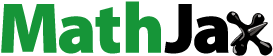
ABSTRACT
Rosa pimpinellifolia, commonly known as the burnet rose, has shown huge functional properties and industrial potential. In this present study, the convective drying process was optimized to enhance the quality, antioxidant activity, and phenolic compounds. The influence of drying conditions on the drying and rehydration kinetics and mathematical modeling of the R. pimpinellifolia fruit (black rose hips) were investigated. Response surface methodology was used to detect the optimum drying conditions (i.e. temperature and air velocity). The optimum operating conditions were 67.21°C and 1.75 m s−1 providing maximum total phenolic compounds, total anthocyanin, DPPH radical scavenging and Ferric Reducing Antioxidant Power (FRAP) values of 1327 ± 5 mg GAE/100 g, 491 ± 2 mg c3g E/100 g, 110 ± 2 mmol TE/g and 698.00 ± 15 mmol ISE/g, respectively. The Page Model was the most fitted model to predict the drying kinetics. Vegas-gálves and Peleg models were the most effective to describe the rehydration kinetics. The rehydration was more efficient at 60°C compared to 20 and 40°C. The convective drying increased hardness, cohesiveness, gumminess, and chewiness while decreasing springiness and resilience. Catechin, epicatechin, quercetin-3-glucoside, protocatechuic acid, chlorogenic acid, quercetin, rutin, fumaric acid, and gallic acid were the major phenolic compounds identified. The drying process thus improved the quality, antioxidant activity, and overall phenolic compounds of R. pimpinellifolia.
Introduction
As the COVID-19 pandemic has taken its worldwide toll in the past year, food bioactives have been suggested to support the immune system against COVID-19 and to promote overall health.[Citation1,Citation2] While seeking solutions for health and nutrition, new sources of food are being investigated. Plants and fruits provide fresh, healthy and nutritional food as well as farm-to-table employment. The genus Rosa of the Rosaceae family counts more than 150 species, mainly spread throughout West Asia, Anatolia, North-West Africa, North America, Northern, and Central Europe. Among these species, Rosa pimpinellifolia L. Syn. Rosa spinosissima L., commonly known as the burnet rose, produces a fruit known as black rose hips or Scottish rose hips. Rosa pimpinellifolia is a biennial, or perennial shrub cultivated for its fruit (black rose hips) which has been used to treat specific ailments for decades. Tea made from the dried fruit, leaves, and flowers of R. pimpinellifolia has long been used as a tonic, tranquilizer, diaphoretic and remedy to prevent or treat flu, cough, stress, sore throat, and pneumonia. In western medicine, rose fruit (rose hip) varieties have long been used for their anti-inflammatory, laxative, flu relief, menstrual cycle regulation, diuretic, and chronic pain reliever properties.[Citation3] Moreover, the fruit is processed into jams, marmalade, juices, syrups, vinegar, jellies, and wine. Previous studies revealed that R. pimpinellifolia fruit display high antioxidant capacity and contain many phytochemical compounds including carotenoids, tocopherols, flavonoids, phenolic acids, tripternoic acids, sugars, and organic acids.[Citation3] These compounds can be recovered using conventional or emerging technologies to be utilized as bioactive additives in food products and cosmetics.[Citation4–6]
As the first step in their application in tea, foods, pharmaceutical, and cosmetic industries and extraction purposes, R. pimpinellifolia fruit must be dried. The drying is typically performed with several systems such as conventional, convective, microwave, microwave-vacuum, pilot-scale heat pump conveyor, fluidized bed, oven, climate chamber, and solar-assisted drying. Different studies have investigated ways to reduce energy consumption and water content and preserve the nutritional and organoleptic properties of fruits and vegetables during drying.[Citation7,Citation8] To the best of our knowledge although published studies have revealed phytochemical compounds and health benefits of R. pimpinellifolia, the main impacts of convective drying on the quality of R. Pimpinellifolia have not been exploited yet. However, convective drying has been successfully performed to dry other fruits (e.g., strawberries) and has shown encouraging results.[Citation7]
In this regard, the present study aimed at modeling the drying of the fruit from R. pimpinellifolia. Response surface methodology (RSM) using a two-level central composite was applied in order to determine the optimum temperature and air velocity conditions of convective drying of R. pimpinellifolia fruit by determining different qualitative parameters phenolic content, total anthocyanins, and antioxidant activity. The drying and rehydration kinetics of the R. pimpinellifolia fruit were subjected to a number of existing mathematical models in order to determine the most adequate models. In addition, the drying process’s effects on the phenolic composition, physical and morphological characteristics of the fruit were evaluated.
Material and methods
Plant material and characterization
Fresh R. pimpinellifolia fruit was collected from the Erzurum province of Turkey in 2019. The impurities and spoiled fruit were removed before washing, draining, and storage at 4°C. Prior to the experiment, the fruit was removed from the refrigerator and let to stand until reaching room temperature (20°C). The fruit flesh to seed ratio (F/S) was carried out by weighing the flesh and seed separately and calculated the following Equationequation (1)(1)
(1) .
where Fw and Sw are the weights (g) of the fruit flesh and seed, respectively. The moisture content was measured using an oven at 70°C overnight. The color was determined by applying a colorimeter (DP- 400, Minolta, Japan) to measure L* (brightness: 0 = dark, 100 = white), a* (- green; + red) and b* (‐ blue; + yellow) values. Calibration was performed using a white tile (No. 19633162).
