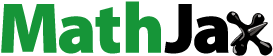
ABSTRACT
To investigate the biosynthesis of silver nanoparticles (T-AgNPs) using leaf extract of Tamarix articulata and their possible role in attenuation of oxidative stress mediated human diseases. T-AgNPs were characterized by several biophysical techniques. Various health beneficial activities were also investigated. The synthesized T-AgNPs showed an absorption peak at 413 nm on UV-Visible spectroscopy indicating the surface plasmon resonance of the nanoparticles. TEM analysis clearly showed that particles were spherical with an average size of about 25 nm. SEM image analysis confirmed the spherical shape of T-AgNPs. T-AgNPs (600 µg/ml) showed effective DPPH scavenging (68.23%), ferric reducing (68.23%), and hydrogen peroxide reducing (70.09%) activities. Besides, T-AgNPs at 600 µg/ml, also showed strong anti-glycating, and AGEs formation inhibitory activities as indicated by reduced browning intensity (51.62%), aggregation index (48.86%), and cross amyloid structures (53.62%) as compared to positive controls (100%). A 600 µg/ml of T-AgNPs was found to have potent in vitro anti-inflammatory activity as revealed by albumin denaturation inhibition (73.19%), protease activity inhibition (70.196%), membrane stability against heat (74.16%), and hyposaline (72.98%) induced hemolysis as compared to controls. Altogether, these findings confirm that T-AgNPs can be promising candidates for the development of antioxidant, anti-inflammatory, membrane stabilizing, antiglycating and AGEs inhibitory agents.
Introduction
Biosynthesis of nanoparticles is a rapidly emerging research field of science because of their wide range of applications.[Citation1,Citation2] which involves synthesis and characterization of nanoparticles synthesized from metals. Nanoparticles are increasingly being important in wide range of sectors: drug delivery, bioengineering, textile engineering, biological labeling, biotechnology, catalysis, water treatment, and the detection of genetic disorders.[Citation3] The particles having a size of 100 nm or lesser than this dimension are considered as nanoparticles. Nanoparticles including silver, platinum and gold are widely applied in medical applications. Nanoparticles have been synthesized by many methods like chemical, physical, and biological methods. In chemical synthesis, many environmentally toxic or biologically hazardous substances are used as reducing agents.[Citation4] Therefore, cost-effective and eco-friendly biological methods are more concerned for nanoparticle synthesis. These days, various biological systems including plant extracts, algae, lichen, bacteria, fungi, and yeast are used for the biosynthesis of metal nanoparticles.
Silver is the important noble metal which shows significant disinfecting, antimicrobial properties and tremendous medicinal values.[Citation5] Silver nanoparticles (AgNps) possess some unique characteristic features like large surface area, high dispersion, and smaller size that contribute to their strong biomedical properties like antimicrobial, anticancer, antidiabetic, antioxidant, and anti-inflammatory activities.[Citation6] The synthesis of silver nanoparticles (AgNPs) with valuable biological properties can be considered to be very significant for various scientific products.[Citation7] Thus, the green synthesis of AgNps for their potent antioxidant, anti-inflammatory, and antimicrobial activities is a main subject of research. Plant extracts have been utilized for the biosynthesis of AgNps due to their advantages on chemical synthesis. In this context, certain plants who are rich in antioxidant compounds have been considered to be better reducing agents in the synthesis of AgNps. Besides, different proteins present in plant extracts have been suggested to be involved in reduction of silver ions.[Citation8] Plant extracts are safe, nontoxic, and readily available. In addition, many valuable phyto-constituents are present in plant extracts that can be helpful in reduction of silver ions.[Citation9] AgNps have been synthesized from diverse parts of plant body.[Citation10] Silver nanoparticles synthesized from different sources have been reported to have sound antibacterial activity.[Citation11–13]
The use of medicinal plant in treatments of various diseases is a distinctive feature of Islam. However, the search for plant derived products for treatment of various health disorders is still continued worldwide.[Citation14] Natural products are less expensive, more effective, and safe.[Citation15] Several types of medicinal plants have been recognized that are used for prevention and treatment of diseases such as curcuma[Citation16] and green tea.[Citation17] Tamarix articulate is a moderate sized halophytic tree with feathery foliage and belongs to Tamaricaece family. Tamarix is very commonly found in unfavorable environmental conditions of Saudi Arabia deserts.[Citation18] One of these plants has been reported to be a rich source of several appreciated phytochemicals.[Citation19] Tamarix has been used in folk medicines for curing of wounds, jaundice, abscesses, bad evils, and rheumatism. Further, the wood of Tamarix is used for timber, and fuel. The leaves of Tamarix are used as a fodder for camels. Tamarix is very important from religious point of view because it has been mentioned in Ahadith also.[Citation14,Citation20]
Salt stress is the prime cause leading to the increased rate of the production and the accumulation of reactive oxygen species (ROS).[Citation21] Oxidative stress mediated by enhanced production and accumulation of ROS lead to damage of various biomolecules, and membranes and tissue injuries.[Citation22] Halophytes are equipped with capability to withstand harsh conditions of salt stress because of counteracting the damaging effects of ROS. Thus, halophytes are known to have excellent antioxidant defense system including enzymatic as well as non-enzymatic systems.[Citation21] Superoxide dismutase is an important antioxidant defense molecule.[Citation23,Citation24] Antioxidant activity of plants may be very significant in prevention of oxidative stress induced damage of biomolecules and hence, in various health disorders.[Citation22]
In the current study, Tamarix leaves extract-mediated AgNps (T-AgNps) were biosynthesized, and were characterized by using UV-visible spectrophotometer, transmission electron microscope (TEM), and scanning electron microscopy (SEM). In addition, the biosynthesized T-AgNps were analyzed for its biochemical activities such as antioxidant, anti-inflammatory, antiglycating, AGEs formation inhibitory potentials, and antimicrobial property. Further, we compared these properties with that of Tamarix ethanolic extract.
Material and methods
Materials
Folin-Ciocalteau reagent, Congo red ferric chloride, potassium ferricyanide, 2,2-diphenyl-1-picrylhydrazyl (DPPH), gallic acid, silver nitrate, trichloroacetic acid, ascorbic acid, quercetin, and trypsin were purchased from Sigma Co. St. Louis, Missouri, USA. DMSO, hydrochloric acid, mono sodium dihydrogen phosphate, disodium hydrogen phosphate, aluminum chloride, sodium carbonate, hydrogen peroxide, methanol, and sodium hydroxide were purchased from Merck, Darmstadt, Germany. All the chemicals and reagents were of analytical grade. The solvents used were of HPLC grade.
Preparation of ethanolic extract of Tamarix leaves
The leaves () of Tamarix articulata were collected from Buraydah, Qassim, Saudi Arabia. After authentication by botanist, the leaves were separated from each other, properly prewashed with water, and set to air-dry under shade at room temperature for 3 days. All leaves were grounded by using a basic electric grinder. The resulting homogenous powder was first completely dried under shade. The dry powder (100 g) was extracted by maceration of ethanol (500 ml) for 5 days. Precautions were taken so that potential phytochemicals of Tamarix leaves were not lost during extraction. Later, Whatman filter paper No.1 (Whatman Ltd., England) was used to filter the extract. The filtrate was collected, 10 ml of extract was collected for synthesis of T-AgNPs. Remaining extract was kept in a vacuum and resulting dried extract was stored in tightly closed flasks in cold for subsequent analysis. The filtrate was reconstituted in the desired amount of DMSO, methanol, or distill water according to experimental needs.
Phytochemical screening of Tamarix leaf extract
Chemical tests were carried out to identify the constituents as described by Odebiyi and Sofowora.[Citation25] For the test for carbohydrate, 1 ml of iodine solution was added to 3 ml Tamarix ethanolic leaf extract. The possible appearance of purple color may indicate the presence of carbohydrates. The test for saponins was performed by adding 5 ml of distill water to 5 leaf extract and was shaken vigorously in a test tube. Later, the test tube was warmed. The possible formation of stable foam may indicate the presence of saponins. However, about 2 ml of leaf extract was added to 2 ml distill water and was stirred. Few drops of FeCl3 were included in the solution. The presence of green precipitate showed the presence of tannins. The presence of flavonoids in leaves extract was indicated by appearance of the intense yellow color by treating the extract (2 ml) with 20% sodium hydroxide solution. The yellow color becomes turns colorless after addition of dilute HCl. The formation of bluish black color by treating of extract with few drops of FeCl3, confirmed the formation of phenolic compounds.
Estimation of total phenol content in leaf extract
Folin-Ciocalteu spectrophotometric method was used to estimate the total phenolic content present in Tamarix leaves extract with little modifications.[Citation22] A calibration curve was generated by using gallic acid as a reference standard and total phenolic content was calculated by using the linear equation of the gallic acid standard curve. All tests were performed in triplicates and the results were expressed as mg of gallic acid equivalents (GAE) per g of dry weight of leaf extract. Following formula was used to calculate total phenolic content (TPC).
TPC = Volume × Concentration/Weight of pure dried sample
where Z is the concentration of gallic acid (mg/ml); V is the volume (ml) of sample used in the extraction; m is the weight of pure dried sample used (g).
