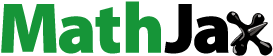
ABSTRACT
In this study, chitosan (CTS) anti-browning coating film was applied to fresh cut lemon slices. The effects of freshly cut lemon slices on peroxidase (POD), polyphenol oxidase (PPO), phenolic acid and storage quality were investigated at different storage temperatures (0°C, 4°C, 7°C and 10°C). Results demonstrated that 0°C had an excellent inhibitory effect on the activity of POD, and PPO, as well as a slight impact on the seven primary phenolic acids, the content of which decreased from 0.94 mg/g and 10.01 mg/g to 0.65 mg/g and 8.87 mg/g after a storage period of 10 d. Moreover, the malonaldehyde content (MDA), relative conductivity, and 2,2-diphenyl-1-picrylhydrazyl (DPPH, free radical) radical scavenging capacity were 0.35 μmol/g, 64.16%, and 91.4%, respectively, while the titratable acidity (TA) and vitamin C (VC) content in the juice were 11.6 g/kg and 33.97 mg/g, respectively. The aroma components denoted by D-limonene, β-pinene, and hydrophyllene also presented a high percentage after 10 d, indicating that combining the treatment of CTS coating with clove oil as a treatment at low temperatures effectively maintained the qualitative properties of freshly cut lemons.
Introduction
Lemon (Citrus limon L. Burm. f.) is one of the key cultivated citrus species after oranges and mandarins,[Citation1] and is well-known for its economic, nutritional and health values. It is rich in natural chemical components such as vitamin C (VC), dietary fiber, citric acid, carotenoids, phenolic compounds, and essential oils.[Citation2–Citation4] Challenges that may occur during transportation, include the abscission of the lemon pedicle and decay due to high transportation temperatures during transit. Therefore, the commercial utilization of lemons may be reduced, limiting further expansion to various additional industries. The efforts to identify safe preservation options for fruits and vegetables during storage are increasing and include techniques such as the application of low temperatures and chitosan (CTS) with essential oils. These methods have been widely utilized since they delay senescence and maintain quality.
Low-temperature storage is one of the main techniques used to extend the shelf life of fresh fruit and vegetables, since it reduces thermal decomposition, as well as respiration processes.[Citation5] Over the past few decades, the interest in developing and using bio-based materials to extend the shelf life and improve the quality of fresh, frozen, and formulated food products has been increasing rapidly.[Citation6] CTS is a nontoxic, high molecular polymer derived from the outer shell of crustaceans, and due to its excellent film-forming and biochemical properties, it has become an ideal compound for the preservation of fresh produce.[Citation7,Citation8] Furthermore, CTS, is a natural carbohydrate biopolymer that contains contain different functional groups (primary -OH, secondary -OH and -NH),[Citation9,Citation10] and is widely used during the storage of fruits and vegetables due to its film-forming and antimicrobial activities.[Citation11] According to results obtained by Xing et al.,[Citation12] a CTS-oil coating, enriched with cinnamon oil, is a promising alternative for improving the preservation quality of sweet peppers. Song et al.[Citation13] demonstrated that treating loquat fruit with a CTS/nano-silica coating at 5°C effectively enhanced the chilling tolerance of loquat fruit, extending its shelf life and exhibiting acceptable external and internal quality. Furthermore, as natural plant protectants, essential oils display low mammalian toxicity and fewer detrimental effects on the environment.[Citation14–Citation16] When incorporated into the CTS coating as a carrier, these essential oils can effectively control pests and disease, therefore, providing safe food as a result.[Citation17]
Numerous studies have been conducted to develop and apply CTS coatings containing essential oils, such as cinnamon oil, lemon essential oil, and clove oil, for improving the quality of both fresh fruits and processed fruits and vegetables.[Citation18–Citation20] Xing et al.[Citation21] reported that treatment with a CTS-oil coating containing cinnamon oil could control the fungi in wound-inoculated fruit. Furthermore, Hosseini[Citation22] proved that CTS combined with savory essential oil (SEO) or tarragon essential oil (TEO) could effectively reduce weight loss (WL) while maintaining the VC content and titratable acidity (TA). Xu et al.[Citation23] reported that a CTS coating containing anti-browning agents combined with cinnamon oil fumigation maintained the quality of freshly cut pear slices and controlled the development of rot. Xing et al[Citation24] demonstrated the combined application of anti-browning agents, oil fumigation, and moderate vacuum packaging (MVP) delayed the microbiological deterioration of lotus root slices while prolonging its shelf-life. According to Saki, M. et al.,[Citation25] a CTS coating combined with thymol essential oil provided a promising option for enhancing the storage quality of fresh figs. Although CTS coatings and essential oils have been applied to a variety of fresh produce, no published research exists regarding the effect of CTS coatings containing clove oil on the qualitative properties of freshly cut lemons when exposed to a diverse range of temperatures.
This investigation aims to evaluate the effects of a CTS-oil coating on the physiological attributes and preservation quality of Anyue Lemons during storage at different temperatures. The weight loss (WL), sensory evaluation, titratable acidity (TA) and vitamin C (VC) content, as well as the degree of browning in these lemons, are determined using different treatments during storage at 0°C, 4°C, 7°C, and 10°C, respectively, for 10 d. Furthermore, the relative conductivity of peroxidase (POD), polyphenol oxidase (PPO), malonaldehyde content (MDA), and 2,2-diphenyl-1-picrylhydrazyl (DPPH) is also evaluated during the entire storage period.
Materials and methods
Fruit material
The lemon fruits (Limon cv. Eureka) at commercially mature stage were obtained from Anyue county, Sichuan Province, China, and were transported immediately after harvesting to the College of Food and Bio-engineering Laboratory at Xihua University. The lemons were sturdy having uniform shape and size without any mechanical damage. Upon arrival at the laboratory, the lemons were thoroughly washed and kept at room temperature until complete drying. After that, the lemons were cut into 5 ~ 8 mm round slices with a sharp kitchen knife. The fresh-cut lemon slices were immediately dipped in composite chitosan coating preservation solution for 2 min. After dipping, the excess water was drained out and slices were packed, then stored at 0 ± 0.5ºC for further analysis.
Preparation of the CTS-based coating
The CTS-based coating was prepared according to the method reported by Xu et al.[Citation26] A stock solution of CTS (12.3 g) was dissolved in 1000 mL of distilled water containing 0.5% acetic acid and 0.3% calcium chloride, and stirred at room temperature for 10 min (CTS, deacetylated > 90%, Jinan Haidebei Marline Bioengineering Co. Ltd., Jinan, China). Then, clove oil at a concentration of 0.10% mixed with sucrose fatty acid esters (0.1%), was added and stirred for another 30 min. Food grade clove oil was purchased from the Xuzhou Aipeisi Commodity Trading Co. Ltd. (Henan, China). The final solutions were homogenized at 10,000 rpm for 15 s in aseptic conditions and left to stand in a low-temperature environment for 1 h while protected from light, after which the coating was obtained.
WL, TA, and VC
The TA and WL in the samples were determined using the method described by Odriozola-Serrano et al.[Citation27] and Kumar et al.[Citation28] A 10.0 g sample, 100 mL distilled water, and two drops of phenolphthalein were mixed and titrated with NaOH 0.1 N until it reached pH 8.2, after which measurements were taken using a pH-meter (Orion Versa, Thermo Fisher Scientific, USA). The TA was expressed as a percentage and citric acid was employed as a reference (meq = 0.064). The value of WL was expressed as the percentage loss of the initial weight using the following formula:
where W0 is the initial weight (g) of the lemons and Wt is the final weight (g) of the lemons on the sampling date.
