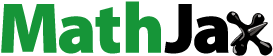
ABSTRACT
Microgreens are an emerging class of fresh food with a high potential for the natural health supplement and support system of space life. Although beet microgreens are praised for their unique color, flavor, and overall eating quality, the study of their physicochemical composition is limited. In this study, profile of total chlorophylls, carotenoids, betalains, ascorbic acid, polyphenols, and equivalent antioxidant capacities (EAC) (as ascorbic acid) of beet (Beta vulgaris) microgreens of day 9 to day 21 were assayed and their correlations were evaluated. All investigated parameters were minimum on day 9 and significantly (p < 0.05) increased to the maximum on day 15. No significant (p > 0.05) reduction occurred in chlorophylls and carotenoids content after day 15, but all other parameters were significantly (p < 0.05) reduced. About 120 g of fresh microgreen could solely fulfill the recommended daily intake of ascorbic acid. Chlorophylls, carotenoids, and polyphenols content were lower as compared to other microgreens. Despite this, beet microgreens possessed high EACs as ascorbic acid due to high betalains content. Very high correlations (>0.76) were observed among all investigated parameters. This study demonstrates beet microgreens as a powerful natural antioxidant. Cell-based and human intervention studies are recommended to draw valuable information about potential bioactivity in humans.
Introduction
Microgreens are young plantlets, 1–3 weeks of age, with the first set of true leaves.[Citation1–3] They can be grown from almost all seeds of grains, vegetables, and herbs.[Citation2,Citation4] Some popular examples include wheat, barley, amaranth, beet, radish, kale, cabbage, broccoli, mustard, lettuce, spinach, coriander, basil, etc.[Citation2,Citation3,Citation5,Citation6] They are gaining popularity as an exciting new culinary food ingredient for the 21st century to enhance salads and for garnishing soups, sandwiches, and wide varieties of other dishes.[Citation7–9] They are usually harvested at the first true leaf stage and marketed with the stem,[Citation9] but containerized production is becoming popular as they offer an additional advantage of harvesting freshly before consumption with less waste than mature counterparts.[Citation3,Citation10] Other than in fresh form, they are available in the market in the form of tablets, frozen juice, and powders.[Citation6,Citation11]
Microgreens are an emerging class of fresh food that grows conveniently in a short time and limited facilities but have high nutritional value especially in terms of bioactive components. With minimal horticultural input, microgreens high harvest index (≈90%) and crop efficiency per unit area (with or without soil), time, and volume.[Citation10,Citation12] , As compared to the counterpart seeds, sprouts, baby greens, and mature plants, microgreens are considered superior because of their delicate textures, vibrant color, distinctive flavors, and a high nutrient density, especially micronutrients like amino acids, enzymes, pigments, vitamins, polyphenols, and overall antioxidants capacity.[Citation1,Citation3,Citation6,Citation7,Citation13–15] With all these features, extensive studies of microgreens are in progress as the potential candidate for the natural health supplement and support system of space life.[Citation1,Citation10]
The majority of the values of microgreens are associated with their abundant bioactive molecules like pigments, ascorbic acid, and polyphenols, and eventually a high antioxidant activity in them.[Citation1,Citation6,Citation12,Citation16] Pigments play a role against clinical conditions like thalassemia and hemolytic anemia and reduce the risk of some chronic diseases, such as cancer, cardiovascular diseases, skin diseases, and age-related eye diseases.[Citation16,Citation17] Humans are one of the few mammals unable to synthesize and store ascorbic acid, which is essential for the prevention of scurvy and maintenance of healthy skin, gums, and blood vessels.[Citation18] Lastly, phenolic compounds are secondary metabolites synthesized during normal germination, growth, and development of plants and are reported to be the major contributor to total antioxidant activity in plants.[Citation6] Due to their antioxidative properties, pigments, ascorbic acid, and phenolic compounds are beneficial against diabetes, cardiovascular, and neurodegenerative diseases, mutagenesis, and carcinogenesis.[Citation1,Citation2,Citation6]
With all the above remarks, it is important to note that the bioactive compound contents in microgreens are affected by various growing conditions throughout their growth period. Several such studies have been carried out on the microgreens of wheat, barley, buckwheat, amaranth, radish, kale, cabbage, broccoli, mustard, lettuce, spinach, coriander, basil, etc.[Citation2,Citation4–6,Citation12,Citation13] Although beet microgreens were reported to have superior eating quality compared to those of Dijon mustard, opal basil, red amaranth, pepper cress, and China rose radish,[Citation8] they remain as the least studied microgreens in terms of bioactive compounds content.
Therefore, this study aimed to evaluate if the contents of pigments, ascorbic acid, and total polyphenols and the overall antioxidant capacities of beet microgreens continuously increase during growth in correlation to each other. For this, the changes in the contents of pigments, ascorbic acid, and total polyphenols and the overall antioxidant capacities [as measured by two analytical assays, DPPH (2,2-diphenyl-1-picrylhydrazyl) radical scavenging activity (DPPH assay), and ferric reducing antioxidant power (FRAP assay)] of beet microgreens were assayed from 9 to 21 days. And finally, the correlations among them were evaluated.
Materials and methods
Material
Common beet (Beta vulgaris L.) seeds were collected from Sajha Agrovet, Bharatpur, Bagmati Province, Nepal in 2019. The seeds were screened for removal of extraneous matter, and small/damaged kernels. The germination activity of the seeds was 99.2%. All reagents used were of analytical grade. DPPH (Sigma-Aldrich Company, Germany), TPTZ (Sigma-Aldrich Company, Germany), Folin–Ciocalteau (F-C) reagent (Finar Limited, India), gallic acid (LOBA Chemie, India), ascorbic acid (Fisher Scientific, India), and ferrous sulfate (Fisher Scientific, India) were used.