Estimation of total flavonoid content in Tamarix leaf extract
Estimation of total flavonoid content in the Tamarix leaf extract was carried out using aluminum chloride (AlCl3) colorimetric method.[Citation26] A standard calibration curve was prepared by using quercetin (20–250 µg/ml). In brief, 500 µl of tamarix leaf extract (50 µg/ml) or standard quercetin solution was added to 500 µl of AlCl3 (2%). The reaction mixture was shaken intermittently. After 1 h at room temperature, the absorbance was measured at 430 nm using a spectrophotometer against methanol as blank. The total flavonoid content was calculated as quercetin equivalent (mg/g) (mg QUE/g) by the following equation to estimate the total flavonoid content (TFC).
TFC = Z × V/m
where Z is concentration of quercetin (mg/ml); V is volume (ml) of sample used in the extraction; m is the weight of pure dried sample used (g).
Bio-inspired synthesis of T-AgNps
Bio-inspired synthesis of T-AgNps was started by mixing of 1 ml ethanolic extract (obtained after filtration by Whatman filter paper) with 30 ml of 1 mM silver nitrate (AgNO3) with the use of continuous stirring, and 35°C. The transformation of colorless suspension to dark brown color indicated the formation of T-AgNPs by reduction of AgNO3. Later, the suspension was subjected to centrifugation at 10000 rpm for 25 min to separate biosynthesized T-AgNps from the solution. The pellet was washed several times and was dried overnight at 65°C .[Citation27] Schematic presentation of silver nanoparticles is given in .
Characterization of T-AgNps by UV-Visible spectroscopic characterization
Color change to brown is considered to be a characteristic feature of silver nanoparticle synthesis. The optical properties of T-AgNps were studied using UV-visible spectral analysis in 200–700 nm wavelength.
Transmission electron microscopy (TEM) analysis
TEM (JEOL 1010) was used to find the structure and size distribution of T-AgNps. For this purpose, drops of T-AgNps suspension were made in double distilled water, and these drops were sonicated to make an even dispersion. A small drop (10 µm) of silver nanoparticle suspension was directly applied to formvar coated grids. The sample was allowed to stand for 2 min, and a blotting paper was used to remove the extra sample. The sample was left to dry under vacuum. Later, the thin film was kept in the grid box sequentially for TEM analysis.
Scanning electron microscopy (SEM) analysis
The morphology, shape, and size of T-AgNps were accomplished by SEM (JEOL-5500). Thin films of samples were prepared by simply dropping a very small amount of T-AgNps on double sided carbon tape which was fixed to the stub, excess solution was removed by using blotting paper. The film was allowed to dry under vacuum, and it was coated with platinum on a sputter coating machine (JEOL JFC1300) and examined under SEM.
Investigation of reducing ability of T-AgNps and Tamarix leaf powder extract using ferric reducing antioxidant power
The capacity of T-AgNps and Tamarix leaf powder extract to reduce Fe3+ was assessed by ferric reducing antioxidant power (FRAP) method according to our previously published method.[Citation22] Ferric reducing antioxidant power (FRAP) assay was carried out in the presence of either T-AgNps or Tamarix leaf extract (100, 200, 300, 400, 500, 600 µg/ml). The absorbance of standard and test samples were measured at 700 nm. Blank solution had phosphate buffer (pH 6.6) only. Higher absorbance denotes to higher reducing power. Ascorbic acid was used as standard and all the samples were run in triplicate.
Percent reducing power = [(Abscontrol – Abssample)/Abscontrol] × 100
where Abscontrol = Absorbance of control.
Abssample = Absorbance in the presence of extract or T-AgNps.
Evaluation of hydrogen peroxide (H2O2) reducing ability
H2O2 reducing ability of T-AgNps and Tamarix leaf extract (100, 200, 300, 400, 500, 600 µg/ml) was investigated to explore their antioxidant capacity using according to our previously published protocol.[Citation22] The absorbance of H2O2 was measured using a UV visible spectrophotometer at a constant wavelength, i.e., 230 nm against a blank solution having phosphate buffer without H2O2. The experiment was performed in triplicates. The percentage of scavenged H2O2 was calculated using the following equation.
Percentage of scavenged H2O2 = [(Abscontrol – Abssample)/Abscontrol] × 100
where Abscontrol = Absorbance of control.
Abssample = Absorbance in the presence of extract or T-AgNps.
Evaluation of free radical scavenging activity by DPPH method
The ability to scavenge 1,1 difenyl-2-picryl-hydrazyl (DPPH) was determined according to our previously published articles with minor modifications.[Citation22,Citation28,Citation29] A 2.5 ml of various dilutions of T-AgNps or extract (100, 200, 300, 400, 500, 600 μg/ml) prepared in methanol was mixed with 1 ml of 0.3 mM methanolic solution of DPPH. After 30 min incubation in darkness and at room temperature, the resultant absorbance was recorded at 517 nm against methanol as a blank. The control was prepared by using methanol instead of extract. The percentage inhibition was calculated in accordance with the following equation.
Percent inhibition = [(Abscontrol – Abssample)/Abscontrol] × 100
where Abscontrol = Absorbance of control.
Abssample = Absorbance in the presence of extract or T-AgNps.
Albumin denaturation inhibition- An evaluation of anti-inflammatory activity
Albumin denaturation inhibition activity was employed to explore the possible mechanism of anti-inflammatory action of both T-AgNps and Tamarix extract according to previously published articles[Citation22,Citation30] Ibuprofen is a powerful non-steroidal anti-inflammatory drug that was used as a reference drug. Different sterile tubes having 1% aqueous solution of bovine serum albumin (BSA) (500 μl) and 100 μl of various aliquots of T-AgNps and Tamarix extract (100, 200, 300, 400, 500, 600 µg/ml) or ibuprofen (200 µg/ml) were incubated at 37°C for 20 min. After incubation, the tubes were kept in a water bath at 71°C for 30 min to induce the denaturation of BSA. Later, the tubes were cooled down, and the absorbance of samples was measured by a UV visible spectrophotometer at 660 nm against blank having distill water only. The experiment was run in triplicates and the average of absorbance was taken. The experiment was carried out in triplicate. The percentage inhibition of protein denaturation was calculated by using the following formula
Percentage Inhibition = [(Abscontrol – Abssample)/Abssample] × 100
where Abscontrol = Absorbance of control; Abssample = Absorbance in the presence of extract or T-AgNPs or ibuprofen
Inhibition of proteinase action
The mechanism of anti-inflammatory activity of both T-AgNps and Tamarix extract was further assessed by proteinase enzyme inhibitory activity.[Citation22,Citation31] In this test, 2.0 ml of reaction mixture contained 0.06 mg trypsin, 1.0 ml Tris-HCl buffer (20 mM, pH 7.4), and 1.0 ml of sample (100, 200, 300, 400, 500, 600 μg/ml) of either T-AgNps, Tamarix extract or diclofenac sodium (200 μg/ml). The reaction mixtures were incubated at 37oC for 5 min. Then, 1 ml of 0.8% (w/v) casein was included in various reaction mixtures. The mixtures were incubated for an additional 20 min. 2 ml of perchloric acid (70%) was added to terminate the reaction. The cloudy suspension was centrifuged for 5 min at 2500 rpm and the absorbance of resultant supernatant was read at 210 nm against buffer as blank. The experiment was done in triplicate. The percentage inhibition of proteinase activity was calculated.
Percentage Inhibition = [(Abscontrol – Abssample)/Abssample] × 100
where Abscontrol = Absorbance of control; Abssample = Absorbance in the presence of T-AgNPs or extract or diclofenac sodium.
Egg albumin denaturation inhibition
The mechanism of anti-arthritic activity of both T-AgNps and Tamarix extract was confirmed by inhibition of heat-induced egg albumin denaturation. For this test, different test tubes having 0.2 ml of fresh hen’s egg albumin, phosphate buffer of pH 6.4 (2.8 ml), and 2 ml of various concentrations (100, 200, 300, 400, 500, 600 µg/ml) of T-AgNps, Tamarix extract, or standard drug (diclofenac sodium) were incubated for 15 min at room temperature. After completion of incubation, the test tubes were kept at 70°C in a water bath for 5 min, and were cooled down under running tap water. The absorbance of different test mixtures was read at 660 nm using a UV visible spectrophotometer against buffer as blank. The inhibition percentage of egg albumin denaturation was figured out using the following equation.
Inhibition of denaturation (%) = [(Abscontrol – Abssample)/Abscontrol] × 100
where Abscontrol is the absorbance of control solution; and Abssample denotes the absorbance of T-AgNPs or extract or diclofenac sodium.
Membrane stabilization test
Red blood cell (RBC) suspension preparation: Erythrocytes suspension was prepared using the fresh blood samples obtained from a healthy volunteer according to our previously published protocol[Citation22,Citation32]
Inhibition of heat-induced hemolysis: The test solution (2 ml) contained 1 ml of either T-AgNps, or Tamarix extract (100, 200, 300, 400, 500, 600 μg/ml) and 1 ml of 10% RBC suspension. In control sample, normal saline replaced T-AgNps or extract. Here, aspirin (200 μg/ml) was used as reference drug. The contents were mixed well by gently inverting the tubes, and all the tubes were kept in a water bath at 56°C for 30 min. After completion of incubation, the tubes were cooled under running tap water and the reaction mixture was centrifuged at 2500 rpm for 5 min at room temperature. The supernatant was collected and the absorbance of supernatant was taken at 560 nm. Phosphate buffer was taken as blank.[Citation33] The percentage membrane stabilization activity was calculated by the formula mentioned below.