The VC content in the lemons was determined using the method developed by Scherer et al.,[Citation29] with some modifications. A portion of 1.0 g of freshly cut lemon pieces was added to 10.0 mL of a 95:5 oxalic acid-methanol solution in an ice bath. The mixture was stirred and centrifuged at 10,000 rpm for 10 min at 4°C using a centrifuge 5804 R. The sample was passed through a Millipore 0.45 µm membrane and injected into the HPLC system.
The HPLC system was equipped with a Varian 325-LC UV Detector operating at 245 nm. According to the method of Xing et al.[Citation12]with slight modifications, duplicates of 10 µL of each extract were injected into a reverse-phase C18 Varian HPLC column (250 mm×4.6 mm) and used as the stationary phase, while a 0.1% solution of oxalic acid-methanol was used as the mobile phase. The flow rate was fixed at 1 mL/min at room temperature, and the results were expressed as milligram (mg) of VC in 100 g of freshly cut lemon slices.
Degree of browning in the fruit and the sensory evaluation
The degree of browning was determined according to the method described by Hu et al.[Citation30] with some modifications. A 1.0 g pulp sample from the freshly sliced lemons was homogenized with 10.0 mL distilled water at 4°C, then centrifuged at 6000 rpm for 10 min. The absorbance was measured using a spectrophotometer at 410 nm, and the degree of browning was expressed as A410 × 10.
The sensory attributes of the freshly cut lemon slices, such as the appearance of the skin and the flesh, as well as the texture and odor of the fruit, were determined using a 10-point hedonic scale (i.e. a score of 2 was extremely poor; 4 was poor; 6 was acceptable; 8 was good, and 10 was excellent).[Citation31,Citation32] Furthermore, the samples were evaluated by ten trained adult panelists selected at random.
MDA content, PPO, and POD activity, and relative conductivity measurement
TBARS were determined and expressed as MDA equivalents, according to the method involving PPO activity, as described by Xing et al.[Citation33] with slight modifications. A 1.0 g sample of lemon flesh was homogenized with 5.0 mL of 100 g/L trichloroacetic acid and centrifuged for 20 min at 10,000 × g and 4°C. A 1 mL volume of the supernatant was mixed with 2 mL of 0.67% thiobarbituric acid (TBA) previously dissolved in 100 g/L trichloroacetic acid. The reaction solution was heat-treated for 20 min at 95°C, cooled rapidly, and then centrifuged for 20 min at 10,000 g to clarify the precipitation, after which absorbance measurements were performed in triplicate at 450 nm, 532 nm, and 600 nm, respectively.
The extraction and assay of the PPO activity were performed as described by Serradell et al.[Citation34] where a 2.0 g lemon peel sample was homogenized in 20 mL of phosphate buffer (1 mmol/L PEG, 4% (W/V) PVPP, and 1% (W/V) Triton X-100) in an ice bath, after which the solution was centrifuged at 6000 rpm for 10 min at 4°C. The reaction mixture consisted of 80 μL 0.5 mol/L catechol, and 100 μL 0.05 mol/L phosphate buffer (pH 6.5), and was incubated at 35°C for 5 min, after which 20 μL crude PPO was added, and the absorbance changes were measured at 420 nm. One unit (U) of PPO activity was defined as a change of 0.001 at 420 nm in the absorbance per min.
The POD activity was determined and assayed spectrophotometrically using a modified method of that described by Wang et al.[Citation35] Here, a 2.0 g lemon peel sample was homogenized in 20 mL of phosphate buffer (1 mmol/L PEG, 4% (W/V) PVPP, and 1% (W/V) Triton X-100) in an ice bath, after which the solution was centrifuged at 6000 rpm for 10 min at 4°C. Then, the supernatant was collected for the POD activity assay. The reaction cuvette contained 60 μL 0.05 mol/L guaiacol, 20 μL of the enzyme solution (the blank group for the sodium phosphate buffer), and 20 μL 0.05 mol/L dissolved hydrogen peroxide. The absorbance changes of the mixture were recorded at 15 s intervas at 470 nm. An enzyme activity U was defined spectrophotometrically as an increase of 1 in absorbance per minute per gram of fresh weight.
Relative conductivity, expressed by membrane permeability (Lt/L0), was performed according to the method outlined by Zhang et al.,[Citation36] with minor modifications. The lemon slices (2.0 g) were placed in flasks with 20.0 mL deionized water and soaked for 10 min. The sample was washed three times with deionized water, after which 20.0 mL was used to soak the flasks for 10 min. The electrical conductivity (Lt) of the solution was measured using a conductivity meter. The flasks containing the solution were heated at 100°C for 15 min and rapidly cooled, after which the electrical conductivity of the solution was measured again (L0).
DPPH, total phenol, and phenolic acid content
The DPPH free radical-scavenging activity was evaluated following the method reported by Elfalleh et al.[Citation37] with some modifications. The lemon peel extract was combined with ethanol to prepare a test solution. A 1.0 g aliquot of the lemon extract sample was added to 10.0 mL of ethanol solution (95%). The mixture was shaken vigorously and left to stand for 60 min at room temperature, after which the absorbance was recorded at 517 nm to determine the concentration of the remaining DPPH. Each value represented the average of three replicates, and the results were expressed as %DPPH inhibition and calculated using the following equation:
where A0 is the absorbance of the control, and A1 is the absorbance of the sample.[Citation38] The total phenolic content of the extract was obtained using a Folin-Ciocalteu assay as described by Du et al.[Citation39] with some modifications. Briefly, 1 g of lemon peel powder was macerated with 20.0 mL of methanol 60%. The mixture was treated ultrasonically at 150 W for 15 min, after which the liquid fraction was recovered and stored at 4°C (sample). This process induced the formation of a blue-colored complex, the absorbance of which was measured against a blank at 765 nm using a microplate spectrophotometer. The experiment was performed by preparing a standard curve using gallic acid as a standard (100–1000 μg mL−1; R2 = 0.9995). The results were calculated as gallic acid equivalents (GAE), and all measurements were performed in triplicate.
Aroma component determination
The aroma components were determined following the method reported by Qiao et al.[Citation40] with minor modifications. The flow rate of the helium carrier gas on the TR-5 MS column (30 m × 0.25 mm×0.25 μm, Thermo Fisher Scientific, USA) was 1 mL/min. The operating parameters were as follows: Injection temperature, 250°C; interface heating, 280°C; ion source heating, 260°C; EI mode, 70 eV; scan range, 45–400 amu. The column was maintained at 40°C for 3 min, after which the temperature was increased to 160°C at 2°C/min, where it was kept for 1 min, and increased again to 200°C at 5°C/min, and maintained for 1 min. Finally, the temperature was increased to 240°C at a rate of 8°C/min and maintained for 3 min with a split ratio of 10:1. The relative content of each compound was calculated as the percentage of the total chromatographic area, and the results were expressed as means of the experiments performed in triplicate.
