The pulp only contained isoimperatorin, and the mesocarp contained none of the coumarins. Terpenoids were the most abundant volatile chemicals in the fruits of CGT, including germacrene-D, D-limonene and β-caryophyllene as the top three compounds. Thymol was identified in all of the exocarps of CGT, and a trace amount of thymol was found in the pulp of HY-ZM. Briefly, the distributions of these secondary metabolites are significant in the different tissues and among the varieties of CGT fruits. With the help of ranking analysis, it can be proposed that FM and HY-ZM exhibit outstanding potentials as premium varieties of CGT. These results may provide important information not only for pharmaceutical manufacturing but also for cultivators and breeders.
Acknowledgments
This research was funded by the Earmarked Fund for China Agriculture Research System [CARS-26], National Key Research and Development Program of China [2017YFD0202005], and Guangdong Science and Technology Plan [2015B090906026].
References
- Smeriglio, A.; Cornara, L.; Denaro, M.; Barreca, D.; Burlando, B.; Xiao, J.; Trombetta, D. Antioxidant and Cytoprotective Activities of an Ancient Mediterranean Citrus (Citrus Lumia risso) Albedo Extract: Microscopic Observations and Polyphenol Characterization. Food Chem. 2019, 279, 347–355. DOI: 10.1016/j.foodchem.2018.11.138.
- Li, P. L.; Liu, M. H.; Hu, J. H.; Su, W. W. Systematic Chemical Profiling of Citrus Grandis ‘tomentosa’ by Ultra-fast Liquid chromatography/diode-array detector/quadrupole Time-of-flight Tandem Mass Spectrometry. J. Pharm. Biomed. Anal. 2014, 90, 167–179. DOI: 10.1016/j.jpba.2013.11.030.
- Yu, X.; Liu, Q.; Xie, Z.; Lam, S.; Xu, X. Chromatographic Fingerprint Analysis of Exocarpium Citri Grandis by High-Performance Liquid Chromatography Coupled with Diode-Array Detector. Food Anal. Methods. 2014, 8(7), 1868–1875. DOI: 10.1007/s12161-014-0071-9.
- Zeng, X.; Su, W.; Zheng, Y.; Liu, H.; Li, P.; Zhang, W.; Liang, Y.; Bai, Y.; Peng, W.; Yao, H. UFLC-Q-TOF-MS/MS-Based Screening and Identification of Flavonoids and Derived Metabolites in Human Urine after Oral Administration of Exocarpium Citri Grandis Extract. Molecules. 2018, 23(4), 895. DOI: 10.3390/molecules23040895.
- Liang, Y.; Huang, Z.; Chen, H.; Zhang, T.; Ito, Y. Preparative Isolation and Purification of Two Closely Related Glycosidic Flavonoids from Exocarpium Citri Grandis by High-Speed Countercurrent Chromatography. J. Liq. Chromatogr. Relat. Technol. 2007, 30(3), 419–430. DOI: 10.1080/10826070601084886.
- Lim, H. K.; Moon, J. Y.; Kim, H.; Cho, M.; Cho, S. K. Induction of Apoptosis in U937 Human Leukaemia Cells by the Hexane Fraction of an Extract of Immature Citrus Grandis Osbeck Fruits. Food Chem. 2009, 114(4), 1245–1250. DOI: 10.1016/j.foodchem.2008.10.088.
- He, X.; Zhang, L.; Chen, J.; Sui, J.; Yi, G.; Wu, J.; Ma, Y. Correlation between Chemical Composition and Antifungal Activity of Clausena Lansium Essential Oil against Candida Spp. Molecules. 2019, 24, 7. DOI: 10.3390/molecules24071394.
- Duan, L.; Guo, L.; Dou, L. L.; Yu, K. Y.; Liu, E. H.; Li, P. Comparison of Chemical Profiling and Antioxidant Activities of Fruits, Leaves, Branches, and Flowers of Citrus Grandis ‘tomentosa’. J. Agric. Food Chem. 2014, 62(46), 11122–11129. DOI: 10.1021/jf5036355.