Percentage protection = [(Abscontrol – Abssample)/Abscontrol] × 100
where Abscontrol is the absorbance of control solution; and Abssample denotes to the absorbance of sample having T-AgNps, or Tamarix extract or aspirin
Inhibition of hyposaline induced hemolysis: This assay was performed by adding hyposaline (2 ml), phosphate buffer (pH 7.4, 0.1 M), 0.5 ml HRBC suspension (10% v/v) and 1 ml of T-AgNps or Tamarix extract (100, 200, 300, 400, 500 and 600 μg/ml). The control solution did not have any test or drug solution and distill water replaced hyposaline in it. The mixtures were incubated for 30 min at 37°C. Later, the reaction mixtures were centrifuged at 3000 rpm for 10 min and supernatant was obtained. The absorbance of collected supernatant was measured at 560 nm.[Citation15,Citation22] Diclofenac (200 μg/ml) was used as a reference drug. The percent protection from hyposalinity-induced hemolysis was calculated by assuming 100% hemolysis in control.
% protection = 100 – [(Abssample/Abscontrol) × 100]
Percentage protection = [(Abscontrol – Abssample)/Abscontrol] × 100
where Abscontrol is the absorbance of control solution; and Abssample denotes to the absorbance of sample having T-AgNps, or Tamarix extract or diclofenac.
Antiglycating and AGEs inhibiting property
Incubation of T-AgNps or tamarix leaf extract with in vitro glycation system: BSA and glucose were used for in vitro glycation and AGEs formation analysis[Citation34] T-AgNps and Tamarix extract were used as antiglycating agent, and AGEs formation inhibitor. Briefly, BSA (10 mg/ml) and glucose (500 mM) were mixed with or without either T-AgNps or Tamarix extract (100, 200, 300, 400, 500, 600 µg/ml) of in 0.1 M phosphate buffer (pH 7.4). The mixtures were kept for 15 days at room temperature away from sunlight. Bound and unbound glucose was removed by dialysis of the incubated samples at 37°C against phosphate buffer (50 mM, pH 7.4) overnight. The concentration of BSA in each sample was determined using the molar extinction coefficient method. The samples were immediately stored at −20°C. All experiments were run in triplicate.
Effect of T-AgNps and Tamarix extract on browning in glycated samples: Glycation reaction is a non-enzymatic reaction[Citation35] and it may cause the denaturation of proteins. In diabetes, glycation accelerates the browning of proteins by glucose.[Citation36] Hence, browning intensity can be considered as an indicator of glycation, Browning intensity of glycated samples was recorded by measuring the absorbance of each sample at 420 nm[Citation31,Citation37] using 1 cm path length cell after diluted with distill water. All experiments were carried out in triplicates. The percentage of protection from browning was calculated in following equation.
Percentage protection = [(Abscontrol – Abssample)/Abscontrol] × 100
where Abscontrol is the absorbance of control solution; and Abssample denotes the absorbance of samples
Effect of T-AgNps and Tamarix extract on protein aggregation index: Glycation is an important cause to induce the structural changes in biomolecules including proteins which lead to formation of aggregates. We investigated the protective capacity of T-AgNps and Tamarix extract against protein aggregation induced by glycation and it was determined by measuring the absorbance of glycated samples that was incubated with glucose in the presence or absence of T-AgNps and Tamarix extract (100–600 µg/ml) .[Citation37]The aggregation index for different samples was calculated by using their absorbance at 340 nm as well as 280 nm.
Percentage of protein aggregation index = [A340/(A280 – A340)] × 100
where A340 = Absorbance at 340 nm, and A280 = Absorbance at 280 nm.
Percent inhibition of fibrillar state: Congo red (CR) assay: Congo Red (amyloid specific dye) was prepared in phosphate buffer saline-10% (v/v) ethanol. CR was used in our study to investigate the percent inhibition of fibrillation of BSA induced by glucose mediated glycation[Citation38] . We recorded the absorbances for various samples including AGE-BSA, AGE-BSA kept with T-AgNPs or Tamarix extract (100, 200, 300, 400, 500, 600 µg/ml), control sample (BSA that was incubated without test solution or glucose) separately, as well as for Congo red background. In short, glycated protein solution (500 μl, 100 μM) and Congo red (100 μl, 100 μM) were kept at room temperature. After 10 minutes, we recorded absorbance at 530 nm for each sample. The percent inhibition of amyloid formation was calculated by following formula.
where Abscontrol is the absorbance of control solution; and Abssample denotes the absorbance of sample.
Antimicrobial activity of silver nanoparticles: Antibacterial and antifungal activities were investigated to determine the antimicrobial activity of T-AgNPs. The antimicrobial activity of silver nanoparticles was evaluated using five bacterial strains and one fungal strain. Two strains of gram-positive cocci (staphylococcus aureus ATCC 29213 and Enterococcus faecalis ATCC 29212), three strains of gram-negative bacilli (Escherichia coli ATCC 25922, Klebsiella pneumonia ATCC 700603, and Pseudomonas aeruginosa ATCC 27853) and one stain of yeast like fungi (Candida albicans ATCC 60193) were used in this study. The microbial strains were provided from the Microbiology lab in college of Applied Medical Sciences, Qassim University, KSA. Bacterial and fungal inoculum were prepared from fresh pure cultures in Muller Hinton broth. Each bacterial and fungal suspension was compared with 0.5 McFarland standard. Two concentrations of T-AgNPs (25 and 50 µg/ml) were prepared by serially diluting the stock solution of T-AgNPs (1 mg/ml) in sterile distilled water.
The antimicrobial activity of T-AgNPs against the selected microorganisms was evaluated using the agar well diffusion assay. The bacterial inoculum was spread on the Muller–Hinton Agar using a sterile cotton swab by lawn culture technique. After inoculation, made two wells in the agar plate with the help of the backside blue micropipette tips. The lower portion of the wells was sealed with two drops of melted MHA agar and waited 10 mins to solidify the melted agar. Then, add two different concentrations of nanoparticle extracts (100 µl) to different wells. Erythromycin15, Vancomycin30, Imipenem30, Amikacin30 and Fluconazole10 were used as a positive control. The plates were then incubated for 18–24 h at 370 C. After incubation, we observed the zone of inhibition around the wells and measured the zone diameter in millimeter (mm) by a ruler.
Statistical analysis
The experimental results were carried out in triplicates and it was expressed by mean ± Standard Error. Statistical analysis was performed using Data Entry: ANOVA.
Results
Preliminary screening of leaf and extract
Tamarix leaves are grayish green color, alternate, persistent, and reduced to sheathing scales on twigs (). The ethanolic extract was grayish green, powdery, and without a specific smell. The preliminary screening of extract is listed in .
Table 1. Preliminary screening of Tamarix leaf extract
Phytochemical screening
The phytochemical screening of Tamarix ethanolic leaf extract showed the presence of carbohydrates, flavonoids, saponins, tannins, flavonoids, and polyphenolic compounds. The results of phytochemical screening, color, odor, and texture of extract are summarized in (). Further, the total concentration of phenolic contents was determined by colorimetric assay using gallic acid calibration curve. The total phenolic content in ethanol extracts of tamarix was expressed in mg of gallic acid equivalents per gram dry weight of Tamarix extract and was 98.54 ± 5.76 mg gallic acid equivalent/g dry weight of extract. This is to keep in mind that phenolic contents are correlated with defense against biotic or abiotic stress. The phenolic contents are not associated with the development of plant tissues, and growth functions.[Citation22]
Table 2. Phytochemical screening of Tamarix leaf extract
The total flavonoid content of extract was determined by using aluminum chloride colorimetric assay and the absorbance was measured at 430 nm. The total flavonoid content was expressed as mg quercetin equivalent per g dry weight of T. articulata extract. Total flavonoid content was 61.39 ± 6.01 mg quercetin equivalents/g dried weight of extract. It was seen that the quantity of flavonoids was lesser than total phenolic content.
Characterization of silver nanoparticles by UV visible absorption spectroscopy
During biosynthesis of nanoparticles, the change in color is the prime indication of nanoparticle synthesis. Silver nanoparticles are yellowish brown colored in aqueous solution because of the vibrations of surface plasmon in nanoparticles.[Citation39] Due to the reduction of silver ions to silver nanoparticles by plant extract results in change in the color as well as their UV visible spectra. In our study, the addition of Tamarix leaf extract into silver nitrate solution led to a wide range of color changes from colorless to yellowish and finally, to reddish brown due to exposure of varying time and extract amount () within the reaction. The absorption spectra of T-AgNps was recorded after 24 h after their preparation and the peak of absorption spectra was found to be at 413 nm which is specific for silver nanoparticles ().