Microgreens cultivation
Seeds were sterilized by soaking in 2.0% aqueous sodium hypochlorite for 15 min at room temperature (20 ±2°C) and then rinsed with tap water for 15 min. The rinsed seeds were spread in perforated fiber trays containing a 5 cm thick homogenous mixture of wet cocopeat and organic manure (1:1) and covered about 1 cm with the same mixture. Adequate water was added until the excess water drained from the pores. After the period of emergence (3 days), adequate water was poured daily and the microgreens were grown for 3 weeks, inside the room at ambient conditions. The room was subjected to a diurnal cycle with fluctuations of natural temperature, humidity, and light. The maximum and minimum temperatures during growing days were 20 ± 3°C and 8 ± 2°C, respectively. To evaluate the reproducibility of results, cultivation was carried in two blocks with a gap of 20 days. Samples were harvested 1 cm above the soil on days 9, 11, 13, 15, 18, and 21 days after sowing and stored in a freezer (−18 ±2°C) before evaluations.
Pigments
Fresh microgreens (1 g) were macerated and stirred in vortex for 10 min with 10% (w/v) of 80% (v/v) acetone–water for chlorophylls (CHLs) and carotenoids (CARs) extraction and with 20% (v/v) methanol–water for betalains extraction. The mixture was centrifuged at 7000 rpm for 10 min and the supernatants were filtered through Whatman No. 1 filter paper. The filtrate was made to 25 ml volume with respective solvents, viz. 80% (v/v) acetone–water for CHLs and CARs, and 20% (v/v) methanol–water for betalains. The absorbance of solution mixture at corresponding wavelengths was analyzed in UV-VIS spectrophotometer, and the concentration was determined using the CHLs equations[Citation19] and CARs equation,[Citation20] and Betalains equations[Citation21] as follows:
CHL a = 12.21 (A663) – 2.81 (A646)
CHL b = 20.13 (A646) – 5.03 (A663)
Total CHLs = CHL a + CHL b
Total CARs = 4.69 (A440.5) – 0.268 (Total CHLs)
β-Cyanins (µg/ml) = 550 (A538)/60000
β-Xanthins (µg/ml) = 308 (A480)/48000
Total betalains (µg/ml) =β-Cyanins + β-Xanthins
where A refers to the absorbance reading at the corresponding wavelengths (440.5, 480, 538, 646, and 663 nm). They were further expressed as follows:
mg Pigment/100 g DM = (µg/ml) × volume makeup/(10 × dry weight of microgreens)
Ascorbic acid
Total ascorbic acid was determined according to the procedure described by Niroula et al. [Citation12] Fresh microgreens (1 g) was macerated and extracted with 10 mL of ice-cold metaphosphoric acid {5% (w/v) in 10% (w/v) acetic acid} by vortexing for 3 min. Homogenized tissue was centrifuged at 7000 rpm for 10 min, and the supernatant was filtered through Whatman No. 1 filter paper. One miililiter of 2,6-dichlorophenol indophenols (0.02% w/v) was added to 2 ml of sample to oxidize the ascorbic acid to dehydroascorbic acid. Two millilitres of thiourea (2% w/v) and 1 ml of 2,4-dinitrophenylhydrazine (2% in 9 N H2SO4) were successively added and incubated at 50°C for 70 min with frequent stirring. After transferring to an ice bath 4 ml of 85% H2SO4 was added, mixed by gentle shaking, and allowed to stand for 30 min. The absorbance was taken at 520 nm. A typical calibration plot (R2 ≥0.99) was prepared with different concentrations of standard ascorbic acid and used to determine the ascorbic acid content in investigated samples.
Total phenolics content (TPC)
For the determination of TPC, extraction of beet microgreens was carried out as described by Niroula et al.[Citation6] with some modifications. Briefly, fresh microgreens (1 g) were macerated and extracted with 10 ml of 80% methanol with a magnetic stirrer for 4 h at 20°C. The extracts were filtered through Whatman No.1 filter paper, and volume make up was done to make a 50 ml stock solution with 80% methanol. The stock solution was kept at 4 ±2°C until evaluations; all evaluations were done on the same day. From the stock solution, working solutions of different concentrations were prepared.
TPC was determined by using F-C reagent spectrophotometrically as described earlier[Citation6,Citation22] with minor modifications. Briefly, 0.5 ml methanolic extract was mixed with 2 ml of 10% F-C reagent. After 5 min, 2 ml of sodium carbonate (7.5%, w/v) and 2 ml of distilled water was added, mixed, and incubated at 45°C for 45 min. The absorbance was measured using a UV-VIS spectrophotometer at 765 nm. A typical calibration plot (R2 ≥0.99) was prepared with gallic acid as standard (0.001–0.006 mg/ml) and used to determine the TPC in investigated samples as gallic acid equivalent (GAE).
Equivalent antioxidant capacity (EAC)
The antioxidant capacities of beet microgreens were evaluated by two analytical assays: FRAP and DPPH assay. The same stock solution prepared for the determination of TPC was used.
DPPH assay
Free radical scavenging activity of beet microgreens was measured in vitro by DPPH assay as described previously[Citation6] with some modifications. Briefly, test aliquots (0.4 ml) of various concentrations (12.5–800 µg/ml) of sample extracts were mixed with 3.6 ml of methanolic DPPH solution of 0.004% (w/v). After incubation in dark for 30 min at room temperature, the absorbance was measured at 517 nm against a blank (80% v/v methanol replacing the methanolic extracts). For comparison, different concentrations of ascorbic acid (0–25 μg/ml) was used as standard and evaluated.
The results are expressed in terms of ascorbic acid equivalent antioxidant capacity (AEAC) of the dry weight of samples required to scavenge 50% of DPPH free radical (i.e. IC50 values) calculated from the non-linear regression analysis of DPPH scavenging percentage against the concentration of samples and ascorbic acid standard. The DPPH scavenging percentage by samples and standard was calculated using the equation:
DPPH radical scavenging (%) =[(AbsBlank – AbsSample)/AbsBlank] x 100
where AbsBlank is the absorbance when solvent (80% v/v methanol) replaced samples/standard and AbsSample is the absorbance with different concentrations of plant extract and AA.
FRAP assay
FRAP assay of the extracts was measured as described by Benzie & Strain[Citation23] with some modifications. Fresh FRAP working reagent was prepared by mixing 300 mM acetate buffer (pH 3.6), 10 mM 2,4,6-tripyridyl-triazine (TPTZ) in the acetate buffer, and 20 mM FeCl3.H2O in the ratio 10:1:1. Freshly prepared FRAP reagent was warmed to 37°C and mixed with various dilutions of stock solution (prepared for TPC) in the ratio 9:1 and allowed to react in dark for 30 min at room temperature. The absorbance was measured using a UV-VIS spectrophotometer at 593 nm. Ferrous sulfate (FeSO4.7H2O) was used as standard and ascorbic acid as the positive control. The results are expressed as mM AEAC/100 g dry matter.