Statistical analysis
The experimental data were analyzed using SPSS 20.0 software (SPSS Inc., Chicago, IL) and OriginPro 9.0 software. The mean values were calculated and reported as the mean ± SD (standard deviation, n = 3). One-way analysis of variance followed by the least significant difference (P < .05) test was performed on the data presented in the figures and tables to determine the significant factors.
Results and discussion
WL, TA, and VC changes
WL is usually associated with the loss of water. The results in ) indicates that freshly cut lemon slices stored at 10°C presented the highest WL (4.28%), while exposure to 0°C presented the lowest WL (0.73%) after 6 d of storage. The experiment at 10°C had to be discontinued due to the severe degradation of the lemon samples after 8 d. At the end of the storage period, the WL of the lemon slices exposed to 0°C was 1.16%, while the highest WL was observed at 7°C. Moreover, the effect of different storage temperatures on the TA content of the freshly cut lemon slices is shown in ). The results revealed that the TA decreased in conjunction with prolonged storage time, and after 10 d at 7°C and 10°C, respectively, the TA, content in the lemon samples was significantly lower than the initial value (P < .01). The lemon slices exposed to 10°C presented the most substantial decrease in TA whereas the slices in the 0°C environment showed the lowest decrease after 10 d of storage with a TA level at 9.5%. However, the changes observed in the total VC content of coated fruits while exposed to four different temperatures presented a downward trend during 10 d of storage ()). The original VC content of the lemons was 38.2 mg/100 g, while VC retention was significantly affected by both the storage temperature and storage time. Although the VC level decreased throughout storage at each respective temperature, the use of a low temperature significantly reduced the loss of VC in the lemon samples. After 10 d of storage, the VC retention in the lemons at 0°C, 4°C, and 7°C were 33.97 mg/g, 29.55 mg/g, and 25.96 mg/g (P < .01), respectively.
Figure 1. Effects of different storage temperature on weight loss rate (a), TA (b) and VC (c) of fresh-cut lemon.
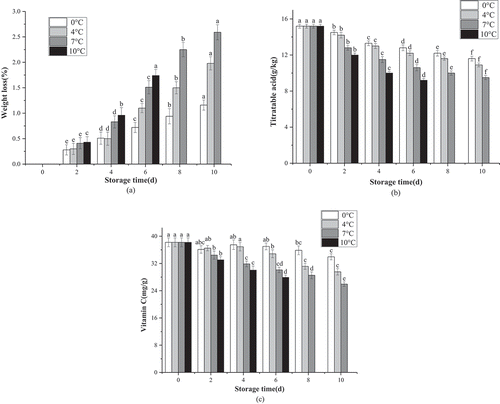
Storing the fruit at low temperatures not only delayed browning but also maintained high relative humidity, thereby reducing WL, as well as water loss or transpiration, which was another vital factor affecting the quality of the freshly cut fruits.[Citation41] The reason for lower WL and higher TA in the lemon slices exposed to low temperatures could be ascribed to the slower ripening rate in this environment, which was expected since organic acids, malic acid, and citric acid were used as substrates for the enzymatic reactions of respiration.[Citation41] In this experiment, the TA declined more slowly in fruit kept at 0°C than at 7°C. Hong K et al.[Citation42] obtained similar results with pineapple where fruits stored at 6°C displayed a higher TA level than those stored at 10°C. VC is a critical endogenous antioxidant in lemons and is easily lost during storage and processing. As such, the CTS coating reportedly slowed down the VC loss by acting as an abiotic elicitor generating reactive oxygen species (ROS), which are scavenged by antioxidant compounds such as VC.[Citation43,Citation44] Moreover, studies found that a methylcellulose-based edible coating reduced the VC loss of both commodities due to the low O2 permeability of the coatings while keeping the fruit away from oxygen delaying the deteriorative oxidation reaction of VC.[Citation45]
Changes in the degree of browning and sensory quality of freshly cut lemon slices
The degree of browning in samples exposed to four different temperatures increased at varying rates, and for the freshly cut lemon slices was more evident at 7°C, with the browning increasing from 0.44 to 2.3 compared to 1.81 at 0°C after 10 d in storage. Furthermore, as revealed in ), the sensory score gradually declined in conjunction with increased storage temperature. High sensory acceptability was evident after 6 d of storage at 0°C, 4°C, and 7°C (P < .01), respectively. After a storage period of 10 d, the scores at temperatures of 0°C, 4°C, and 7°C were 7.04, 6.4, and 5.8 (P < .01), respectively, while the fourth sample group of lemons displayed the lowest sensory acceptability after 10 d of storage at 10°C ()).
Figure 2. Browning degree (a) and sensory score (b) of fresh-cut lemon slices at different storage temperature.
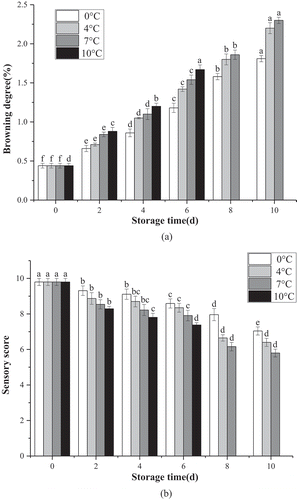
Results demonstrated that the CTS-oil coating and low-temperature storage conditions improved the sensory quality of lemons slices during storage while simultaneously providing better control of the degree of browning(Figure S1). Moreover, Min T et al. described the significant effect of low temperatures on the level of browning in freshly cut lotus root.[Citation46] Low-temperature storage can effectively inhibit the oxidation process responsible for the formation of phenolic compounds, and can also effectively control the degree of browning in freshly cut coated lemon slices during storage.[Citation47] The increase in the degree of browning at higher temperatures further supports the efficacy of low temperatures in maintaining the quality of freshly cut lemons slices. Furthermore, sensory acceptability was improved by low temperatures, as well as the addition of essential oil, which could be due to the high decay incidence and changes in carbohydrates, proteins, amino acids, lipids and phenolic compounds, influencing the flavor of fresh fruits. The highest score was attributed to the lowest level of water loss from the fruit surface and retention of a better balance between the sugars and acids in the fruit juice.[Citation48]
Changes in POD, PPO activity, MDA content, and the relative conductivity in the lemon peel
The POD, PPO activity, MDA content, and relative conductivity in the freshly cut lemon slices stored at different temperatures for 10 d were investigated. The changes in PPO and POD activity occurred in conjunction with an increase in the degree of browning (). The POD activity increased rapidly at 7°C from the initial value of 14.63 U to 20.04 U after 4 d and then decreased to 10.52 U after 10 d, while other enzymatic activity rose to its highest levels in 2 d at 14.25 U (0°C), 15.54 U (4°C), and 17.87 U (10°C), respectively. However, ) illustrates that, the PPO activity in the freshly cut lemon peel initially increased, followed by a decline, in conjunction with an extension of storage time, Furthermore, the PPO activity in the samples exposed to four different temperatures increased to varying degrees of increase, from the initial value of 21.90 U to17.45 U, 23.14 U, 35.81 U, and 32.05 U, respectively, after 6 d. The lemon samples stored at 0°C exhibited the lowest PPO activity, which was only 10.18 U after 10 d of storage. The MDA and electrolyte leakage content were used as direct indicators of membrane injury.[Citation36] ) indicates a continuous increase in the MDA content of the four groups of lemons during storage, while a temperature of 0°C significantly inhibited a rise in the MDA content. After 6 d of storage, the MDA content in the samples exposed to 0°C, 4°C, and 7°C was 0.27 μmol/g, 0.33 μmol/g, and 0.42 μmol/g (P < .01), respectively, while temperatures of 4°C and 7°C exhibited no significanct differences (P > .05). At the end of storage, the MDA content in the freshly cut lemon slices stored at 0°C was 0.36 μmol/g, which was lower than the samples stored at 7°C. Changes in electrolyte leakage are presented in ), indicating that the initial value was 27.30%. After 6 d of storage, the electrolyte leakage contents of the samples exposed to 0°C, 4°C, and 7°C were 64.16%, 64.54%, and 67.92%, respectively. The membrane permeability of the lemon slices stored at 10°C was substantially higher than the samples stored at 0°C, indicating that low temperatures maintain higher membrane integrity during storage.