Figure 3. (a) Color change of silver nitrate to silver nanoparticles by addition of Tamarix leaf extract. Different vials represent the color of solution. A at 0 min, B at 20 min, C at 30 min, D at 1 h, E at 3 h, F at 24 h. Brown color (F) shows the formation of T-AgNPs. (b) The UV-visible spectra of silver nanoparticles synthesized by Tamarix leaf extract at 24 h
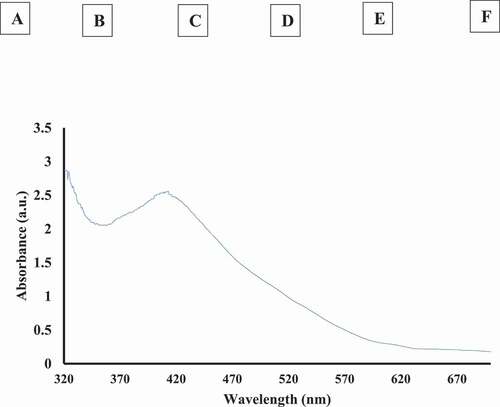
Morphological characterization of T-AgNPs
Electron micrographs exhibited that particles were of various shapes and sizes. It is well known that efficacy of particles is dependent on their sizes and shapes and the smaller particle sizes correlated with greater properties.[Citation40]
Scanning electron microscope (SEM)
SEM images of T-AgNPs are shown in . SEM images analysis predicts the size and morphology of resultant silver nanoparticles and showed that the particle sizes are within 50 nm size (). Further, it showed that T-AgNPs are relatively spherical in shape. show the SEM images of T-AgNPs at different magnifications and 20 kV.
Transmission electron microscopy (TEM)
The morphology, shape, and size details of T-AgNPs have been analyzed by transmission electron microscopy (TEM). The TEM images showed that T-AgNPs were largely uniform with narrow size distribution. Further, TEM analysis displayed that relatively spherical in shape with an average size of about 25 nm. TEM micrograph was processed by Gatan digital micrograph (GMS 3.x digital micrograph software) and different properties of T-AgNPs are presented in (). TEM images and size distributions of T-AgNPs are shown in (). displays the general micrograph of T-AgNPs. demonstrates the histogram and datasheet, respectively. We have used Gatan Digital Micrograph (GMS 3.x digital software. Gatan Microscopy Suite® (GMS) is the industry standard software that is used for (scanning) transmission electron microscope experimental control and analysis.
Table 3. Digital micrograph script of TEM images. Different properties of T-AgNPs
Ferric reducing antioxidant power (FRAP) assay
We investigated the reducing potential of T-AgNps and ethanolic Tamarix extract by FRAP assay using ascorbic acid as standard reference. This assay is based on the reduction of Fe+3 to Fe+2 by the extract and T-AgNPs. The extract and T-AgNPs reduced Fe+3 significantly. Though, FRAP value of T-AgNPs was higher than extract. The ascorbic acid solution (50–250 μg/ml) conformed to Beer’s Law at 700 nm with a regression co-efficient (R2) = 0.9961 and slope (m) = 0.003. The intercept for this plot was 0.0216. The equation of standard curve is y = 0.003x + 0.0216. FRAP value of ethanolic extract of Tamarix extract and T-AgNPs were 19.52 ± 0.158, and 32.41 ± 86 µg ascorbic acid/100 mg dry weight of extract, respectively. It had been found that the ferric reducing power of Tamarix extract and T-AgNPs was increased in a dose dependent manner (). Values are in mean ± SEM.
Hydrogen Peroxide (H2O2) Scavenging ability
Scavenging of H2O2 for different concentrations of T-AgNps and ethanolic Tamarix leaf extract may be attributed to electron donating ability of phenolic components of T-AgNps and ethanolic tamarix extract[Citation41] and was investigated according to previously described method .[Citation22] H2O2 scavenging activity of extract was found to increases in concentration-dependent manner (). The maximum percentage scavenging activity was exhibited by 600 µg/ml of ethanolic extract. This activity of Tamarix extract and T-AgNPs is very important because disintegration of H2O2 gives rise to production of hydroxyl radical. Hydroxyl radical is cytotoxic in nature.[Citation42]
DPPH radical scavenging assay
DPPH-free radical scavenging is one of the most popular method around the world to investigate the antioxidant potentials of different substances. The scavenging activities of Tamarix extract and T-AgNPs on DPPH radical are illustrated in (). Both T-AgNPs and Tamarix extract have significant scavenging effect on DPPH. The DPPH scavenging effect was found to be increased with increase in concentration of T-AgNPs and Tamrix extract from 100 to 600 µg/ml but the scavenging activity of extract was found to be lower than T-AgNPs. Tamarix extract and T-AgNPs showed 46.6% and 68.21% DPPH reducing (scavenging) activity at 600 µg/ml.
Investigation of anti-inflammatory activity by protein denaturation inhibition
It is well known that there are significant ethical issues for the use of animals in research purposes. Hence, we used protein denaturation method to investigate in vitro anti-inflammatory potential of T-AgNPs and ethanolic tamarix extract. During inflammatory and arthritic reactions, tissue proteins have been noticed to be denatured. Hence, the substances who have the ability to protect from denaturation of proteins that would be used in the development of anti-inflammatory drug[Citation43] and to understand the mechanism. Both T-AgNps and Tamarix extract showed dose-dependent inhibition of heat induced albumin denaturation but percent inhibition of denaturation was more for T-AgNPs as compared to ethanolic tamarix extract (). Tamarix extract and T-AgNPs displayed 63.46% and 73.19% protection from denaturation at 600 µg/ml. Ibuprofen was used as a standard anti-inflammatory drug that showed the percentage protection 58.31 ± 0.35% at a concentration of 200 µg/ml (Data not shown in figure).
Anti-proteinase activity
Proteinases are lysosomal enzymes that are involved in the hydrolysis of proteins. During inflammatory reactions, proteinases are implicated in tissue damage. The substances that are capable to inhibit proteinase activity are assumed to provide protection against tissue damage induced by proteinase.[Citation44] presents the antiproteinase activity of T- AgNPs and ethanolic tamarix extract. Both T-AgNPs and Tamarix extract inhibited proteinase activity. At 600 µg/ml, ethanolic Tamarix extract and T-AgNPs sexhibited highest inhibition capacity (48.469% and 70.196%, respectively) (). At all concentrations, inhibitory capacity of T-AgNPs was significantly higher compared to Tamarix extract. We tested the inhibitory capacity of diclofenac sodium against proteinase action and it was showed maximum inhibition 74.231 ± 2.114% at 200 µg/ml (Data not shown in figure).
Inhibition of egg albumin denaturation
The inhibitory capacities against egg albumin denaturation were investigated to evaluate the anti-arthritic effect of T-AgNPs and ethanolic extracts of Tamarix (). Our results suggest that ethanolic extracts of Tamarix and T-AgNPs are highly effective against heat induced egg albumin denaturation and their percent inhibitory capacity was found to be increased with an increase in the concentration. Both ethanolic Tamarix extract and T-AgNPs exhibited egg albumin denaturation inhibition but their percentage inhibitions at a particular concentration were different (47.70% and 73.19% at 600 μg/ml). We used diclofenac sodium as a standard drug who showed a significant inhibition (69.71 ± 1.35%) at a concentration of 200 µg/ml.
Membrane stabilization test
Protecting ability of T-AgNPs and Tamarix extract against erythrocyte membrane lysis induced by heat and hyposaline was evaluated to further establish the mechanism of anti‐inflammatory activity of T-AgNPs and ethanolic Tamarix extracts.
Inhibition of heat-induced hemolysis: We used human erythrocyte membrane stabilization test to determine in vitro anti-inflammatory potential of T-AgNPs and Tamarix extract. Lysosomal membrane is analogous to erythrocyte membrane and lysis of lysosomal membrane releases enzymes and proteases. Many of these lysosomal enzymes and proteases are known to be implicated in inflammatory reaction and are associated with several disorders. Therefore, protection from the lysosomal membrane lysis can be important for prevention of inflammatory response.[Citation45] Our study shows that Tamarix extract and T-AgNPs offer protection against heat induced hemolysis. Tamarix extract and T-AgNPs at different concentrations (100–600 µg/ml) showed considerable stabilization toward erythrocyte membrane. The percentage protection of Tamarix extract and T-AgNPs was higher at 600 µg/ml (49.169% and 74.16%, respectively) (). The standard anti-inflammatory drug aspirin displayed the highest erythrocyte membrane stabilization (75.32 ± 1.228%) at a concentration of 200 µg/ml (Data not shown in figure).
Figure 12. Percentage protection from heat induced hemolysis. X-axis indicates the various concentrations (100–600 µg/ml) of Tamarix extract (blue) and T-AgNPs (yellow). The results are presented as means ± SEM (n = 3, p < .05)
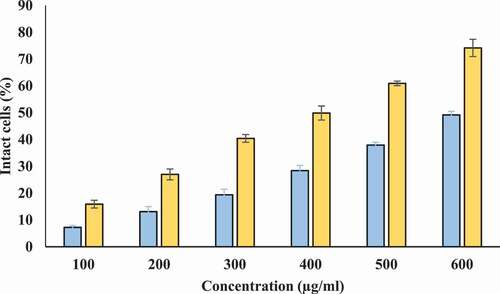
Protection from hyposaline induced hemolysis: Hyposalinity induces lysis of erythrocyte membrane leading to osmotic loss. Hence, protecting ability of Tamarix extract and T-AgNPs against hyposaline induced lysis was tested. Tamarix extract showed protection against hyposaline induced lysis of erythrocyte membrane. However, this protecting ability of Tamarix extract was lower as compared to T-AgNPs. T-AgNPs prevented lysis of erythrocyte membrane at better potency (72.98% at 600 µg/ml) in comparison to Tamarix extract (46.19% at 600 µg/ml) at same concentration. Hence, T-AgNPs could have a significant role in stabilizing the erythrocyte membrane against hyposaline induced lysis and is a potent membrane stabilizer at high concentrations (). Diclofenac sodium showed maximum protection 66.36 ± .1.29% at 200 µg/ml.