Statistical analysis
All analyses were carried out in triplicates, in microgreens cultivated in two blocks. The data were analyzed by one-way analysis of variance (ANOVA) and the sample means were compared by the Tukey HSD test where necessary. P-value <0.05 was considered significant in all cases. Pearson’s correlation was used to study the correlation and the regression analysis was carried out by response surface methodology. The JMP 15 software was used for statistical analysis.
RESULTS AND DISCUSSION
Pigments content
The pigments analyzed were total CHLs (CHL a + CHL b), total CARs, and total betalains (betacyanins + betaxanthins). The changes in pigment content during growth are illustrated in . Betalains were the most abundant pigment, followed by CHLs, while CARs content was the least. Statistical analysis showed that all pigments were significantly (p < 0.05) affected by the number of days of growth. The contents of pigments significantly (p < 0.05) increased in initial days of growth and reached the maximum on day 15. A slight reduction was observed on days 18 and 21 in the case of CHLs and CARs, but the reductions were not statistically significant (p > 0.05). The increase in the CHLs and CARs content up to day 15 was also reported for wheat and barley microgreens.[Citation16] They further reported that, although the contents of CHLs and CARs were increased, the rate of accumulation decreased after day 10 for wheat microgreens and after day 13 for barley microgreens. An initial significant increase in the CHLs and CARs concentration up to the second week, no significant change in the third week, and a significant reduction from the fourth week were reported in the previous study of Kale.[Citation24] Betalains also followed similar kinetics to CHLs and CARs, but the reduction after day 15 was statistically significant (p < 0.05). Previous reports on the change in betalains during the growth of microgreens were not available. CHLs content in leaves of Arabidopsis thaliana was also reported to exhibit a sharp increase from day 7 to 14 followed by a very high reduction from day 14 to 21.[Citation25] The rate of pigment accumulation is an indication of the maximum activities of biosynthetic enzymes and energy requirement by the cell which also signifies the rapid production of phenolics and other antioxidants.[Citation16] Pigments content in plants not only have health benefits but also reflect on the appearance of microgreens.[Citation26] CHLs in microgreens offer various shades of greenness,[Citation26] CARs contribute to yellow, orange, and red color, while betalains impart yellow-orange (betaxanthins), and red-violet (betacyanins) colors.[Citation27,Citation28]
Table 1. Changes in the contents of pigments in beet microgreens during different days of growth.a
The ratio of CHL a and CHL b (CHL a/b) was maximum at the youngest age (day 9) and gradually decreased with increasing days of growth (). Nath et al.[Citation25] also reported a gradual decrease in the CHL a/b ratio with increasing age of leaves. CHL a/b ratio means ranged between 2.32 and 2.48, which is comparable to wheatgrass (2.47 ± 0.38) grown in a greenhouse[Citation29] and within the range of crops grown in shades or low-light (2.3–2.8), but lower than those grown in open-field conditions or sunlight (2.9–3.8).[Citation30] The CHL a/b ratio in this study was higher than 1.30–2.15 reported in microgreens of the vegetable species (spinach, carrot, mustard, radish, roselle, and onion), leguminous species (fenugreek), oleagineous species (sunflower), and aromatic species (French basil and fennel)[Citation26]; however, the growth temperature and light conditions were not specified. The warmer temperature was also reported to result in a high CHL a/b ratio.[Citation31] CHL b is more resistant to thermal degradation[Citation32] and was reported to have high in vitro enterocytic absorption,[Citation33] and therefore CHLs in microgreens grown in daylight or cooler temperature having low CHL a/b ratio is more bioaccessible than those grown in sunlight which has higher CHL a/b ratio.[Citation16]
The CHL/CAR ratio significantly (p < 0.05) increased in the initial growth stage of microgreens, but after day 15 no significant difference (p > 0.05) was observed. The increasing CHL/CAR ratio (2.54 to 4.46) in the initial days signifies a higher rate of chlorophyll synthesis and accumulation compared to carotenoids. The stable CHL/CAR ratio after day 15 was due to lesser changes in the contents of CHLs and CARs. The values are in contradiction to the concept of low CHL/CAR ratio (3.6–4.6) at high light irradiance and high CHL/CAR ratio (4.8–7.0) in low-light irradiance or growth in shades, [Citation30] and very high ratio (5.9–11.5) in other microgreens of the rocket, sweet chard, basil, wheat, barley and amaranth.[Citation16,Citation34,Citation35] However, the results were very close to that reported for beet microgreens (4.25–4.62; day 14) and parsley (4.04–4.92; day 13) grown under blue light dosage (0–33%).[Citation36] This suggests that the CHL/CAR ratio in beet microgreens is difficult to generalize in terms of the level of irradiation. In a study of six different microgreen species, it was revealed that the bioactive components that are high in microgreens as compared to mature counterparts are unique to the microgreen species.[Citation15]
Betacyanins were twice (1.88–2.06) in contents than betaxanthins. Betacyanins/betaxanthin gradually decreased with the days of growth of microgreens. The previous report on betacyanins/betaxanthin ratio in beet microgreens was not found, but in various cultivars of red beetroots, the ratio was reported to decrease with increasing maturity; from 2.5 at an early stage to about 1 in the late harvest period,[Citation27] while in some varieties of yellow-beet, betacyanins were reported to be absent.[Citation37] In various genotypes of amaranths of 30 days of age, the betacyanins/betaxanthin ratio was approximately 1.[Citation38] Betacyanins are red-violet pigments and more stable against pH and temperature.[Citation39] Therefore, a high betacyanins/betaxanthin ratio is favorable for its red-violet hue and better stability against pH and temperature compared to those with lower ratio values.