- Jiang, K.; Song, Q.; Wang, L.; Xie, T.; Wu, X.; Wang, P.; Yin, G.; Ye, W.; Wang, T. Antitussive, Expectorant and Anti-inflammatory Activities of Different Extracts from Exocarpium Citri Grandis. J. Ethnopharmacol. 2014, 156, 97–101. DOI: 10.1016/j.jep.2014.08.030.
- Wang, X.; Xu, Y.; Zhang, S.; Cao, L.; Huang, Y.; Cheng, J.; Wu, G.; Tian, S.; Chen, C.; Liu, Y.;, et al. Genomic Analyses of Primitive, Wild and Cultivated Citrus Provide Insights into Asexual Reproduction. Nat. Genet. 2017, 49(5), 765–772. DOI: 10.1038/ng.3839.
- Gottwald, T. R. Current Epidemiological Understanding of Citrus Huanglongbing. Annu. Rev. Phytopathol. 2010, 48, 119–139. DOI: 10.1146/annurev-phyto-073009-114418.
- Tamene, D.; Endale, M. Antibacterial Activity of Coumarins and Carbazole Alkaloid from Roots of Clausena Anisata. Adv. Pharmacol. Sci. 2019, 2019, 1–8. DOI: 10.1155/2019/5419854.
- Kong, F.; Bi, Y.; Yan, C.; Zeng, Z. Orthogonal Test Design for Optimization of the Ultrasonic Extraction of Naringin from Citrus Grandis Tomentosa. J. Med. Plants Res. 2013, 7(12), 720–726. DOI: 10.5897/JMPR12.104.
- Luo, Y. L.; Zhang, C. C.; Li, P. B.; Nie, Y. C.; Wu, H.; Shen, J. G.; Su, W. W. Naringin Attenuates Enhanced Cough, Airway Hyperresponsiveness and Airway Inflammation in a Guinea Pig Model of Chronic Bronchitis Induced by Cigarette Smoke. Int. Immunopharmacol. 2012, 13(3), 301–307. DOI: 10.1016/j.intimp.2012.04.019.
- Gao, S.; Li, P.; Yang, H.; Fang, S.; Su, W. Antitussive Effect of Naringin on Experimentally Induced Cough in Guinea Pigs. Planta Med. 2011, 77(1), 16–21. DOI: 10.1055/s-0030-1250117.
- Karakaya, S.; Şimşek, D.; Özbek, H.; Güvenalp, Z.; Altanlar, N.; Kazaz, C.; Kılıc, C. Antimicrobial Activities of Extracts and Isolated Coumarins from the Roots of Four Ferulago Species Growing in Turkey. Iran. J. Pharm. Res. World. 2019, 18(3), 1516–1529. DOI: 10.22037/IJPR.2019.1100718.
- Wang, G.; Liu, Y.; Zhang, L.; An, L.; Chen, R.; Liu, Y.; Luo, Q.; Li, Y.; Wang, H.; Xue, Y. Computational Study on the Antioxidant Property of Coumarin-fused Coumarins. Food Chem. 2020, 304, 125446. DOI: 10.1016/j.foodchem.2019.125446.
- Dai, H.; Huang, M.; Qian, J.; Liu, J.; Meng, C.; Li, Y.; Ming, G.; Zhang, T.; Wang, S.; Shi, Y.;, et al. Excellent Antitumor and Antimetastatic Activities Based on Novel coumarin/pyrazole Oxime Hybrids. Eur. J. Med. Chem. 2019, 166, 470–479. DOI: 10.1016/j.ejmech.2019.01.070.
- Ayodele, O. O.; Onajobi, F. D.; Osoniyi, R. O. In Vitro Anticoagulant Effect of Crassocephalum Crepidioides Leaf Methanol Extract and Fractions on Human Blood. J. Exp. Pharmacol. 2019, 11, 99–107. DOI: 10.2147/JEP.S218261.