Evaluation of protecting ability against glycation by determining browning intensity
Evaluation of protecting ability of T-AgNPs and Tamarix extract against glycation was carried out by determining remained percentage browning intensity of BSA incubated with glucose. Under hyperglycemic conditions, glycation of proteins leads to increase in the browning intensity.[Citation46] In our experiments, we measured degree of browning at 420 nm. The result of antiglycating studies displayed the highest browning intensity for BSA that was incubated with glucose without Tamarix extract and T-AgNPs, We considered 100% browning intensity for this sample. However, a noteworthy reduced browning intensity for BSA was noticed that was kept with glucose but in the presence of Tamarix extract (67.21%) or T-AgNps (51.62%) at a concentration of 600 µg/ml (). In addition, some browning was also noticed in the case of BSA kept without glucose or Tamarix extract/T-AgNPs). The possible explanation for this sample is time-dependent internal structural modifications. These results confirm that extract inhibited the formation of glycated brown products.
Figure 14. Browning intensity (%) in the presence of Tamarix extract and T-AgNPs- Sample 1 (red) presents BSA kept with glucose for 15 days and was assumed to display 100% glycation (browning). Sample 2–7 had various concentrations of Tamarix extract (blue) and T-AgNPs (yellow) (100–600 µg/ml). The browning intensity (the degree of glycation) was found to be gradually decreasing in a concentration dependent manner for all samples having Tamarix extract and T-AgNPs. Sample 8 (green) had BSA that was kept incubated without glucose and extract or T-AgNps (minimum glycation or browning intensity). The results are presented as means ± SEM (n = 3, p < .05)

Protein aggregation inhibition
Glyction results in the attachment of carbonyl groups to protein which subsequently induce the formation of clusters. Therefore, glycation of proteins leads to protein aggregate formation. We found positive influence of Tamarix extract and T-AgNPs on inhibition of protein aggregation. T-AgNPs was shown to be better aggregation and hence, glycation inhibitor in comparison with Tamarix extract. Results clearly show that T-AgNPs are better inhibitors of carbonyl attachment with proteins, i.e., glycation as compared to Tamarix extract. Further, T-AgNPs are assumed to be good blocker of the conversion of intermediate dicarbonyl compounds to AGEs. Moreover, our observation showed that the presence of Tamarix extract and T-AgNPs reduced aggregation index of BSA kept with glucose in a concentration dependent manner (). T-AgNPs and Tamarix extract showed maximum aggregation (48.28% and 55.325%, respectively) at a concentration of 600 µg/ml.
Figure 15. Decrease in aggregation index in presence of Tamarix extract and T-AgNPs. Sample 1 (red) corresponds to BSA incubated with glucose for 15 days and considered to show maximum aggregation index and hence, glycation aggregation index. Sample 2–7 contained 100–600 µg/ml of Tamarix extract (blue) and T-AgNPs (yellow). Sample 8 (green) contained BSA incubated in absence of glucose and Tamarix extract and T-AgNPs and showed least aggregation index. The results are presented as means±SEM (n = 3, p < .05)
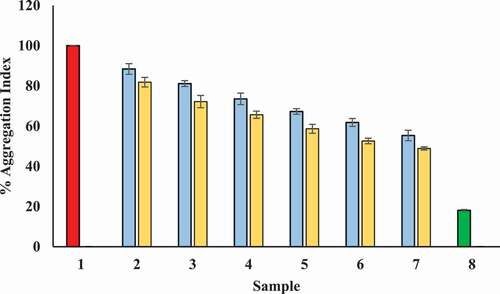
Inhibition of amyloid structure
Congo red (CR) dye can be used to evaluate the degree of modification occurred within the secondary structures of protein. CR has a specific binding capacity to the β-sheet structure of proteins and it binds hydrophobic clefts present between antiparallel beta fibrils. CR dye exhibits specific absorbance at 530 nm after binding. The results of the CR binding assay are presented in . Tamarix extract and T-AgNPs showed reduction in fibrillation of BSA and possibly inhibited the transition of α-helix to β-sheet by concealing the glycation sites and by decreasing the solvent-accessible surface area.
Figure 16. Decrease in cross-amyloid structures in presence of Tamarix extract and T-AgNPs. Sample 1 (red) corresponds to BSA incubated with glucose for 15 days and considered to show maximum structural maximum modifications and hence considered to be 100%. Sample 2–7 contained 100–600 µg/ml of Tamarix extract (blue) and T-AgNPs (yellow), and cross amyloid structures are shown to be decrease with increase in concentration of Tamarix extract and T-AgNPs. Sample 8 (green column) contained BSA incubated in absence of glucose and extract and showed least cross-amyloid structures. The results are presented as means ± SEM (n = 3, p < .05)
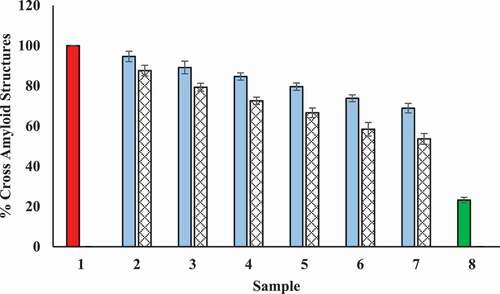
Antimicrobial activity of T-AgNPs
Silver nanoparticles are well known to have antimicrobial activity. Therefore, we confirmed the antimicrobial activity of T-AgNPs against bacterial strains (listed below) as well as Candida albicans (yeast-like fungi) in our study. It was clear that T-AgNPs had good antimicrobial activity against gram-negative bacilli and yeast-like fungi but only mild activity against gram-positive cocci. In this experiment, two concentrations of T-AgNPs (25 µg/ml & 50 µg/ml) were used and zone of inhibition for both concentrations did not show much difference for the tested bacteria and candida. The inhibition zone for these organisms is provided in .
Table 4. Antimicrobial activity of T-AgNPs. The zone of inhibition for different test bacterial strains and C. albicans is provided for two concentrations T-AgNPs (25 and 50 µg/ml). Various positive controls and their zone of inhibition against microbes are also provided in the table. The results are presented as means ± SEM (n = 3, p < .05)
Discussion
The synthesis of nanoparticles using biological methods is a valuable alternative to chemical and physical methods. Besides, biosynthesized silver nanoparticles have been reported to have a wide range of applications in biomedical sciences. In this context, diverse natural products have been used for the biosynthesis of silver nanoparticles. However, there is a continuous search for better reducing natural product for synthesizing stable, and broadly effective silver nanoparticles.[Citation47] In addition, natural products are important part of traditional medicinal system for human health maintenance and combatting diseases. Several secondary metabolites of plants including flavonoids, tannins, terpenoids, and alkanoids have been described for in vitro medicinal properties.[Citation48]
Tamarix articulata is an important halophytic plant and has been reported to have several medicinal properties. Being a halophytic plant, Tamarix has the ability to endure the salt stress, and correlated oxidative stress due to diverse kind of phytochemicals. Most of the studies related to biosynthesized silver nanoparticles are based on their antibacterial activity. Therefore, the current study aims to investigate the anti-inflammatory, antiglycating, and antimicrobial properties of silver nanoparticles. For this purpose, we synthesized silver nanoparticles using Tamarix ethanol extract. To the best of our knowledge, there is no previous study regarding the synthesis of silver nanoparticles using this plants. Using various phytochemical screening assays, we confirmed the presence of several health beneficial phytochemicals including polyphenolic compounds, flavonoids, saponins, and tannins in Tamarix ethanol extract. These compounds could be suggested to be responsible for bioreduction, and capping of T-AgNPs.
Our data suggested that exposure of Tamarix extract reduced Ag+ ions as indicated by visual observation and UV-visible spectroscopy. It was seen that the color of solution was changed gradually from colorless to deep brown. After biosynthesizing T-AgNPs, UV-visible spectra was taken between the wavelengths ranges of 300–700 nm. In our study, a peak at 413 nm was recorded for this solution which is very close to characteristic peak of silver nanoparticles as reported by previous researchers.[Citation49,Citation50] Moreover, the absorption intensity was found to be increased with time which indicated the presence of T-AgNPs in the solution. TEM was carried out to find the structure and size distribution of T-AgNPs. In our study, TEM revealed that T-AgNPs were largely uniform, spherical, with an average size of 25 nm, and with narrow size distribution. Visualization of T-AgNPs by SEM confirmed the spherical shape of T-AgNPs. After their biosynthesis, and characterization, antioxidant, membrane stabilizing, inhibitory property against heat-induced denaturation of proteins, antiglycating, and AGEs formation inhibition potential of T-AgNPs were accomplished.