On the ninth day, total betalains content was about 6.04-fold and 15.33-fold of total CHLs and total CARs content, respectively; the ratios however significantly (p < 0.05) decreased with the number of days of growth, because of the relatively higher percentage of CHL and CAR synthesis and accumulation compared to betalains. The betalains/CHL ratio reached 1.95 ± 0.03 on day 21, which is in the range of 1–2.6 in different genotypes of amaranthus on day 30.[Citation38]
Ascorbic acid content
The ascorbic acid content of beet microgreens was significantly (p < 0.05) affected by days of growth. The ascorbic acid contents in beet microgreens were least on day 9 (710.97 ± 20.24 mg/100 g DM) and maximum on day 15 (1083.30 ± 30.73 mg/100 g DM) (). The reduction in ascorbic acid content after day 15 was comparatively slower than the rate at which it increased in the initial days. The initial increase in ascorbic acid content in microgreens was associated with the reactivation of enzyme l-galactono-1,4-lactone dehydrogenase, which catalyzes the last enzymatic step of ascorbic acid biosynthesis; while the decrease after day 15 could be due to the onset of the conversion of ascorbic acid to tartarate in the presence of l-idonate dehydrogenase, or C2/C3 cleavage into oxalate and l-threonate.[Citation12,Citation40] On a fresh matter (FM) basis, ascorbic acid content was in the range of 35–51 mg/100 g FM, the mean of day 15 being 51.10 ± 2.26 mg/100 g FM. This content of ascorbic acid is very high as a vitamin and can solely fulfill the recommended daily allowance (RDA) of 60 mg/day for vitamin C with about 120 g FM of beet microgreens (day 15). This result is comparable to 46.4 ± 3.0 mg/100 g FM (DM = 6.2 ± 0.1%; day not specified),[Citation3] and 55 ± 4 mg/100 g FM (day 14),[Citation41] but lower than 73 ± 8 mg/100 g FM beet microgreens on day 14 at low UV-irradiance.[Citation42] However, other red beet variety microgreens were reported to have significantly low ascorbic content 28.8 ± 0.4 mg/100 g FM (DM = 4.6 ± 0.1%; day not specified) in the study of Xiao et al.[Citation3] These values are higher than the microgreens of mustard (30.67 ± 1.02 mg/100 g FM) and comparable to that of radish (45.43 ± 1.15 mg/100 g FM), broccoli (50.99 ± 1.91 mg/100 g FM), and kale (56.14 ± 1.04 mg/100 g FM) as reported by De la Fuente et al.[Citation2]
Figure 1. Beet microgreens attributes on dry matter (DM) basis on different days of growth. (a) ascorbic acid content, (b) total polyphenols content as gallic acid equivalent (GAE), (c) antioxidant activity via DPPH assay as ascorbic acid equivalent antioxidant capacity (AEAC), (d) antioxidant activity via FRAP assay as AEAC. Data are expressed as the mean ± SD (block = 2, n = 2 × *3). Different lowercase alphabets indicate significant differences (p < 0.05) among samples within the category
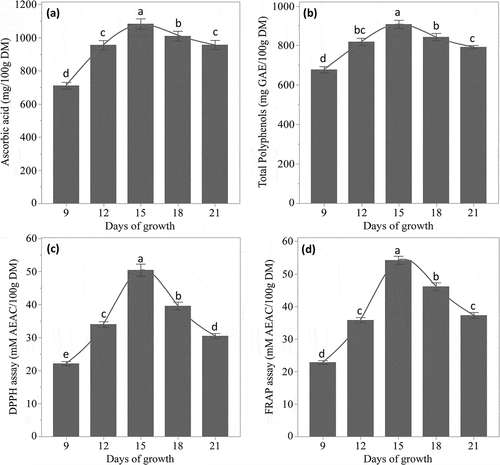
Total polyphenols content (TPC)
The TPC of beet microgreens was significantly (p < 0.05) affected by days of growth. The change in TPC during growth was similar to pigments and ascorbic acid, but the contents were lower than ascorbic acid and betalains and higher than chlorophylls and carotenoids (). An initial increase in TPC to reach the maximum on the second week of growth of microgreens was also reported in wheat and barley microgreens.[Citation6,Citation43] The initial increase in TPCs of microgreens was probably due to the stimulation of phenylpropanoid pathways[Citation44] and subsequent reduction may be associated with the accumulation of TPCs that auto-regulate the enzymes responsible for their biosynthesis, for example, phenylalanine ammonia-lyase (PAL) responsible for the biosynthesis of hydroxycinamic acids.[Citation12,Citation45] An apparent reduction may also be due to the rapid development of non-phenolic structural components in microgreens as compared to TPCs.[Citation6,Citation12] Further, phenolic components may polymerize and/or cross-linking into cell walls structures, which could make them less extractible with organic solvents.[Citation6,Citation46]
TPC in beet microgreens was least on day 9 (678.01 ± 16.10 mg GAE/100 g DM) which significantly (p < 0.05) increased on each evaluating day until maximum on day 15 (908.16 ± 20.65 mg GAE/100 g DM) and again decreased significantly (p < 0.05). This content is more than double of the value; 300 ± 10 mg GAE/100 g DM reported in bull’s blood beet microgreens by Xiao et al.,[Citation8] but comparable to 38–47 mg GAE/100 g FM in beet microgreens treated with supplement short-term red LED lightings. On the FM basis, TPCs in this study ranged from 33.24 ± 1.91 to 42.84 ± 1.76 mg GAE/100 g FM. These values are higher than green cultivars (25.00 ± 2.63 mg GAE/100 g FM), comparable to red cultivars (28.47 ± 4.35 mg GAE/100 g FM) and lower than katwa cultivars (48.55 ± 0.72 mg GAE/100 g FM) of amaranthus.[Citation47] The TPC was also lower than other microgreens like Brussels sprouts (1.92 ± 0.24 mg GAE/g FM),[Citation48] broccoli (1.06 ± 0.12 mg GAE/g FM),[Citation48] pakchoi (0.78 ± 0.16 mg GAE/g FM),[Citation48] mustard (1889.76 ± 64.81 mg GAE/100 g DM),[Citation2] kale (215.95 ± 109.34 mg/100 g DM),[Citation2] radish (135.74 ± 0.21 mg GAE/100 g FM),[Citation47] palak (60.00 ± 4.21 mg GAE/100 g FM),[Citation47] and water spinach (121.44 ± 5.00 mg GAE/100 g FM),[Citation47] wheat (1290.51 ± 56.45 mg GAE/100 g DM),[Citation6] and barley (1325.50 ± 58.14 mg GAE/100 g DM).[Citation6]
Equivalent antioxidant capacity (EAC)
EAC was evaluated by two assays: DPPH assay and FRAP assay. EACs obtained from both assays followed a similar profile. EACs significantly increased (p < 0.05) during the initial growth stage until day 15, after which a significant decline (p < 0.05) was observed. This initial rise in EACs of microgreens could be attributed to the biosynthesis and accumulation of pigments, ascorbic acid, TPCs, and other antioxidative components in the initial days of growth, while the subsequent decline in EACs of microgreens could be attributed to the decrease in the most antioxidative components, i.e. betalains and TPCs. EAC is the sum of antioxidant capacities of all the antioxidative components present in a sample.[Citation12]
DPPH assay