Figure 3. POD (a), PPO (b), MOD (c) activities and relative electrolytic leakage (d) of fresh-cut lemon during different storage temperatures.
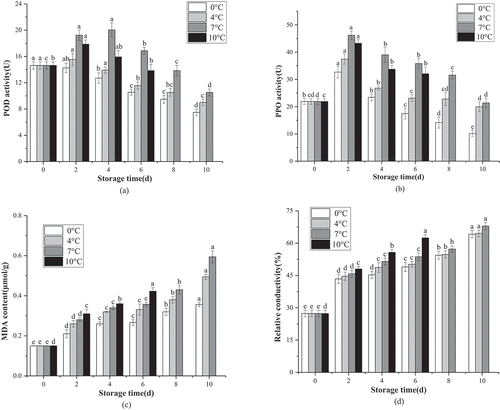
POD and PPO typically catalyze reactions and cause the discoloration of fruits and vegetables.[Citation49] POD enzymes oxidize phenolics to quinones, which are condensed to form browning polymers in the presence of H2O2.[Citation42] This study shows that low temperatures can effectively inhibit PPO enzyme activity, thereby reducing the storage in the process of enzymatic browning substrate consumption happen. Liu et al.[Citation47] reported that PPO activity was an essential factor affecting enzymatic browning, except for phenolics and VC. Browning resulted from polyphenol oxidation by PPO, as well as the accumulation of phenolic compounds after cold storage based on antioxidant capacity. Therefore, a balance may be evident between the antioxidation and enzymatic browning of phenolics when the fruits are stored at low temperatures. ROS accumulation can cause oxidative damage to lipids, forming toxic products such as MDA, which is often used as an index of cell oxidative damage since it is the product of lipid peroxidation.[Citation50] Combined treatment by a CTS-based coating and a low-temperature storage environment was an effective method for controlling the accumulation of MDA and reducing oxidative damage during storage. Additional studies have also indicated that low temperatures can inhibit MDA content in cold-stressed fruits.[Citation51] This result may be due to low temperatures enhancing the chilling resistance in fruit by regulating the activity of PPO, the production of MDA, and secondary metabolites (total phenolics and anthocyanin).[Citation42] Electrolyte leakage is generally considered an indirect indicator of cell membrane damage resulting from adverse circumstances and the senescence of tissues. These results showed that membrane permeability, as an indicator of membrane integrity, gradually increased in conjunction with temperature and storage time. The lower relative leakage rate in the 0°C storage conditions indicated that the membrane integrity was better maintained during cold storage.
Effect of DPPH, total phenol content, and phenolic acid content on the lemon peel
The DPPH clearance rate of the lemons exposed to 0°C showed slight changes during storage, while significantly more intensive (P < .01) changes were evident at other temperatures. ) shows that storage time and storage temperature substantially affected the DPPH clearance rate, exhibiting the highest value when stored at 0°C for 10 d. After 6 d of storage, the DPPH clearance rate of samples stored at 0°C, 4°C, 7°C, and 10°C was 95.75%, 79.60%, 712%, and 58.17%, respectively. Significant differences were evident between the samples exposed to 0°C, 4°C, and 10°C (P < .01). ) shows the changes in the total soluble phenolic content of the lemons treated with the CTS-oil coating. When the fruits were stored at 7°C and 10°C for 6 d, the total phenolics content declined by 47.52%, and 51.65%, while the difference was not significant (P > .05). When stored for 10 d, the total phenolic content at 0°C, 4°C, and 7°C was 3.18 mg/g, 3.07 mg/g, and 2.40 mg/g. The differences between 4°C and 7°C were highly significant (P < .01), suggesting that the low temperature affected the total phenolic content in the lemons, while also inducing the phenolic accumulation after 10 d of storage.
Figure 4. Effects of different storage temperatures on DPPH radical scavenging activity (a) and TPC (b) of fresh-cut lemon.
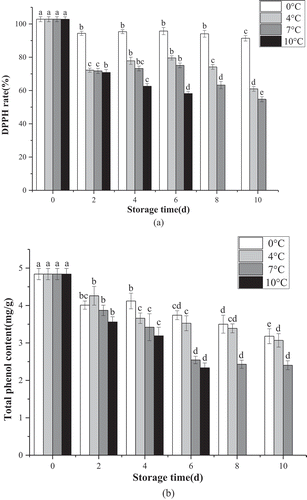
DPPH denotes the functional type and amount of bioactive compounds present. In this study, the DPPH free radical-scavenging activity was used to determine the changes in the antioxidant capacity changes of the freshly cut lemon slices, as well as to assess the potential antioxidant status of the tissue.[Citation52] Antioxidants can prevent or delay oxidation processes by reacting with free radicals and chelating metals, and by acting as oxygen scavengers in triplet, as well as singlet forms, and transferring hydrogen atoms to the free radical structure. Low temperatures increased the total soluble phenolic content of the lemons during the storage period, as evidenced by additional research. Siboza et al.[Citation53] suggested that the storage temperature affected the total phenolic content of the flavedo, while low-temperature conditions maintained an antioxidant pool of a specific size, extending the storage life of the lemons.
The phenolic acid in the lemons was detected using HPLC and included hydroxybenzoic acids such as protocatechuic acid, ρ-hydroxybenzoic acid, vanillic acid, guaiacol, ρ-coumaric acid, ferulic acid, and sinapic acid. Results showed that the cinnamic acid content was the highest during the beginning and end phases of the storage period, followed by benzoic acid, and guaiacol, while the catechin content was the lowest (Figure S2).
The coumaric acid content in the cinnamic phenolic acid was the highest at 10.01 μg/g before storage, and was significantly different at the four respective temperatures after 6 d of storage (P < .01). The p-coumaric acid content showed the most substantial decline of 8.73 μg/g after storage at 10°C for 6 d, while the cinnamic acid type erucic acid exhibited the lowest content level, having decreased by 25.5%, and 31.9%, respectively (P < .01) after 6 d of storage at 0°C and 10°C. No significant changes (P > .05) were evident between the content of the cinnamic acid type erucic acid stored at 4°C and 7°C following 6 d and 10 d of cold storage and were 0.68 μg/g, 0.67 μg/g, 0.63 μg/g, and 0.62 μg/g, respectively. After a storage period of 6 d, the ferulic acid content decreased by 3.65 μg/g, 28.5% (0°C), 39.5% (4°C), 42.0% (7°C), and 44.8% (10°C), respectively. After 10 d of storage, the ferulic acid content stored at 0°C, 4°C, and 7°C were 1.92 μg/g (0°C), 1.68 μg/g (4°C), and 1.52 μg/g, respectively. Furthermore, significant differences (P < .01) were evident between the samples stored at 0°C, 4°C, and 7°C. Therefore, 0°C was optimal in preventing the decrease of the cinnamon type phenolic acid content in the lemon peel, while temperatures of 4°C, and 7°C similar had a similar impact, and 10°C had the most unfavorable effect. Although phenolic benzoic acids displayed a lower antibacterial and antioxidant activity than cinnamic acids, they did influence enzymatic browning in freshly cut lemon slices.