- Luo, M.; Luo, H.; Hu, P.; Yang, Y.; Wu, B.; Zheng, G. Evaluation of Chemical Components in Citri Reticulatae Pericarpium of Different Cultivars Collected from Different Regions by GC-MS and HPLC. Food Sci. Nutr. 2018, 6(2), 400–416. DOI: 10.1002/fsn3.569.
- Xie, Z.; Liu, Q.; Liang, Z.; Zhao, M.; Yu, X.; Yang, D.; Xu, X. The GC/MS Analysis of Volatile Components Extracted by Different Methods from Exocarpium Citri Grandis. J. Anal. Methods Chem. 2013, 2013, 1–8. DOI: 10.1155/2013/918406.
- Conde-Hernández, L. A.; Guerrero-Beltrán, J. Á. Total Phenolics and Antioxidant Activity of Piper Auritum and Porophyllum Ruderale. Food Chem. 2014, 142, 455–460. DOI: 10.1016/j.foodchem.2013.07.078.
- Re, R.; Pellegrini, N.; Proteggente, A.; Pannala, A.; Yang, M.; Rice-Evans, C. Antioxidant Activity Applying an Improved ABTS Radical Cation Decolorization Assay. Free Radical Biol. Med. 1999, 26(9–10), 1231–1237. DOI: 10.1016/S0891-5849(98)00315-3.
- Brand-Williams, W.; Cuvelier, M. E.; Berset, C. L. W. T. Use of a Free Radical Method to Evaluate Antioxidant Activity. LWT Food Sci. Technol. 1995, 28(1), 25–30. DOI: 10.1016/S0023-6438(95)80008-5.
- Benzie, I. F.; Strain, J. J. Ferric reducing/Antioxidant Power Assay: Direct Measure of Total Antioxidant Activity of Biological Fluids and Modified Version for Simultaneous Measurement of Total Antioxidant Power and Ascorbic Acid Concentration. Methods Enzymol. 1999, 299, 15–27. DOI: 10.1016/S0076-6879(99)99005-5.
- Zhang, Y.; Sun, Y.; Xi, W.; Shen, Y.; Qiao, L.; Zhong, L.; Ye, X.; Zhou, Z. Phenolic Compositions and Antioxidant Capacities of Chinese Wild Mandarin (Citrus Reticulata blanco) Fruits. Food Chem. 2014, 145, 674–680. DOI: 10.1016/j.foodchem.2013.08.012.
- Chang, X.; Ye, Y.; Pan, J.; Lin, Z.; Qiu, J.; Guo, X.; Lu, Y. Comparative Assessment of Phytochemical Profiles and Antioxidant Activities in Selected Five Varieties of Wampee (Clausena lansium) Fruits. Int. J. Food Sci. Technol. 2018, 53(12), 2680–2686. DOI: 10.1111/ijfs.13877.
- Dong, J.; Ma, X.; Wei, Q.; Peng, S.; Zhang, S. Effects of Growing Location on the Contents of Secondary Metabolites in the Leaves of Four Selected Superior Clones of Eucommia Ulmoides. Ind. Crops Prod. 2011, 34(3), 1607–1614. DOI: 10.1016/j.indcrop.2011.06.007.
- Cheng, L.; Ren, Y.; Lin, D.; Peng, S.; Zhong, B.; Ma, Z. The Anti-inflammatory Properties of Citrus Wilsonii Tanaka Extract in IPS-induced RAW 264.7 And Primary Mouse Bone Marrow-derived Dendritic Cells. Molecules. 2017, 22, 7. DOI: 10.3390/molecules22071213.
- Chung, H.; Chung, W. Y.; Yoo, E. S.; Cho, S. K.; Oh, S. K.; Kim, Y. S. Characterization of Volatile Aroma-active Compounds in Dangyooja (Citrus Grandis osbeck). J. Korean Soc. Appl. Biol. Chem. 2012, 55(1), 133–136. DOI: 10.1007/s13765-012-0023-2.