For the DPPH assay, microgreens required to scavenge 50% of DPPH radical present in a solution were evaluated and compared to the same by ascorbic acid. Results are expressed as mM AEAC/100 g DM microgreens (). EAC of samples significantly (p < 0.05) increased on each evaluation from day 9 (22.16 ± 0.61 mM AEAC/100 g DM) until the maximum on day 15 (52.64 ± 1.32 mM AEAC/100 g DM) followed by significant (p < 0.05) reduction. EACs in fresh matter ranged from 1.09 ± 0.07 to 2.38 ± 0.10 mM AEAC/100 g FM and is higher than that reported for wheatgrass (0.2–0.8 mM AEAC/100 g FM at different growth conditions),[Citation11] different cultivars of amaranths (0.08–0.28 mM Trolox EAC/100 g FM)[Citation47] and even higher than those that had higher TPC than beet microgreens, namely palak (0.12 ± 0.02 mM Trolox EAC/100 g FM),[Citation47] water spinach (0.34 ± 0.07 mM Trolox EAC/100 g FM),[Citation47] and radish (0.21 ± 0.08 mM Trolox EAC/100 g FM).[Citation47] Despite low TPC, comparatively high EAC of beet microgreens are associated with betalains, which were higher than TPC and more anti-oxidative. Betalains were reported to have excellent antioxidant activity, 3–7.5 times of ascorbic acid, 1.5–2.0 times of anthocyanins, and much higher than rutin and catechin during in vitro studies.[Citation17,Citation49,Citation50] Therefore, betalains were the principal component in beet microgreens determining their antioxidant activity.
FRAP assay
For FRAP assay, results are expressed as mM AEAC/100 g DM microgreens (). Minimum antioxidant capacity was observed on day 9 (22.83 ± 0.56 mM AEAC/100 g DM) which significantly (p < 0.05) increased with days of growth until day 15 (54.19 ± 1.23 mM AEAC/100 g DM) and decreased afterward. This result in fresh matter ranged from 1.12 ± 0.06 to 2.56 ± 0.18mMAEAC/100 g FM. According to Benzie and Strain[Citation23] the stoichiometric factors of Trolox, and ascorbic acid, were 2 for FRAP assay. When compared with this consideration, the antioxidant power of beet microgreens was higher than that of different cultivars of amaranthus (0.21–0.50 mM Trolox EAC/100 g FM),[Citation47] wheatgrass (0.12–0.40 mM Trolox EAC/100 g FM)[Citation11] and even higher than those that had higher TPC than beet microgreens, namely palak (0.67 ± 0.05 mM Trolox EAC/100 g FM),[Citation47] and water spinach (1.02 ± 0.08 mM Trolox EAC/100 g FM).[Citation47]
Correlation coefficients
Correlation coefficients among pigments, ascorbic acid, total polyphenols, and antioxidant capacity in beet microgreens during different days of growth were evaluated in a row-wise manner (). A high correlation (R2> 0.75) existed among all the evaluated parameters. A good correlation among CHLs, CARs, total polyphenols, and antioxidant capacities has been mentioned previously.[Citation6,Citation11,Citation16,Citation47] In the study of 10 microgreens, correlations between ascorbic acid content and antioxidant capacity were non-significant.[Citation47] A good correlation in our study was possibly due to the study of changes during growth in only one type of microgreen. Although no reports were found on the correlation of betalains with TPC and antioxidant activities in microgreens, studies in beetroot and colored quinoa have shown a good positive correlation among the betalains (betacyanins and betaxanthins) content, TPC, and antioxidant activity.[Citation51,Citation52]
Table 2. Multivariate correlation coefficients among pigments, ascorbic acid, total polyphenols, and antioxidant capacity in beet microgreens during different days of growth.a
Regression evaluations
Based on very high correlations of betacyanins and betaxanthins to other parameters, regression evaluation was carried out by response surface methodology with betacyanins and betaxanthins as the factors and other investigated parameters as the response. The evaluations revealed that chlorophyll content, DPPH assay, and FRAP assay were quadratically affected by both betacyanins and betaxanthins, and further the effects betacyanins and betaxanthins were interacting. The interaction effect refers to the effect of betacyanins on these parameters is affected by the contents of betaxanthins and vice versa. Similarly, betaxanthins had linear and betacyanins had quadratic effects on total chlorophylls, total carotenoids, and ascorbic acid contents. Polyphenols were however unaffected by betacyanins content and linearly affected by betaxanthin alone. The R2 for all responses was 0.98 or more. The regression expressions for each parameter are presented below:
where a, b, c, d, and e are coefficients of individual parameters and the coefficients including constant are dependent on individual response as presented in .
Table 3. Regression coefficients for total chlorophylls, total carotenoids, ascorbic acid, total polyphenols, and antioxidant capacities of beet microgreens as the function of betaxanthins (BX) and betacyanins (BC).a
Conclusion
This study for the first time reports comprehensive profiling of pigments, ascorbic acid, polyphenols, and antioxidant capacities of beet microgreens during different days of growth. In addition to replicating previous findings, this study has charted the profile of pigments, ascorbic acid, TPCs, and EACs levels at different numbers of days of growth. All investigated parameters were minimum on the ninth day and reached the maximum on the fifteenth day. No significant reductions were observed in CHLs and CARs, all other parameters were reduced after day 15. Ascorbic acid was present in a relevant amount to fulfill RDA, and despite low TPC, beet microgreens possessed high EACs as compared to studies in other microgreens. This was because of betalains, whose contents and antioxidative activities were higher than other pigments, ascorbic acid, and TPCs. Pearson correlation evaluation revealed a very high correlation among all evaluated parameters. Betaxanthins and betacyanins had high corretaions with all investigated parameters and were used to formulate regression expression for each response. This study mainly focused on quantification during growth, which demonstrates the importance of beet microgreens as natural health supplements. Potential bioactivity in cell-based and human intervention studies in the future would draw valuable information related to human health.