The p-hydroxybenzoic acid and vanillic acid content in the lemon peel decreased gradually during storage, and of all the storage temperatures, displayed the most substantial decline of 51% and 34.4%, respectively at 10°C after 6 d. The initial value of the p-hydroxybenzoic acid content was 3.99 μg/g but exhibited an extremely significant difference (P < .01) after 6 d of being exposed to four different storage temperatures. The p-hydroxybenzoic acid content in the lemon peel was most significantly affected by a storage temperature of 10°C, displaying a value of 1.79 μg/g.
In most fruits and vegetables, guaiacol is the browning substrate of the POD, and its content represents the next major phenolic acid detected in the lemon peel, which was significantly impacted by the four storage temperatures. indicated that significant differences (P < .01) were evident between 0°C and 4°C, 7°C, and 10°C. The guaiacol content was reduced to 2.25 μg/g (0°C), 2.02 μg/g (4°C), and 1.95 μg/g (7°C) in the lemon peel that was stored for 10 d, but no significant differences (P > .05) were evident. Catechin, known as catechin acid, is a type of polyhydroxy phenolic acid. After exposure to different temperature conditions during storage, the catechin content in the lemon peel decreased from 1.40 μg/g to 1.01 μg/g after 6 d (0°C). Significant differences (P < .01) were apparent between the samples subjected to storage temperatures of 7°C and 10°C. After 10 d of storage at 0°C, 4°C, and 7°C, significant differences (P < .01) were evident at levels of 0.91 μg/g, 1.00 μg/g, and 0.81 μg/g, respectively.
Table 1. Effects of storage at different temperatures for 6 and 10 days on flavode phenolic acid types and content in fresh cut lemon slices.
As a result, the optimal storage conditions beneficial to phenolic acid content in the lemon peel were 0°C and 4°C. During storage, neither the p-coumaric acid nor erucic acid content displayed a significant decrease, while guaiacol and p-hydroxybenzoic acid exhibited a considerable decline. Putnik[Citation54] showed that fresh cut apple polyphenols and antioxidant capacity in stability at 4°C for the best. Wei et al.[Citation55] studied the polyphenol metabolism of freshly cut pineapple in cold storage conditions following ultrasonic treatment, and found that low-temperature storage provided higher stability to phenolic substances.
Effect of aroma components on Lemon Peel
A total of 52 volatile compounds were identified in the freshly cut lemon peel using GC/MS, which were only marginally less than the 54 types of volatile components evident in samples stored at 4°C for 5 d. The lemons stored at different temperatures were grouped according to the following chemical categories (Table S1): 20 terpenoids, 14 alcohols, eight esters, and seven aldehydes were identified. In addition, other compounds, including organic acids, alkanes, and ketones, were also detected.
The volatile components found in the lemon peel after storage at different temperatures are shown in . Of these components, alkenes, alcohols, aldehydes, and esters exhibited the most significant concentrations, representing between 70% ~ 97% of all the volatile compounds. Furthermore, the limonene and β-bisabolene content in the pericarp exceeded 50%, while geraniol and nerol, pine oil, alcohol, and bisabolol accounted for over 50% of the alcohols. Citral and 3,7-dimethyl-2,6-diene aldehyde denoted over 90% of the aldehyde compounds, while nerol acetate, and geranyl acetate ester compounds represented more than 90%, and cycloheptane accounted for over 65% of the alkane compounds. On day 6 of the storage process, the fruits that were kept at 4°C exhibited elevated levels of olefins, aldehydes, alkanes, and alkynes (Table S1). The main types of olefins that exhibited an increase were cyclohexene, octylene, longifolene, and pinene. The increased aldehydes were n-hexanal, isocyclocitral, β-pinene, limonene, trans-caryophyllene, 4-terpene alcohol, octyl alcohol, isophorol, 2-methyl-3-methylene-2-cycloheptane, 2-methylbutyric acid, α-pinene, and trans-orange tertiary alcohol. The vanillin, vanillin acetate, eugenol, guaiacol, eugene oxide, and (Z)-syringal all increased after storage, and similar results were observed by Huang et al.[Citation56] The percentages denoting the changes in the limonene and geranyl acetate content after storage were inconsistent with the findings of Tietel.[Citation57] These results could be attributed to the destruction of the essential oil cysts in the lemon peel after cutting, which was covered by a CTS composite coating to form a new protective film similar to the cell wall, resulting in increased evaporation and moisture loss during cryopreservation.
Table 2. The results of volatile components of lemon peel at different storage temperatures.
Geraniol is one of the main components responsible for the characteristic aroma of lemons,[Citation58] and presented the highest content after 6 d and 10 d of storage at 0°C, at 2.06 U and 1.92 U, respectively. There were no significant differences (P > .05) between the content percentages of the fresh lemon slices stored at 10°C and the other storage temperatures, indicating that the flavor of the freshly cut lemon slices stored at 10°C maintained an acceptable level. Significant differences (P < .01) were evident in the freshly cut lemon slices exposed to the four different storage temperatures of 0°C, 4°C, 7°C, and 10°C, indicating that the degree of volatilization of the β-bisabolene and neryl acetate decreased at low temperatures, but displayed a higher content level in the standard aroma components. With an increase in storage temperature and time, the β-myrrh content in the lemon peel decreased after a storage period of 6 d at 7°C, while the β-bisabolene content was higher than that observed initially at 7°C after 6 d, and then decreased after 10 d.
According to , the clove essential oil added to the film preservation liquid affected the aroma components in the peel of the freshly cut lemon slices after storage. Pathak et al.[Citation59] also reported that eugenol represented the primary active substance in eugenol essential oil. The results in showed that only eugenol was detected after 6 d of storage, while guaiacol was detected at 10 d, and all of them were more than 10%, suggesting that the clove essential oil in the compound film infiltrated the peel during storage, and the eugenol could be converted into guaiacol. However, the specific mechanism and conditions of the conversion still require further investigation.
Conclusion
CTS-oil coatings present a promising alternative treatment for enhancing the storage quality of fruits since it is environmentally safe and presents low toxicity to mammals. This study shows that the application of CTS-based coatings containing clove oil in low temperature (0°C) storage conditions is an excellent method for maintaining the qualitative properties and inhibiting the key enzyme activity responsible for the enzymatic browning of freshly cut lemons. After exposing the samples to four different storage temperatures for 10 d, they are treated with CTS-oil coatings at 0°C, which is responsible for the optimal preservation effect, as well as excellent sensory acceptability. The main volatile components in the lemon peel are optimal when stored at 4°C. After storage, the characteristic aroma components, such as limonene, β-pinene, and phellandrene, increase, while the content of γ-terpinol, linalool, 4-terpineol, citral and β-elemene decrease slightly. Furthermore, the phenolic acids are the same as initially observed, with guaiacol displaying the fastest decrease, and coumaric acid the slowest. The rate of decline of other pentaphenolic acids occur in the following descending order: ferulic acid > p-hydroxybenzoic acid > catechin > vanillin > erucic acid.