Ethanol extract of Tamarix showed the presence of remarkable amount of polyphenolic compounds and flavonoids, which are main contributors to health beneficial activities of a plant product due to their antioxidant activities.[Citation22]Possible explanation for the formation of T-AgNPs is the interaction of proteins, metabolites, functional groups such as carboxylic group, and amino groups present in Tamarix leaf extract with silver surface. In the current study, T-AgNPs and Tamarix ethanol extract both displayed promising antioxidant activities (in a concentration dependent manner) as confirmed by FRAP, DPPH, and H2O2 assays. However, T-AgNPs exhibited better antioxidant potential as compared to extract which may be due to difference in reaction mechanism. The antioxidant activity of T-AgNPs might be due to interference of several functional groups present in the extract. These functional groups might be responsible for capping and stabilization procedure of T-AgNPs synthesis.[Citation51] The inflammation is a major health problem worldwide and is stimulated by irritants and pathogens.[Citation48] Despite this, inflammation is often a healing process of the body but it is very painful and uncomfortable. Moreover, inflammation has been suggested to be associated with malignant cell persistence and invasion through different cellular and molecular mechanisms.[Citation52] Many anti-inflammatory synthetic drugs are available to suppress the symptoms of inflammation. These anti-inflammatory chemical drugs interfere the natural repairing and healing ability of body’s immune system. Thus, the use of natural products can be a better substitute of chemical drugs to achieve true and ultimate healing of the body which is a true property of anti-inflammatory drug. Various methods have been employed to screen in vitro anti-inflammatory activity of drugs such as fibrinolytic assays, platelet aggregation, uncoupling of oxidative phosphorylation, erythrocyte membrane stabilization, lysosomal membrane stabilization, anti-proteinase assays, and inhibition of protein denaturation.[Citation53] Stabilization of erythrocyte membrane can be considered to be an in vitro model for screening of anti-inflammatory effect of T-AgNPs because this membrane is analogues to lysosomal membrane. Stabilization of lysosomal membrane is very important step to avoid the release of lysosomal enzymes and hence, in preventing inflammatory process. The release of lysosomal enzymes may cause the damaging of bio macromolecules and lipid peroxidation of membranes.[Citation54]
In the current study, both T-AgNPs and Tamarix extract exhibited potential inhibitory property against heat-induced protein denaturation and proteinase action. In addition, T-AgNPs stabilized the erythrocyte membrane in a concentration dependent manner. Likewise, Tamarix extract also showed protection against heat and hyposaline induced lysis of erythrocyte membrane. However, the beneficial effects of T-AgNPs against denaturation of proteins, proteinase action, and erythrocyte membrane lysis were observed at much lower concentration as compared to Tamrix extract. Further, these effects of T-AgNPs were analogous to standard reference drugs.
Glycation contributes to structural and functional deterioration of biomolecules and is a major factor implicated in pathogenesis of diabetes and its complications.[Citation55,Citation56] Therefore, to manage diabetes and associated complications in diabetic patients, inhibition of glycation can be considered as an important strategy for alleviating complications.[Citation57] Moreover, inhibition of protein glycation has been suggested to understand the mechanism of protection against hyperglycemic conditions in diabetes.[Citation58] In our investigations. BSA was used as a model protein for this experiment and the increase in browning intensity was used as a marker of glycation.[Citation22] The conformational changes induced by glycation increases the amyloid cross β structures in proteins. The increase in amyloid cross β structures leads to aggregation of proteins indicating the formation of AGEs. We evaluated the protein aggregation by using a β amyloid specific dye, i.e., Congo Red. Congo Red is a specific dye that binds as a planar molecule in whether a monomeric or supramolecular form in grooves that are developed along the β – sheets. Our data indicated that browning intensity, cross amyloid structures and aggregation index of glycated BSA were significantly high as compared to BSA kept in the absence of glucose. T-AgNPs have shown the significant antiglycating potential as confirmed by decreased browning intensity, aggregation index, and amyloid structures of glycated BSA incubated with T-AgNPs. Crude Tamarix extract similarly showed antiglycating and AGEs formation inhibition activity. However, these activities of T-AgNPs were considerably high in comparison with those of extract.
There are several theories regarding the antimicrobial activities of silver nanoparticles (SNP). According to one theory, electrostatic attraction between positive charge on silver nanoparticles and negative charge on cell membrane contribute to their antimicrobial activity. Another theory suggests that concentration of SNPs is the main contributing factor for antimicrobial activity (charge on SNPs does not matter), and it is closely associated with formation of pits in cell membrane. The accumulation silver nanoparticles on cell membrane leads to permeability of cell membrane which causes the cell death. Further, increased permeability may lead to continuous release of lipopolysaccharides and membrane proteins also.[Citation59] In 2015, Ibrahim has suggested more possible mechanisms for the antimicrobial properties of silver nanoparticles. AgNPs might produce free radicals, and attach with surface of a cell membrane. Thus, they can disturb important functions including electron transport, osmoregulation, permeability, and respiration. Further, silver nanoparticles can interact with thiol groups in enzymes and phosphorus-containing cell constituents and DNA. This may prevent DNA replication and cell division and may result in cell death.[Citation60]
Oxidative stress mediated health complications including inflammation are major contributing factors for the health complications of diabetic patients. Advanced glycation end products (AGEs) and non-enzymatic glycation have been reported to be implicated in secondary complications of diabetes. Therefore, new compounds are being screened for their potential AGEs formation inhibiting property. Tamarix articulata are halophytic plants with several medicinal properties. However, Tamarix is not yet much used in modern medicine. In the present study, first time synthesized nanoparticles using Tamarix leaf extract. The novelty of our work lies in the investigation of the inhibitory effects of antimicrobial, anti-inflmmatory, membrane stabilizing, antiglycating and AGEs formation inhibiting properties of AgNPs (silver nanoparticles) in a single study. Further, we have the compared these properties of T-AgNPs with extract.
In our investigation, we observed different biological activities of T-AgNPs were more significant as compared with Tamarix extract. It may be due to the change in properties and behavior of substances in biological environment during nanoparticle formation. In addition, nanoparticles might promote sustained release of active ingredients, reduce the required dose, decrease side effects, and improve the activity.[Citation61]
Conclusion
T. articulata leaf ethanol extract was successfully used for quick biosynthesis of nanoparticles. T-AgNPs were characterized and were investigated for their antioxidant, anti-inflammatory, antiglycating, and AGEs formation inhibition as well as anti-microbial activity. T-AgNPs were found to be uniform and spherical. Synthesized silver nanoparticles revealed excellent antioxidant, antiglycating, AGEs formation inhibition activities and excellent antimicrobial activity against the selected microorganisms. Moreover, they showed protection from heat induced denaturation of proteins and were capable of maintaining the stability of erythrocytes. Thus, these silver nanoparticles may be used in the treatment of various oxidative stress mediated health disorders, pain and swelling associated with inflammation. Our results indicated that antiglycating and AGEs formation inhibition properties of these nanoparticles make them a suitable candidate for the therapeutics for complications in diabetic patients. Further, T-AgNPs can be used against various infections in diabetic patients. As a conclusion, our findings suggest that biosynthesized T-AgNPs can be used as potential drug candidates with high therapeutic applications in various medical fields.
Acknowledgments
Researchers would like to thank the Deanship of Scientific Research, Qassim University for funding publication of this project.
References
- Govarthanan, M.; Selvankumar, T.; Manoharan, K.; Rathika, R.; Shanthi, K.; Lee, K. J.; Cho, M.; Kamala-Kannan, S.; Oh, B. T. Biosynthesis and Characterization of Silver Nanoparticles Using Panchakavya, an Indian Traditional Farming Formulating Agent. Int. J. Nanomed. 2014, 9, 1593–1599. DOI: https://doi.org/10.2147/IJN.S58932.
- Lee, K.-J.; Park, S.-H.; Govarthanan, M.; Hwang, P.-H.; Seo, Y.-S.; Cho, M.; Lee, W.-H.; Lee, J.-Y.; Kamala-Kannan, S.; Oh, B.-T. Synthesis of Silver Nanoparticles Using Cow Milk and Their Antifungal Activity against Phytopathogens. Mater. Lett. 2013, 105, 128–131. DOI: https://doi.org/10.1016/j.matlet.2013.04.076.
- Helmy, A.; El-Shazly, M.; Seleem, A.; Abdelmohsen, U.; Alaraby Salem, M.; Samir, A.; Rabeh, M.; Elshamy, A.; Singab, A. N. B. The Synergistic Effect of Biosynthesized Silver Nanoparticles from a combined Extract of Parsley, Corn Silk, and Gum Arabic: In Vivo Antioxidant, Anti-inflammatory and Antimicrobial Activities. Mater. Res. Express. 2020, 7, 025002.
- Khandel, P.; Shahi, S. K.; Kanwar, L.; Yadaw, R. K.; Soni, D. K. Biochemical Profiling of Microbes Inhibiting Silver Nanoparticles Using Symbiotic Organisms. Int. J. Nano. Dimens. 2018, 9, 273–285.
- Deyá, C.; Bellotti, N. Biosynthesized Silver Nanoparticles to Control Fungal Infections in Indoor Environments. Adv. Nat. Sci.: Nanosci. Nanotechnol. 2017, 8, 8025005.
- Johnson, P.; Krishnan, V.; Loganathan, C.; Govindhan, K.; Raji, V.; Sakayanathan, P.; Vijayan, S.; Sathishkumar, P.; Palvannan, T. Rapid Biosynthesis of Bauhiniavariegata Flower Extract-mediated Silver Nanoparticles: An Effective Antioxidant Scavenger and α-amylase Inhibitor. Artif. Cells Nanomed and Biotechnol. 2018, 46, 1488–1494. DOI: https://doi.org/10.1080/21691401.2017.1374283.