Acknowledgments
Authors would like to thank Nagarik College, Tribhuvan University, Nepal for the internal grant.
References
- Choe, U.; Yu, L.; Wang, T. T. Y. The Science behind Microgreens as an Exciting New Food for the 21th Century. J. Agric. Food Chem. 2018, 66, 11519–11530. DOI: https://doi.org/10.1021/acs.jafc.8b03096.
- De la Fuente, B.; López-García, G.; Máñez, V.; Alegría, A.; Barberá, R.; Cilla, A. Evaluation of the Bioaccessibility of Antioxidant Bioactive Compounds and Minerals of Four Genotypes of Brassicaceae Microgreens. Foods. 2019, 8, 250. DOI: https://doi.org/10.3390/foods8070250.
- Xiao, Z.; Lester, G. E.; Luo, Y.; Wang, Q. Assessment of Vitamin and Carotenoid Concentrations of Emerging Food Products: Edible Microgreens. J. Agric. Food Chem. 2012, 60(31), 7644–7651. DOI: https://doi.org/10.1021/jf300459b.
- Xiao, Z.; Rausch, S. R.; Luo, Y.; Sun, J.; Yu, L.; Wang, Q.; Chen, P.; Yu, L.; Stommel, J. R. Microgreens of Brassicaceae: Genetic Diversity of Phytochemical Concentrations and Antioxidant Capacity. LWT - Food Sci. Technol. 2019, 101, 731–737. DOI: https://doi.org/10.1016/j.lwt.2018.10.076.
- Kyriacou, M. C.; Rouphael, Y.; Di Gioia, F.; Kyratzis, A.; Serio, F.; Renna, M.; De Pascale, S.; Santamaria, P.; Di, G. F.; Kyratzis, A.; et al. Micro-scale Vegetable Production and the Rise of Microgreens. Trends Food Sci. Technol. 2016, 9, 103–115. DOI: https://doi.org/10.1016/j.tifs.2016.09.005.
- Niroula, A.; Khatri, S.; Khadka, D.; Timilsina, R. Total Phenolic Contents and Antioxidant Activity Profile of Selected Cereal Sprouts and Grasses. Int. J. Food Prop. 2019, 22, 427–437. DOI: https://doi.org/10.1080/10942912.2019.1588297.
- Mir, S. A.; Shah, M. A.; Mir, M. M. Microgreens: Production, Shelf Life and Bioactive Components. Crit. Rev. Food Sci. Nutr. 2016, 57(12), 2730–2736. DOI: https://doi.org/10.1080/10408398.2016.1144557.
- Xiao, Z.; Lester, G. E.; Park, E.; Saftner, R. A.; Luo, Y.; Wang, Q. Evaluation and Correlation of Sensory Attributes and Chemical Compositions of Emerging Fresh Produce: Microgreens. Postharvest Biol. Technol. 2015, 110, 140–148. DOI: https://doi.org/10.1016/j.postharvbio.2015.07.021.
- Treadwell, D. D.; Hochmuth, R.; Landrum, L.; Laughlin, W. Microgreens: A New Specialty Crop. Univ. Florida IFAS Ext. 2010, HS1164.
- Kyriacou, M. C.; De, P. S.; Kyratzis, A.; Rouphael, Y. Microgreens as A Component of Space Life Support Systems: A Cornucopia of Functional Food. Front. Plant Sci. 2017, 8. DOI: https://doi.org/10.3389/fpls.2017.01587.
- Kulkarni, S. D.; Tilak, J. C.; Acharya, R.; Rajurkar, N. S.; Devasagayam, T. P. A.; Reddy, A. V. R. Evaluation of the Antioxidant Activity of Wheatgrass (Triticum Aestivum L.) As a Function of Growth under Different Conditions. Phyther. Res. 2006, 227, 218–227. DOI: https://doi.org/10.1002/ptr.1838.
- Niroula, A.; Amgain, N.; Kc, R.; Adhikari, S.; Acharya, J. Pigments, Ascorbic Acid, Total Polyphenols and Antioxidant Capacities in Deetiolated Barley (Hordeum Vulgare) and Wheat (Triticum Aestivum) Microgreens. Food Chem. 2021, 354, 129491. DOI: https://doi.org/10.1016/j.foodchem.2021.129491.
- Kou, L.; Luo, Y.; Yang, T.; Xiao, Z.; Turner, E. R.; Lester, G. E.; Wang, Q.; Camp, M. J. Postharvest Biology, Quality and Shelf Life of Buckwheat Microgreens. LWT - Food Sci. Technol. 2013, 51(1), 73–78. DOI: https://doi.org/10.1016/j.lwt.2012.11.017.
- Ghoora, M. D.; Babu, D. R.; Srividya, N. Nutrient Composition, Oxalate Content and Nutritional Ranking of Ten Culinary Microgreens. J. Food Compos. Anal. 2020, 91, 103495. DOI: https://doi.org/10.1016/j.jfca.2020.103495.
- Johnson, S. A.; Prenni, J. E.; Heuberger, A. L.; Isweiri, H.; Chaparro, J. M.; Newman, S. E.; Uchanski, M. E.; Omerigic, H. M.; Michell, K. A.; Bunning, M.; et al. Comprehensive Evaluation of Metabolites and Minerals in 6 Microgreen Species and the Influence of Maturity. Curr. Dev. Nutr. 2021, 5. DOI: https://doi.org/10.1093/cdn/nzaa180.
- Niroula, A.; Khatri, S.; Timilsina, R.; Khadka, D.; Khadka, A.; Ojha, P. Profile of Chlorophylls and Carotenoids of Wheat (Triticum Aestivum L.) And Barley (Hordeum Vulgare L.) Microgreens. J. Food Sci. Technol. 2019, 56, 2758–2763. DOI: https://doi.org/10.1007/s13197-019-03768-9.