- Gonzalez-Mas, M. C.; Rambla, J. L.; Lopez-Gresa, M. P.; Blazquez, M. A.; Granell, A. Volatile Compounds in Citrus Essential Oils: A Comprehensive Review. Front. Plant Sci. 2019, 10, 12. DOI: 10.3389/fpls.2019.00012.
- Cai, R.; Zhang, M.; Cui, L.; Yuan, Y.; Yang, Y.; Wang, Z.; Yue, T. Antibacterial Activity and Mechanism of Thymol against Alicyclobacillus Acidoterrestris Vegetative Cells and Spores. LWT Food Sci. Technol. 2019, 105, 377–384. DOI: 10.1016/j.lwt.2019.01.066.
- Kong, J.; Zhang, Y.; Ju, J.; Xie, Y.; Guo, Y.; Cheng, Y.; Qian, H.; Quek, S. Y.; Yao, W. Antifungal Effects of Thymol and Salicylic Acid on Cell Membrane and Mitochondria of Rhizopus Stolonifer and Their Application in Postharvest Preservation of Tomatoes. Food Chem. 2019, 285, 380–388. DOI: 10.1016/j.foodchem.2019.01.099.
- Islam, M. T.; Khalipha, A. B. R.; Bagchi, R.; Mondal, M.; Smrity, S. Z.; Uddin, S. J.; Shilpi, J. A.; Rouf, R. Anticancer Activity of Thymol: A Literature-based Review and Docking Study with Emphasis on Its Anticancer Mechanisms. IUBMB Life. 2019, 71(1), 9–19. DOI: 10.1002/iub.1935.
- Geyikoglu, F.; Yilmaz, E. G. L.; Erol, H. S.; Koc, K.; Cerig, S.; Ozek, N. S.; Aysin, F. Hepatoprotective Role of Thymol in Drug-Induced Gastric Ulcer Model. Ann. Hepatol. 2018, 17(6), 980–991. DOI: 10.5604/01.3001.0012.7198.
- Costa, M. F.; Durco, A. O.; Rabelo, T. K.; Barreto, R. S. S.; Guimaraes, A. G. Effects of Carvacrol, Thymol and Essential Oils Containing Such Monoterpenes on Wound Healing: A Systematic Review. J. Pharm. Pharmacol. 2019, 71(2), 141–155. DOI: 10.1111/jphp.13054.
- Xiang, J.; Apea-Bah, F. B.; Ndolo, V. U.; Katundu, M. C.; Beta, T. Profile of Phenolic Compounds and Antioxidant Activity of Finger Millet Varieties. Food Chem. 2019, 275, 361–368. DOI: 10.1016/j.foodchem.2018.09.120.
- Lien, E. J.; Ren, S.; Bui, H. H.; Wang, R. Quantitative Structure-activity Relationship Analysis of Phenolic Antioxidants. Free Radical Biol. Med. 1999, 26(3–4), 285–294. DOI: 10.1016/S0891-5849(98)00190-7.
- Zhang, L.; Liu, P.; Li, L.; Huang, Y.; Pu, Y.; Hou, X.; Song, L. Identification and Antioxidant Activity of Flavonoids Extracted from Xinjiang Jujube (Ziziphus Jujube mill.) Leaves with Ultra-high Pressure Extraction Technology. Molecules. 2018, 24(1). DOI: 10.3390/molecules24010122.
- Fan, R.; Xie, Y.; Zhu, C.; Qiu, D.; Zeng, J.; Liu, Z. Structural Elucidation of an Acidic Polysaccharide from Citrus Grandis ‘tomentosa’ and Its Anti-proliferative Effects on LOVO and SW620 Cells. Int. J. Biol. Macromol. 2019. DOI: 10.1016/j.ijbiomac.2019.07.117.
- Silva, K. D. R. R.; Sirasa, M. S. F. Antioxidant Properties of Selected Fruit Cultivars Grown in Sri Lanka. Food Chem. 2018, 238, 203–208. DOI: 10.1016/j.foodchem.2016.08.102.