Supplemental Material
Download MS Word (11 MB)Disclosure statement
The author(s) declare(s) that there is no conflict of interests regarding the publication of this article.
Supplementary material
Supplemental data for this article can be accessed on publisher’s website.
Additional information
Funding
References
- Dong, X. Y.; Hu, Y.; Li, Y.; Zhou, Z. Q. The Maturity Degree, Phenolic Compounds and Antioxidant Activity of Eureka Lemon [Citrus Limon (L.) Burm. F.]: A Negative Correlation between Total Phenolic Content, Antioxidant Capacity and Soluble Solid Content. Sci. Hortic-Amsterdam. 2019, 243, 281–289. DOI: 10.1016/j.scienta.2018.08.036.
- Del Rıo, J. A.; Fuster, M. D.; Gómez, P.; Porras, I.; Garc ́ Ia-lidón, A.; Ortuño, A. Citrus Limon: A Source of Flavonoids of Pharmaceutical Interest. Food Chem. 2004, 84, 457–461. DOI: 10.1016/S0308-8146(03)00272-3.
- Gil-Izquierdo, A.; Riquelme, M. T.; Porras, I.; Ferreres, F. Effect of the Rootstock and Interstock Grafted in Lemon Tree (\r, Citrus Limon\r, (L.) Burm.) On the Flavonoid Content of Lemon Juice. J. Agric. Food Chem. 2004, 52, 324–331. DOI: 10.1021/jf0304775.
- González-Molina, E.; Domínguez-Perles, R.; Moreno, D. A.; García-Viguera, C. Natural Bioactive Compounds of Citrus Limon for Food and Health. J. Pharm. Biomed. Anal. 2010, 51, 327–345. DOI: 10.1016/j.jpba.2009.07.027.
- Zahid, N.; Ali, A.; Siddiqui, Y.; Maqbool, M. Efficacy of Ethanolic Extract of Propolis in Maintaining Postharvest Quality of Dragon Fruit during Storage. Postharvest Biol. Technol. 2013, 79, 69–72. DOI: 10.1016/j.postharvbio.2013.01.003.
- Diab, T.; Biliaderis, C. G.; Gerasopoulos, D.; Sfakiotakis, E. Physicochemical Properties and Application of Pullulan Edible Films and Coatings in Fruit Preservation. J. Sci. Food Agric. 2001, 81, 988–1000. DOI: 10.1002/jsfa.883.
- Muzzarelli, R. A. A.; Boudrant, J.; Meyer, D.; Manno, N.; Demarchis, M.; Paoletti, M. G. Current Views on Fungal Chitin/chitosan, Human Chitinases, Food Preservation, Glucans, Pectins and Inulin: A Tribute to Henri Braconnot, Precursor of the Carbohydrate Polymers Science, on the Chitin Bicentennial. Carbohydr. Polym. 2012, 87, 995–1012. DOI: 10.1016/j.carbpol.2011.09.063.
- Terry, L. A.; Joyce, D. C. Elicitors of Induced Disease Resistance in Postharvest Horticultural Crops: A Brief Review. Postharvest Biol. Tec. 2004, 32, 1–13. DOI: 10.1016/j.postharvbio.2003.09.016.
- Li, H.; Yu, T. Effect of Chitosan on Incidence of Brown Rot, Quality and Physiological Attributes of Postharvest Peach Fruit. J. Sci. Food Agric. 2001, 81, 269–274. DOI: 10.1002/1097-0010(20010115)81:2<269::AID-JSFA806>3.0.CO;2-F.
- Abbasi, N. A.; Iqbal, Z.; Maqbool, M.; Hafiz, I. A. Postharvest Quality of Mango (Mangifera Indica L.) Fruit as Affected by Chitosan Coating. Pakistan. J. Bot. 2009, 41, 343–357.
- Badawy, M. E. I.; Rabea, E. I. Potential of the Biopolymer Chitosan with Different Molecular Weights to Control Postharvest Gray Mold of Tomato Fruit. Postharvest Biol. Tec. 2009, 51, 110–117. DOI: 10.1016/j.postharvbio.2008.05.018.
- Xing, Y.; Li, X. H.; Xu, Q. L.; Yun, J.; Lu, Y. Q.; Tang, Y. Effects of Chitosan Coating Enriched with Cinnamon Oil on Qualitative Properties of Sweet Pepper (Capsicum Annuum L.). Food Chem. 2011, 124, 1443–1450. DOI: 10.1016/j.foodchem.2010.07.105.
- Song, H. W.; Yuan, W. M.; Jin, P.; Wang, W.; Wang, X. F.; Yang, L. M.; Zhang, Y. F. Effects of Chitosan/nano-silica on Postharvest Quality and Antioxidant Capacity of Loquat Fruit during Cold Storage. Postharvest Biol. Tec. 2016, 119, 41–48. DOI: 10.1016/j.postharvbio.2016.04.015.
- Isman, M. B.;. Plant Essential Oils for Pest and Disease Management. Crop. Prot. 2000, 19, 0–608. DOI: 10.1016/S0261-2194(00)00079-X.
- Kalemba, D.; Kunicka, A. Antibacterial and Antifungal Properties of Essential Oils. Curr. Med. Chem. 2003, 10, 813–829. DOI: 10.2174/0929867033457719.
- Burt, S. A.;. Essential Oils: Their Antibacterial Properties and Potential Applications in Foods- a Review. Int. J. Food Microbiol. 2004, 4, 233–253.
- Sivakumar, D.; Bautista-Banos, S. A Review on the Use of Essential Oils for Postharvest Decay Control and Maintenance of Fruit Quality during Storage. Crop. Prot. 2014, 64, 27–37. DOI: 10.1016/j.cropro.2014.05.012.
- Rojas-Graü, M. A.; Soliva-Fortuny, R.; Martín-Belloso, O. Edible Coatings to Incorporate Active Ingredients to Fresh-cut Fruits: A Review. Trends Food Sci. Tech. 2009, 20, 438–447. DOI: 10.1016/j.tifs.2009.05.002.
- Xiao, C. L.; Luo, W.; Liu, M. Y.; Zhu, L. W.; Li, M.; Yang, H. S.; Deng, Y. Quality of Fresh-cut Pears (Pyrus Bretschneideri Rehd Cv. Huangguan) Coated with Chitosan Combined with Ascorbic Acid and Rosemary Extracts. Philipp. Agric. Sci. 2010, 93, 66–75.
- Perdones, A.; Escriche, I.; Chiralt, A.; Vargas, M. Effect of Chitosan–lemon Essential Oil Coatings on Volatile Profile of Strawberries during Storage. Food Chem. 2016, 197, 979–986. DOI: 10.1016/j.foodchem.2015.11.054.