- Gengatharan, A.; Dykes, G. A.; Choo, W. S. Betalains: Natural Plant Pigments with Potential Application in Functional Foods. LWT - Food Sci. Technol. 2015, 64, 645–649. DOI: https://doi.org/10.1016/j.lwt.2015.06.052.
- Mastrangelo, D.; Massai, L.; Fioritoni, G.; Lo Coco, F.; Noguera, N.; Testa, U. High Doses of Vitamin C and Leukemia: In Vitro Update. In Myeloid Leukemia; Lasfer, A., Ed.; InTech: London, UK, 2018. DOI: https://doi.org/10.5772/intechopen.71484.
- Lichtenthaler, H. K.; Wellburn, A. R. Determinations of Total Carotenoids and Chlorophylls a and b of Leaf Extracts in Different Solvents. Biochemical Society Transactions; 11, 1983; pp 591–592. DOI:https://doi.org/10.1042/bst0110591.
- Holm, G.;. Chlorophyll Mutations in Barley. Acta Agric. Scand. 1954, 4, 457–471. DOI: https://doi.org/10.1080/00015125409439955.
- Stintzing, F. C.; Schieber, A.; Carle, R. Evaluation of Colour Properties and Chemical Quality Parameters of Cactus Juices. Eur. Food Res. Technol. 2003, 216(4), 303–311. DOI: https://doi.org/10.1007/s00217-002-0657-0.
- Singleton, V. L.; Orthofer, R.; Lamuela-Raventós, R. Analysis of Total Phenols and Other Oxidation Substrates and Antioxidants by Means of Folin-ciocalteu Reagent. Oxid. Antioxidants Part A. 1999, 299, 152–178. DOI: https://doi.org/10.1016/S0076-6879(99)99017-1.
- Benzie, I. F. F.; Strain, J. J. The Ferric Reducing Ability of Plasma (FRAP) as a Measure of “Antioxidant Power”: The FRAP Assay. Anal. Biochem. 1996, 239, 70–76. DOI: https://doi.org/10.1006/abio.1996.0292.
- Lefsrud, M.; Kopsell, D.; Wenzel, A.; Sheehan, J. Changes in Kale (Brassica Oleracea L. Var. Acephala) Carotenoid and Chlorophyll Pigment Concentrations during Leaf Ontogeny. Sci. Hortic. (Amsterdam). 2007, 112, 136–141. DOI: https://doi.org/10.1016/j.scienta.2006.12.026.
- Nath, K.; Phee, B. K.; Jeong, S.; Lee, S. Y.; Tateno, Y.; Allakhverdiev, S. I.; Lee, C. H.; Nam, H. G. Age-dependent Changes in the Functions and Compositions of Photosynthetic Complexes in the Thylakoid Membranes of Arabidopsis Thaliana. Photosynth. Res. 2013, 117, 547–556. DOI: https://doi.org/10.1007/s11120-013-9906-2.
- Ghoora, M. D.; Haldipur, A. C.; Srividya, N. Comparative Evaluation of Phytochemical Content, Antioxidant Capacities and Overall Antioxidant Potential of Select Culinary Microgreens. J. Agric. Food Res. 2020, 2, 100046. DOI: https://doi.org/10.1016/j.jafr.2020.100046.
- Stintzing, F. C.; Herbach, K. M.; Mosshammer, M. R.; Kugler, F.; Carle, R. Betalain Pigments and Color Quality. In ACS Symposium Series; American Chemical Society, Washington, DC, US, 2008; Vol. 983, pp. 82–101.
- De Azeredo, H. M. C.; Pereira, A. C.; De Souza, A. C. R.; Gouveia, S. T.; Mendes, K. C. B. Study on Efficiency of Betacyanin Extraction from Red Beetroots. Int. J. Food Sci. Technol. 2009, 44, 2464–2469. DOI: https://doi.org/10.1111/j.1365-2621.2009.02037.x.
- Shah, S. H.; Houborg, R.; Mccabe, M. F. Response of Chlorophyll, Carotenoid and SPAD-502 Measurement to Salinity and Nutrient Stress in Wheat (Triticum Aestivum L .). Agronomy. 2017, 7, 61. DOI: https://doi.org/10.3390/agronomy7030061.
- Lichtenthaler, H. K.;. Biosynthesis, Accumulation and Emission of Carotenoids, a -tocopherol, Plastoquinone, and Isoprene in Leaves under High Photosynthetic Irradiance. Photosynth. Res. 2007, 92, 163–179. DOI: https://doi.org/10.1007/s11120-007-9204-y.
- Haldimann, P.;. How Do Changes in Temperature during Growth Affect Leaf Pigment Composition and Photosynthesis in Zea Mays Genotypes Differing in Sensitivity to Low Temperature? J. Exp. Bot. 1999, 50, 543–550. DOI: https://doi.org/10.1093/jxb/50.333.543.
- Okpalanma, F. E.; Ojimelukwe, P. C. Evaluation of Effects of Storage Condition and Processing on Carotenoids, Chlorophyll, Vitamins and Minerals in a Water Leaf (Talinum Triangulare). Asian Food Sci. J. 2018, 2, 41603. DOI: https://doi.org/10.9734/AFSJ/2018/41603.
- Chen, K.; Roca, M. In Vitro Bioavailability of Chlorophyll Pigments from Edible Seaweeds. J. Funct. Foods. 2018, 41, 25–33. DOI: https://doi.org/10.1016/j.jff.2017.12.029.
- Bulgari, R.; Baldi, A.; Ferrante, A.; Lenzi, A. Yield and Quality of Basil, Swiss Chard and Rocket Microgreens Grown in a Hydroponic System Project LIFE Hortised-Demonstration of the Suitability of Dredged Remediated Sediments for Safe and Sustainable Horticulture Production LIFE ENV/IT/000113 View Pro. Artic. New Zeal. J. Crop Hortic. Sci. 2016, 45(2), 119–129. DOI: https://doi.org/10.1080/01140671.2016.1259642.