- Antony, E.; Sathiavelu, M.; Arunachalam, S. Synthesis of Silver Nanoparticles from the Medicinal Plant Bauhinia Acuminata and Biophytum Sensitivum–a Comparative Study of Its Biological Activities with Plant Extract. Int. J. App. Pharm. 2017, 9, 22–29. DOI: https://doi.org/10.22159/ijap.2017v9i1.16277.
- Aravinthan, A.; Govarthanan, M.; Selvam, K.; Praburaman, L.; Selvankumar, T.; Balamurugan, R.; Kamala-Kannan, S.; Kim, J. Sunroot Mediated Synthesis and Characterization of Silver Nanoparticles and Evaluation of Its Antibacterial and Rat Splenocyte Cytotoxic Effects. Internat. J. Nanomed. 1977-1983, 2015(10).
- Govarthanan, M.; Seo, Y.-S.; Lee, K.-J.; Jung, I.-B.; Ju, H.-J.; Kim, J. S.; Cho, M.; Kamala-Kannan, S.; Oh, B.-T. Low-cost and Eco-friendly Synthesis of Silver Nanoparticles Using Coconut (Cocos Nucifera) Oil Cake Extract and Its Antibacterial Activity. Artif. Cells Nanomed. Biotechnol. 2016, 44(44), 1878–1882. DOI: https://doi.org/10.3109/21691401.2015.1111230.
- Rajawat, S.; Qureshi, M. S. Comparative Study on Bactericidal Effect of Silver Nanoparticles, Synthesized Using Green Technology, in Combination with Antibiotics on Salmonella Typhi. J. Biomater. Nanobiotechnol. 2012, 3, 480–485. DOI: https://doi.org/10.4236/jbnb.2012.34049.
- Muthusamy, G.; Thangasamy, S.; Raja, M.; Chinnappan, S.; Kandasamy, S. Biosynthesis of Silver Nanoparticles from Spirulina Microalgae and Its Antibacterial Activity. Environ. Sci. Pollut. Res. 2017, 24, 19459–19464. DOI: https://doi.org/10.1007/s11356-017-9772-0.
- Mythili, R.; Selvankumar, T.; Kamala-Kannan, S.; Sudhakar, C.; Ameen, F.; Al-Sabri, A.; Selvam, K.; Govarthanan, M.; Kim, H. S. Utilization of Market Vegetable Waste for Silver Nanoparticle Synthesis and Its Antibacterial Activity. Mater. Lett. 2018, 225, 101–104. DOI: https://doi.org/10.1016/j.matlet.2018.04.111.
- Ameen, F.; AlYahya, S.; Govarthanan, M.; ALjahdali, N.; Al-Enazi, N.; Alsamhary, K.; Alshehri, W. A.; Alwakeel, S. S.; Alharbi, S. A. Soil Bacteria Cupriavidus Sp. Mediates the Extracellular Synthesis of Antibacterial Silver Nanoparticles. J. Mol. Struct. 2020, 1202, 127233. DOI: https://doi.org/10.1016/j.molstruc.2019.127233.
- Aslam, M.; Ahmad, M. Alhagi Maurorum and Tamarix Aphylla – two Medicinal Weeds Mentioned in Holy Quran and Ahadith and Their Ethnomedicinal Uses in District Rajhanpur of Pakistan. Univers. J. Pharm. Res. 2016, 1. DOI: https://doi.org/10.22270/ujpr.v1i1.RW5.
- Rahmani, A. H.; Al Zohairy, M. A.; Aly, S. M.; Khan, M. A. Curcumin: A Potential Candidate in Prevention of Cancer via Modulation of Molecular Pathways. Biomed. Res. Int. 2014, 2014, 761608. DOI: https://doi.org/10.1155/2014/761608.
- Rahmani, A. H.; AlSahli, M. A.; Aly, S. M.; Khan, M. A.; Aldebasi, Y. H. Role of Curcumin in Disease Prevention and Treatment. Adv. Biomed. Res. 2018, 7, 38. DOI: https://doi.org/10.4103/abr.abr_147_16.
- Rahmani, A. H.; Allemailem, K. S.; Aly, S. M.; Khan, M. A. Implications of Green Tea and Its Constituents in the Prevention of Cancer via the Modulation of Cell Signalling Pathway. Biomed. Res. Int. 2015, 12. Article ID 925640. DOI: https://doi.org/10.1155/2015/925640.
- Alnuqyadan, A.; Rah, B. Comparative Assessment of Biological Activities of Different Parts of Halophytic Plant Tamarix Articulata (T. Articulata) Growing in Saudi Arabia. Saudi J. Biol. Sci. 2020, 27(10), 2586–2592. DOI: https://doi.org/10.1016/j.sjbs.2020.05.028.
- Alnuqyadan, A.; Rah, B. A. Tamarix Articulata (T. Articulata) - an Important Halophytic Medicinal Plant with Potential Pharmacological Properties. Curr. Pharm. Biotechnol. 2019, 20(4). DOI: https://doi.org/10.2174/1389201020666190318120103.
- Marwat, S. K.; Khan, M. A.; Khan, M. A. Fazal-ur-Rehman; Ahmad, M.; Zafar, M. Salvadora Persica, Tamarix Aphylla and Zizyphus Mauritiana – three Woody Plant Species Mentioned in Holy Quran and Ahadith and Their Ethnobotanical Uses in North Western Part (D.I. Khan) of Pakistan. Ethnobot. Leaflets. 2008, 2, 1013–1021.
- Reginato, M. A.; Castagna, A.; Furlán, A.; Castro, S.; Ranieri, A.; Luna, V. Physiological Responses of a Halophytic Shrub to Salt Stress by Na2SO4 and NaCl: Oxidative Damage and the Role of Polyphenols in Antioxidant Protection. AoB Plants. 2014, 6, plu042. DOI: https://doi.org/10.1093/aobpla/plu042.
- Anwar, S.; Almatroudi, A.; Allemailem, K. S.; Jacob Joseph, R.; Khan, A. A.; Rahmani, A. H. Protective Effects of Ginger Extract against Glycation and Oxidative Stress-Induced Health Complications: An in Vitro Study. Processes. 2020, 8, 468. DOI: https://doi.org/10.3390/pr8040468.
- Khan, M. A.; Anwar, S.; Aljarbou, A. N.; Al-Orainy, M.; Aldebasi, Y. H.; Islam, S.; Younus, H. Protective Effect of Thymoquinone on Glucose Ormethylglyoxal-induced Glycation of Superoxidedismutase. Int. J. Biol. Macromol. 2014, 65, 16–20. DOI: https://doi.org/10.1016/j.ijbiomac.2014.01.001.
- Anwar, S.; Khan, M. A.; Sadaf, A.; Younus, H. A Structural Study on the Protection of Glycation of Superoxide Dismutase by Thymoquinone. Int. J. Biol. Macromol. 2014, 69, 476–481. DOI: https://doi.org/10.1016/j.ijbiomac.2014.06.003.
- Odebiyi, O. O.; Sofowora, E. A. Phytochemical Screening of Nigerian Medicinal Plants. Litoydia. 1979, 41(3), 234–246.
- Taie, H. A. A.; Salama, Z. A. E. R.; Radwan, S. Potential Activity of Basil Plants as a Source of Antioxidants and Anticancer Agents as Affected by Organic and Bio-organic Fertilization. Not. Bot. Horti. Agrobo. 2010, 38, 119127.
- Qais, F. A.; Shafiq, A.; Khan, M. H.; Ahmad, I.; Alsalme, A.; Ahmad, I. Antibacterial Effect of Silver Nanoparticles Synthesized Using Murraya Koenigii (L.) Against Multidrug-Resistant Pathogens. Bioinorg. Chem. Appl. 2019, 2019, 1–11. |Article ID 4649506. DOI: https://doi.org/10.1155/2019/4649506.
- Almatroodi, S. A.; Anwar, S.; Almatroudi, A.; Khan, A. A.; Alrumaihi, F.; Alsahli, M. A.; Rahmani, A. H. Hepatoprotective Effects of Garlic Extract against Carbon Tetrachloride (Ccl4)-induced Liver Injury via Modulation of Antioxidant, Anti-Inflammatory Activities and Hepatocyte Architecture. Appl. Sci. 2020, 10, 6200. DOI: https://doi.org/10.3390/app10186200.
- Alsahli, M. A.; Almatroodi, S. A.; Almatroudi, A.; Khan, A. A.; Anwar, S.; Almutary, A. G.; Alrumaihi, F.; Rahmani, A. H. 6-Gingerol, a Major Ingredient of Ginger Attenuates Diethylnitrosamine-Induced Liver Injury in Rats through the Modulation of Oxidative Stress and Anti-Inflammatory Activity. Mediators Inflamm. 2021, 2021, 1–17. Article ID 6661937. DOI: https://doi.org/10.1155/2021/6661937.
- Mizushima, Y.; Kobayashi, M. Interaction of Anti‐inflammatory Drugs with Serum Proteins, Especially with Some Biologically Active Proteins. J. Pharma. Pharmacol. 1968, 20, 169‐173. DOI: https://doi.org/10.1111/j.2042-7158.1968.tb09718.x.
- Oyedepo, O. O.; Femurewa, A. J. Anti‐protease and Membrane Stabilizing Activities of Extracts of Fagra Zanthoxiloides, Olax Subscorpioides and Tetrapleura Tetraptera. Int. J. Pharmacong. 1995, 33, 65‐69.