- Xing, Y. G.; Li, X. H.; Xu, Q. L.; Yun, J.; Lu, Y. Q. Antifungal Activities of Cinnamon Oil against Rhizopus Nigricans, Aspergillus Flavus and Penicillium Expansum in Vitroand in Vivo Fruit Test. Int. J. Food. Sci. Tech. 2010, 45, 1837–1842. DOI: 10.1111/j.1365-2621.2010.02342.x.
- Hosseini, S. F.; Amraie, M.; Salehi, M.; Mohseni, M.; Aloui, H. Effect of Chitosan-based Coatings Enriched with Savory And/or Tarragon Essential Oils on Postharvest Maintenance of Kumquat (Fortunella Sp.) Fruit. Food. Sci. Nutr. 2018, 7, 155–162. DOI: 10.1002/fsn3.835.
- Xu, Q. L.; Xing, Y. G.; Che, Z. M.; Guan, T. W.; Zhang, L.; Bai, Y. M.; Gong, L. Effect of Chitosan Coating and Oil Fumigation on the Microbiological and Quality Safety of Fresh-cut Pear. J. Food Safety. 2013, 33, 179–189. DOI: 10.1111/jfs.12038.
- Xing, Y. G.; Li, X. H.; Xu, Q. L.; Yun, J.; Lu, Y. Q. Extending the Shelf Life of Fresh-cut Lotus Root with Antibrowning Agents, Cinnamon Oil Fumigation and Moderate Vacuum Packaging. J. Food Process. Eng. 2012, 35, 505–521. DOI: 10.1111/j.1745-4530.2010.00604.x.
- Saki, M.; Valizadehkaji, B.; Abbasifar, A.; Shahrjerdi, I. Effect of Chitosan Coating Combined with Thymol Essential Oil on Physicochemical and Qualitative Properties of Fresh Fig (Ficus Carica L.) Fruit during Cold Storage. J. Food Meas. Charact. 2019, 13, 1147–1158. DOI: 10.1007/s11694-019-00030-w.
- Xu, Q. L.; Li, S. H..; Shui, Y. R.; Yang, H.; Li, X. L.; Lin, H. B.; Xing, Y. G. Optimized Formulation of Chitosan Compound Preservative Coating for Fresh-cut Lemon. Food Ferment. Ind. 2019, 45, 166–172. (in Chinese).
- Odriozola-Serrano, I.; Hernández-Jover, T.; Martín-Belloso, O. Comparative Evaluation of Uv-hplc Methods and Reducing Agents to Determine Vitamin C in Fruits. Food Chem. 2007, 105, 1151–1158. DOI: 10.1016/j.foodchem.2007.02.037.
- Kumar, P.; Sethi, S.; Sharma, R. R.; Srivastav, M.; Varghese, E. Effect of Chitosan Coating on Postharvest Life and Quality of Plum during Storage at Low Temperature. Sci. Hortic-Amsterdam 2017, 226, 104–109. DOI: 10.1016/j.scienta.2017.08.037.
- Scherer, R.; Rybka, A. C. P.; Ballus, C. A.; Meinhart, A. D.; Teixeira, J.; Godoy, H. T. Validation of a HPLC Method for Simultaneous Determination of Main Organic Acids in Fruits and Juices. Food Chem. 2012, 135, 150–154. DOI: 10.1016/j.foodchem.2012.03.111.
- Hu, J. Q.; Yang, L. Z.; Wu, W. J.; Li, Y.; Zhan, L. J. Slicing Increases Antioxidant Capacity of Fresh-cut Lotus Root (\r, Nelumbo Nucifera\r, G.) Slices by Accumulating Total Phenols. Int. J. Food. Sci. Tech. 2014, 49, 2418–2424. DOI: 10.1111/ijfs.12563.
- Rasouli, M.; Koushesh Saba, M.; Ramezanian, A. Inhibitory Effect of Salicylic Acid and Aloe Vera Gel Edible Coating on Microbial Load and Chilling Injury of Orange Fruit. Sci. Hortic-Amsterdam 2019, 247, 27–34. DOI: 10.1016/j.scienta.2018.12.004.
- Huang, Q. H.; Qian, X. C.; Jiang, T. J.; Zheng, X. L. Effect of Chitosan and Guar Gum Based Composite Edible Coating on Quality of Mushroom (Lentinus Edodes) during Postharvest Storage. Sci. Hortic-Amsterdam 2019, 253, 382–389. DOI: 10.1016/j.scienta.2019.04.062.
- Xing, Y. G.; Li, X. H.; Xu, Q. L.; Jiang, Y. H.; Yun, J.; Li, W. L. Effects of Chitosan-based Coating and Modified Atmosphere Packaging (MAP) on Browning and Shelf Life of Fresh-cut Lotus Root (Nelumbo Nucifera Gaerth). Innov. Food. Sci. Emerg. 2010, 11, 684–689. DOI: 10.1016/j.ifset.2010.07.006.
- Serradell, M.; Rozenfeld, P. A. Polyphenoloxidase Activity from Strawberry Fruit (Fragaria X Ananassa, Duch, Cv Selva): Characterisation and Partial Purification. J. Sci. Food Agric. 2000, 80, 1421–1427. DOI: 10.1002/1097-0010(200007)80:9<1421::AID-JSFA649>3.0.CO;2-K.
- Wang, Y.; Yang, Z. M.; Zhang, Q. F.; Li, J. L. Enhanced Chilling Tolerance Inzoysia Matrellaby Pre-treatment with Salicylic Acid, Calcium Chloride, Hydrogen Peroxide or 6-benzylaminopurine. Biol. Plantarum. 2009, 53, 179–182. DOI: 10.1007/s10535-009-0030-2.
- Zhang, J. H.; Huang, W. D. Improvement of Chilling Tolerance and Accumulation of Small Heat Shock Proteins (Shsp17.6) In Grape Berry by Heat-pretreatment. Acta Agric. Boreali-Sinica. 2006, 21, 1–5.
- Elfalleh, W.; Nasri, N.; Marzougui, N.; Thabti, I.; M’rabet, A.; Yahya, Y.; Lachiheb, B.; Guasmi, F.; Ferchichi, A. Physico-chemical Properties and DPPH-ABTS Scavenging Activity of Some Local Pomegranate (Punica Granatum) Ecotypes. Int. J. Food. Sci. Nutr. 2009, 60, 197–210. DOI: 10.1080/09637480903067037.
- Gorinstein, S.; Haruenkit, R.; Park, Y. S.; Jung, S. T.; Zachwieja, Z.; Jastrzebski, Z.; Katrich., E.; Trakhtenberg, S.; Belloso., O. M. Bioactive Compounds and Antioxidant Potential in Fresh and Dried Jaffa Sweeties, a New Kind of Citrus Fruit. J. Sci. Food Agric. 2004, 84, 1459–1463. DOI: 10.1002/jsfa.1800.
- Du, G. R.; Li, M. J.; Ma, F. W.; Liang, D. Antioxidant Capacity and the Relationship with Polyphenol and Vitamin C in Actinidia Fruits. Food Chem. 2009, 113, 557–562. DOI: 10.1016/j.foodchem.2008.08.025.
- Qiao, Y.; Xie, B. J.; Zhang, Y.; Zhang, Y.; Fan, G.; Yao, X. L.; Pan, S. Y. Characterization of Aroma Active Compounds in Fruit Juice and Peel Oil of Jinchen Sweet Orange Fruit (Citrus Sinensis (L.) Osbeck) by GC-MS and GC-O. Molecules 2008, 13, 1333–1344. DOI: 10.3390/molecules13061333.