- Khanam, U. K. S.; Oba, S. Bioactive Substances in Leaves of Two Amaranth Species, Amaranthus Tricolor and A. Hypochondriacus. Can. J. Plant Sci. 2013, 93, 47–58. DOI: https://doi.org/10.4141/CJPS2012-117.
- Samuolienė, G.; Viršilė, A.; Brazaitytė, A.; Jankauskienė, J.; Sakalauskienė, S.; Vaštakaitė, V.; Novičkovas, A.; Viškelienė, A.; Sasnauskas, A.; Duchovskis, P. Blue Light Dosage Affects Carotenoids and Tocopherols in Microgreens. Food Chem. 2017, 228, 50–56. DOI: https://doi.org/10.1016/j.foodchem.2017.01.144.
- Stintzing, F. C.; Schieber, A.; Carle, R. Identification of Betalains from Yellow Beet (Beta Vulgaris L.) And Cactus Pear [Opuntia Ficus-indica (L.) Mill.] By High-Performance Liquid Chromatography−Electrospray Ionization Mass Spectrometry. J. Agric. Food Chem. 2002, 50 2302–2307. DOI: https://doi.org/10.1021/jf011305f.
- Sarker, U.; Oba, S.; Elfalleh, W. Protein, Dietary Fiber, Minerals, Antioxidant Pigments and Phytochemicals, and Antioxidant Activity in Selected Red Morph Amaranthus Leafy Vegetable. PLoS One. 2019, 14, e0222517. DOI: https://doi.org/10.1371/journal.pone.0222517.
- Saguy, I.;. Thermostability of Red Beet Pigments (Betanine and Vulgaxanthinin): Influence of pH and Temperature. J. Food Sci. 1979, 44, 1554–1555. DOI: https://doi.org/10.1111/j.1365-2621.1979.tb06488.x.
- Melino, V. J.; Soole, K. L.; Ford, C. M. Ascorbate Metabolism and the Developmental Demand for Tartaric and Oxalic Acids in Ripening Grape Berries. BMC Plant Biol. 2009, 9, 145. DOI: https://doi.org/10.1186/1471-2229-9-145.
- Samuolienė, G.; Brazaitytė, A.; Sirtautas, R.; Sakalauskienė, S.; Jankauskienė, J.; Duchovskis, P.; Novičkovas, A. The Impact of Supplementary Short-term Red LED Lighting on the Antioxidant Properties of Microgreens. Acta Hortic. 2012, 956, 649–656. doi: https://doi.org/10.17660/ActaHortic.2012.956.78.
- Brazaitytė, A.; Viršilė, A.; Jankauskienė, J.; Sakalauskienė, S.; Samuolienė, G.; Sirtautas, R.; Novičkovas, A.; Dabašinskas, L.; Miliauskienė, J.; Vaštakaitė, V.; et al. Effect of Supplemental UV-A Irradiation in Solid-state Lighting on the Growth and Phytochemical Content of Microgreens**. Int. Agrophys. 2015, 29(1), 13–22. DOI: https://doi.org/10.1515/intag-2015-0004.
- Agrawal, A.; Gupta, E.; Chaturvedi, R. Determination of Minerals and Antioxidant Activities at Different Levels of Jointing Stage in Juice of Wheat Grass -the Green Wonder. Int. J. Pure Appl. Biosci. 2015, 3, 311–316.
- Randhir, R.; Shetty, K. Developmental Stimulation of Total Phenolics and Related Antioxidant Activity in Light- and Dark-germinated Corn by Natural Elicitors. Process Biochem. 2005, 40, 1721–1732. DOI: https://doi.org/10.1016/j.procbio.2004.06.064.
- Oh, M. M.; Carey, E. E.; Rajashekar, C. B. Regulated Water Deficits Improve Phytochemical Concentration in Lettuce. J. Am. Soc. Hortic. Sci. 2010, 135, 223–229. DOI: https://doi.org/10.21273/jashs.135.3.223.
- Mattila, P.; Kumpulainen, J. Determination of Free and Total Phenolic Acids in Plant-derived Foods by HPLC with Diode-array Detection. J. Agric. Food Chem. 2002, 50, 3660–3667. DOI: https://doi.org/10.1021/jf020028p.
- Yadav, L. P.; Koley, T. K.; Tripathi, A.; Singh, S. Antioxidant Potentiality and Mineral Content of Summer Season Leafy Greens: Comparison at Mature and Microgreen Stages Using Chemometric. Agric. Res. 2019, 8, 165–175. DOI: https://doi.org/10.1007/s40003-018-0378-7.
- Li, Z.; Lee, H.; Liang, X.; Liang, D.; Wang, Q.; Huang, D.; Ong, C. Profiling of Phenolic Compounds and Antioxidant Activity of 12 Cruciferous Vegetables. Molecules. 2018, 23, 1139. DOI: https://doi.org/10.3390/molecules23051139.
- Cai, Y.; Sun, M.; Corke, H. Antioxidant Activity of Betalains from Plants of the Amaranthaceae. J. Agric. Food Chem. 2003, 51, 2288–2294. DOI: https://doi.org/10.1021/jf030045u.
- Gliszczyńska-Świgło, A.; Szymusiak, H.; Malinowska, P. Betanin, the Main Pigment of Red Beet: Molecular Origin of Its Exceptionally High Free Radical-scavenging Activity. Food Addit. Contam. 2006, 23, 1079–1087. DOI: https://doi.org/10.1080/02652030600986032.
- Vulić, J.; Čanadanović-Brunet, J.; Ćetković, G.; Tumbas, V.; Djilas, S.; Četojević-Simin, D.; Čanadanović, V. Antioxidant and Cell Growth Activities of Beetroot Pomace Extracts. J. Funct. Foods. 2012, 4, 670–678. DOI: https://doi.org/10.1016/j.jff.2012.04.008.
- Abderrahim, F.; Huanatico, E.; Segura, R.; Arribas, S.; Gonzalez, M. C.; Condezo-Hoyos, L. Physical Features, Phenolic Compounds, Betalains and Total Antioxidant Capacity of Coloured Quinoa Seeds (Chenopodium Quinoa Willd.) From Peruvian Altiplano. Food Chem. 2015, 183, 83–90. DOI: https://doi.org/10.1016/j.foodchem.2015.03.029.