- Chanda, S.; Juvekar, A. In Vitro Anti-inflammatory Activity of Syringic Acid. Int. J. Pharm. Pharm. Sci. 2019, 11, 71–73.
- Govindappaa, M.; Hemashekhar, B.; Arthikala, M. K.; Ravishankar Raid, V.; Ramachandra, Y. L. Characterization, Antibacterial, Antioxidant, Antidiabetic, Anti-inflammatory and Antityrosinase Activity of Green Synthesized Silver Nanoparticles Using Calophyllum Tomentosum Leaves Extract. Results. Phys. 2018, 9, 400–408. DOI: https://doi.org/10.1016/j.rinp.2018.02.049.
- Brownlee, M.; Vlassara, H.; Kooney, A.; Ulrich, P.; Cerami, A. Aminoguanidine Prevents Diabetes Induced Arterial Wall Protein Cross-linking. Science. 1986(232), 1629-1632.
- Younus, H.; Anwar, S. Prevention of Non-enzymatic Glycosylation (Glycation): Implication in the Treatment of Diabetic Complication. Int. J. Health. Sci. (Qassim). 2016, 10, 261–277.
- Matsuura, N.; Aradate, T.; Sasaki, C.; Kojima, H.; Ohara, M.; Hasegawa, J.; Ubukata, M. Screening System for the Maillard Reaction. Inhibitor from Natural Product Extracts. J. Health Sci. 2002, 48(6), 520–526. DOI: https://doi.org/10.1248/jhs.48.520.
- Kumar, D.; Ali, A. Antiglycation and Antiaggregation Potential of Thymoquinone. Nat. Volatiles Essent. Oils. 2019, 6(1), 25–33.
- Klunk, W. E.; Jacob, R. F.; Mason, R. P. Quantifying Amyloid Beta-peptide (Abeta) Aggregation Using the Congo red-Abeta (Cr-abeta) Spectrophotometric Assay. Anal. Biochem. 1999, 266, 66–76. DOI: https://doi.org/10.1006/abio.1998.2933.
- Shankar, S. S.; Rai, A.; Ahmad, A.; Sastry, M. Rapid Synthesis of Au, Ag, and Bimetallic Au Core Ag Shell Nanoparticles usingNeem (Azadirachta Indica) Leaf Broth. J. Colloid Interface Sci. 2004, 275, 496–502. DOI: https://doi.org/10.1016/j.jcis.2004.03.003.
- Hoshyar, N.; Gray, S.; Han, H.; Bao, G. The Effect of Nanoparticle Size on in Vivo Pharmacokinetics and Cellular Interaction. Nanomedicine (Lond). 2016, 11(6), 673–692. DOI: https://doi.org/10.2217/nnm.16.5.
- Nabavi, S. M.; Ebrahimzadeh, M. A.; Nabavi, S. F.; Hamidinia, A.; Bekhradnia, A. R. Determination of Antioxidant Activity, Phenol and Flavonoids Content of Parrotia Persica Mey. Pharmacology Online. 2008, 2, 560–567.
- Nabavi, S. M.; Ebrahimzadeh, M. A.; Nabavi, S. F.; Jafari, M. Free Radical Scavenging Activity and Antioxidant Capacity of Eryngium Caucasicum Trautv and Froripia Subpinnata. Pharmacology Online. 2008, 3, 19–25.
- Chatterjee, P.; Chandra, S.; Dey, P.; Bhattacharya, S. Evaluation of Anti-inflammatory Effects of Green Tea and Black Tea: A Comparative in Vitro Study. J. Adv. Pharm. Technol. Res. 2012, 3(2), 136–138. DOI: https://doi.org/10.4103/2231-4040.97298.
- Das, S. N.; Chatterjee, S. Long Term Toxicity Study of ART‐400. Indian Indg. Med. 1995, 16(2), 117‐123.
- Leelaprakash, G.; Mohan Dass, S. In Vitro Anti-Inflammatory Activity of Methanol Extract of Enicostemma Axillare. Int. J. Drug Dev. Res. 2011, 3(3), 189–196.
- Ajandouz, E. H.; Tchiakpe, L. S.; Ore, F. D.; Benajiba, A.; Puigserver, A. Effect of pH on Caramelization and Maillard Reaction Kinetics in Fructose-lysine Model Systems. J. Food Sci. 2011, 66, 926–931. DOI: https://doi.org/10.1111/j.1365-2621.2001.tb08213.x.
- Das, P.; Ghosal, K.; Jana, N. K.; Mukherjee, A.; Basak, P. Green Synthesis and Characterization of Silver Nanoparticles Using Belladonna Mother Tincture and Its Efficacy as a Potential Antibacterial and Anti-inflammatory Agent. Mater. Chem. Phys. 2019, 228, 310–317. DOI: https://doi.org/10.1016/j.matchemphys.2019.02.064.
- Sri Kumaran, N.; Vijayaraj, R. Biosynthesis of Silver Nanoparticles Using Abutilon Indicum (Link): An Investigation of Anti-inflammatory and Antioxidant Potential against Carrageen Induced Paw Edema in Rats. Asian J. Pharm. Sci. 2017, 11(2), 92.
- Petit, C.; Lixon, P.; Pileni, M. P. In Situ Synthesis of Silver Nanocluster in AOT Reverse Micelles. J. Phys. Chem. 1993, 97, 12974. DOI: https://doi.org/10.1021/j100151a054.
- Kong, H.; Jang, J. One-step Fabrication of Silver Nanoparticle Embedded Polymer Nanofibers by Radical-mediated Dispersion Polymerization. Chem. Commun. 2006, 3010–3012. DOI: https://doi.org/10.1039/b605286j.
- Das, G.; Patra, J. K.; Basavegowda, N.; Vishnuprasad, C. N.; Shin, H. S. Comparative Study on Antidiabetic, Cytotoxicity, Antioxidant and Antibacterial Properties of Biosynthesized Silver Nanoparticles Using Outer Peels of Two Varieties of Ipomoea Batatas (L.) Lam. Int. J. Nanomed. 2019, 14, 4741–4754. DOI: https://doi.org/10.2147/IJN.S210517.
- Anwar, S.; Almatroudi, A.; Alsahli, M. A.; Khan, M. A.; Khan, A. A.; Rahmani, A. H. Natural Products: Implication in Cancer Prevention and Treatment through Modulating Various Biological Activities. Anticancer Agents Med. Chem. 2020, 20(17), 2025–2040. DOI: https://doi.org/10.2174/1871520620666200705220307.
- Gupta, S.; Kohli, S.; Dwivedi, S. In-Vitro Anti-inflammatory Activity of Sarcostemma Acidum Wight. & Arn. Indian Herb by Human Red Blood Cell Membrane Stabilization Method. I. J. P. T. P. 2011, 2(4), 184–188.
- Vijayakumar, S.; Divya, M.; Vaseeharan, B.; Chen, C.; Biruntha, M.; Silva, P.; Durán‑Lara, E. F.; Shreema, K.; Ranjan, S.; Dasgupta, N. Biological Compound Capping of Silver Nanoparticle with the Seed Extracts of Blackcumin (Nigella Sativa): A Potential Antibacterial, Antidiabetic, Anti-inflammatory, and Antioxidant. J. Inorg. Organomet. Polym. Mater. 2020, 31, 624–635. DOI: https://doi.org/10.1007/s10904-020-01713-4.
- Anwar, S.; Younus, H. Antiglycating Potential of Ellagic Acid against Glucose and Methylglyoxal-induced Glycation of Superoxide Dismutase. J. P. P. 2017, 8, 1–12.
- Younus, H.; Anwar, S. Antiglycating Activity of Aloe Vera Gel Extract and Its Active Component Aloin. Proteins Proteom. 2018, 9, 115–125.
- Anwar, S.; Younus, H. Inhibitory Effect of Alliinfrom Allium Sativum on the Glycation of Superoxide Dismutase. Int. J. Biol. Macromol. 2017, 103, 182–193. DOI: https://doi.org/10.1016/j.ijbiomac.2017.05.043.
- Miroliaei, M.; Aminjafari, A.; Ślusarczyk, S.; Nawrot-Hadzik, I.; Rahimmalek, M.; Matkowski, A. Inhibition of Glycation-induced Cytotoxicity, Protein Glycation, and Activity of Proteolytic Enzymes by Extract from Perovskia Atriplicifolia Roots. Pharmacogn. Mag. 2017, 13(Suppl 3), S676–S683. DOI: https://doi.org/10.4103/pm.pm_559_16.
- Vijaykumar, M.; Priya, K.; Nancy, F. T.; Noorlidah, A.; Ahmed, A. B. A. Biosynthesis, Characterization, and Antibacterial Effect of Plant-mediated Silver Nanoparticles Using Artemisia Nilagirica. Ind. Crops Prod. 2013, 41, 215–240.
- Ibrahim, H. M. M.;. Green Synthesis and Characterization of Silver nanoparticles Using Banana Peel Extract and Their Antimicrobial Activity against Representative Microorganisms. J. Radiat. Res. Appl. Sc. 2015, 8, 265–275. DOI: https://doi.org/10.1016/j.jrras.2015.01.007.
- Bonifácio, B. V.; Da Silva, P. B. Nanotechnology-based Drug Delivery systems and Herbal Medicines: A Review. Int. J. Nanomedicine. 2014, 9, 1–15.