- Bico, S. L. S.; Raposo, M. F. J.; Morais, R. M. S. C.; Morais, A. M. M. B. Combined Effects of Chemical Dip And/or Carrageenan Coating And/or Controlled Atmosphere on Quality of Fresh-cut Banana. Food Control 2009, 20, 508–514. DOI: 10.1016/j.foodcont.2008.07.017.
- Hong, K. Q.; Xu, H. B.; Wang, J. N.; Zhang, L. B.; Hu, H. G.; Jia, Z. W.; Gu, H.; He, Q. G.; Gong, D. Q. Quality Changes and Internal Browning Developments of Summer Pineapple Fruit during Storage at Different Temperatures. Sci. Hortic-Amsterdam 2013, 151, 68–74. DOI: 10.1016/j.scienta.2012.12.016.
- Simões, A. D. N.; Tudela, J. A.; Allende, A.; Puschmann, R.; Gil, M. I. Edible Coatings Containing Chitosan and Moderate Modified Atmospheres Maintain Quality and Enhance Phytochemicals of Carrot Sticks. Postharvest Biol. Tec. 2009, 51, 364–370. DOI: 10.1016/j.postharvbio.2008.08.012.
- Hong, K. Q.; Xie, J. H.; Zhang, L. B.; Sun, D. Q.; Gong, D. Q. Effects of Chitosan Coating on Postharvest Life and Quality of Guava (Psidium Guajava L.) Fruit during Cold Storage. Sci. Hortic-Amsterdam 2012, 144, 172–178. DOI: 10.1016/j.scienta.2012.07.002.
- Ayranci, E.; Tunc, S. The Effect of Edible Coatings on Water and Vitamin C Loss of Apricots (Armeniaca Vulgaris Lam.) And Green Peppers (Capsicum Annuum L.). Food Chem. 2004, 87, 339–342. DOI: 10.1016/j.foodchem.2003.12.003.
- Min, T.; Xie, J.; Zheng, M. L.; Yi, Y.; Hou, W. F.; Wang, L. M.; Ai, Y. W.; Wang, H. X. The Effect of Different Temperatures on Browning Incidence and Phenol Compound Metabolism in Fresh-cut Lotus (Nelumbo Nucifera G.) Root. Postharvest Biol. Tec. 2017, 123, 69–76. DOI: 10.1016/j.postharvbio.2016.08.011.
- Liu, H.; Jiang, W. B.; Cao, J. K.; Li, Y. C. Effect of Chilling Temperatures on Physiological Properties, Phenolic Metabolism and Antioxidant Level Accompanying Pulp Browning of Peach during Cold Storage. Sci. Hortic-Amsterdam 2019, 255, 175–182. DOI: 10.1016/j.scienta.2019.05.037.
- Jhalegar, J.; Sharma, R. R.; Singh, D. In Vitro and in Vivo Activity of Essential Oils against Major Postharvest Pathogens of Kinnow (Citrus Nobilis X C. Deliciosa) Mandarin. J. Food. Sci. Tech. 2015, 52, 2229–2237. DOI: 10.1007/s13197-014-1281-2.
- Shi, J. Y.; Zuo, J. H.; Zhou, F. H.; Gao, L. P.; Wang, Q.; Jiang, A. L. Low-temperature Conditioning Enhances Chilling Tolerance and Reduces Damage in Cold-stored Eggplant (Solanum Melongena L.) Fruit. Postharvest Biol. Tec. 2018, 141, 33–38. DOI: 10.1016/j.postharvbio.2018.03.007.
- Xu, W. T.; Peng, X. L.; Luo, Y. B.; Wang, J. A.; Guo, X.; Huang, K. L. Physiological and Biochemical Responses of Grapefruit Seed Extract Dip on ‘Redglobe’ Grape. LWT-Food Sci. Technol. 2009, 42, 471–476. DOI: 10.1016/j.lwt.2008.09.002.
- Chen, Q. M.; Wang, Q. G.; Fu, M. R. Postharvest Low Temperature Conditioning Reduces Peel Browning and Improves Fruit Quality in Storage and Subsequent Shelf Life of Huangguan Pear. Food Nutr. Sci. 2015, 06, 1351–1361. DOI: 10.4236/fns.2015.615141.
- Robles-Sánchez, R. M.; Rojas-Graü, M. A.; Odriozola-Serrano, I.; González-Aguilar, G.; Martin-Belloso, O. Influence of Alginate-based Edible Coating as Carrier of Antibrowning Agents on Bioactive Compounds and Antioxidant Activity in Fresh-cut Kent Mangoes. LWT-Food Sci. Technol. 2013, 50, 240–246. DOI: 10.1016/j.lwt.2012.05.021.
- Siboza, X. I.; Bertling, I.; Odindo, A. O. Salicylic Acid and Methyl Jasmonate Improve Chilling Tolerance in Cold-stored Lemon Fruit (Citrus Limon). J. Plant Physiol. 2014, 171, 1722–1731. DOI: 10.1016/j.jplph.2014.05.012.
- Putnik, P.; Bursać Kovačević, D.; Herceg, K.; Pavkov, I.; Zorić, Z.; Levaj, B. Effects of Modified Atmosphere, Anti-browning Treatments and Ultrasound on the Polyphenolic Stability, Antioxidant Capacity and Microbial Growth in Fresh-cut Apples. J. Food Process. Eng. 2017, 40, e12539.1-e12539.12. DOI: 10.1111/jfpe.12539.
- Yeoh, W. K.; Ali, A. Ultrasound Treatment on Phenolic Metabolism and Antioxidant Capacity of Fresh-cut Pineapple during Cold Storage. Food Chem. 2017, 216, 247–253. DOI: 10.1016/j.foodchem.2016.07.074.
- Huang, M. Y.; Valim, M. F.; Feng, S.; Reuss, L.; Yao, L. X.; Gmitter, F.; Wang, Y. Characterization of the Major Aroma-Active Compounds in Peel Oil of an HLB-Tolerant Mandarin Hybrid Using Aroma Extraction Dilution Analysis and Gas Chromatography-Mass Spectrometry/Olfactometry. Chemosens. Percept. 2017, 10, 161–169. DOI: 10.1007/s12078-017-9221-y.
- Tietel, Z.; Bar, E.; Lewinsohn, E.; Feldmesser, E.; Fallik, E.; Proat, R. Effects of Wax Coatings and Postharvest Storage on Sensory Quality and Aroma Volatile Composition of ‘Mor’ Mandarins. J. Sci. Food Agric. 2010, 90, 995–1007. DOI: 10.1002/jsfa.3909.
- Lota, M. L.; Serra, D. D.; Tomi, F.; Jacquemond, C.; Casanova, J. Volatile Components of Peel and Leaf Oils of Lemon and Lime Species. J. Agric. Food Chem. 2002, 50, 796–805. DOI: 10.1021/jf010924l.
- Pathak, S. B.; Niranjan, K.; Padh, H.; Rajani, M. TLC Densitometric Method for the Quantification of Eugenol and Gallic Acid in Clove. Chromatographia 2004, 60, 241–244. DOI: 10.1365/s10337-004-0373-y.