Chemicals
Distilled water was purified by a Millipore-Q system (Millipore Billerica, Massachusetts, USA). Methanol, 2,2- diphenyl-1-picrylhydrazyl (DPPH), Trolox, gallic acid, sodium carbonate, (-)-epicatechin, quercetin-3-glucoside, protocatechuic acid, chlorogenic acid, tannic acid, p-coumaric acid, rutin, naringenin, gallic acid, catechin, fumaric acid, and quercetin were provided by Sigma-Aldrich. A Folin Ciocalteu reagent was purchased from Merck.
Drying system
Drying was performed using a laboratory-scale cabinet dryer (EKSIS, Turkey) functioning in its convection mode Pashazadeh et al.[Citation9] have successfully used this drying system. The loss of moisture was recorded every 15 min.
Design and drying experiments
The drying of R. pimpinellifolia fruit included operation of the dryer at different temperatures and air velocities and design using Design Expert Software 9.0 (Trial version, Stat-EaseInc., Minneapolis, USA). An RSM with a central composite design was applied using two factors in order to identify the optimal temperature (°C) and air velocity (m s−1). A combination of independent variables such as temperature (55–84°C) and air velocity (0.5–1.95 m.s−1) was chosen for convective drying of the fruit (). Prior to the experiment, the dryer was left to run in a blank mode for 20 minutes (without samples) in order to compare the drying conditions. For each drying batch, 450 g of samples were weighed and equally distributed onto three different perforated trays in triplicate (150 g of sample fold three). The moisture loss was continuously recorded at 15 minutes intervals until the weight steadied. Afterward, the samples were removed from the dryer and left to cool at room temperature before being packed in low-density polyethylene bags and sealed.
Table 1. Actual and coded values of variables
The drying temperature (A) and air velocity (B) were considered as the independent variables. The DPPH radical scavenging activity, Ferric Reducing Antioxidant Power (FRAP), total phenolic and anthocyanin contents, and drying time were considered responses (Y). A total of 13 experiment points, including five replicates at the central point, were investigated, and the results are shown in . Before the analyses, the samples were powdered and sieved. The experiment was fitted to the following quadratic polynomial model:
Table 2. Coded central composite design with the responses
where Ai and Aj are the coded independent variables, βij, βii, and βi interactive, quadratic, and linear coefficients, respectively, β is the model intercept, and Y is the predicted response.
Total color change
The total color change value (ΔE) after the drying process of R. pimpinellifolia fruit was identified using the following expression:
where ΔL*, Δα* and Δb* are the variation of L*, α* and b* before and after drying, respectively.
Modeling of drying curves
The moisture ratio (MR) of R. pimpinellifolia was determined with Equationequation (4)(4)
(4) :
where M0 and Mt are initial moisture and moisture at time t, respectively. The drying data for R. pimpinellifolia was applied to thirteen drying models ().
Table 3. Models used to describe the drying curves of R. pimpinellifolia.
The statistical parameters, including root, mean square error (RMSE), chi-square (X2), and coefficient of determination (R2), were obtained using MATLAB software (R2016d) aimed at monitoring whether or not the models fit with the drying data for R. pimpinellifolia. Consequently, the highest R2 and the lowest X2 and RMSE were considered to be the best models. These parameters were expressed as Equationequations 5(5)
(5) –Equation7
(7)
(7) .
where represents the experimental mean moisture ratio, and MRp,i and MRe, i, reflect the predicted and ith experimental moisture ratios, respectively. n and N represent the constants and observations in the models, respectively.
Sample extraction
The dried fruit was ground and sieved with uniform particle size. The extraction was performed by weighing the sample (5 g) before mixing it with 20 mL of 80% methanol and allowing it to stand overnight at room temperature. After that, the mixtures were filtered and adequately diluted for total anthocyanins, total phenolic content, and antioxidant activity analyses.
Total phenolic content (TPC) measurement
The TPC was determined using a changed Folin-Ciocalteu method.[Citation16] A 20 μl of the 80% methanolic extract mixed with 100 μl of 10% (v/v) Folin-Ciocalteu reagent and 1.5 mL distilled water. The mixture was homogenized and stored in the dark for 5 min, followed by the addition of 300 μl of 7.5% (w/v) Na2CO3. The resultant mixture was stored in the dark for 2 hours, and the absorbance was noted at 760 nm using a Thermo Spectronic UV- spectrometer. The standard curve was obtained using gallic acid, and The TPC was expressed in gallic acid equivalent (mg GAE/100 g dw).
DPPH radical scavenging activity
The DPPH assay was performed following the procedure of Odabaş & Koca[Citation17] with some modifications. One mL of DPPH solution (prepared in 80% methanol) was mixed with 50 µL of the diluted extract and placed in the dark for 1 hour. The absorbances were noted at 517 nm by using the DPPH solution as a blank. The DPPH’s reduction ratio was determined with the following Equationequation (10)(10)
(10) .
where As is the absorbance of extract and Ac is the absorbance of the control. The values of DPPH radical scavenging calculated using a calibration curve of Trolox, and the results expressed in mmol Trolox equivalent (TE)/g dw.
Ferric Reducing Antioxidant Power assay (FRAP)
FRAP assay performed according to the procedure indicated in Pashazadeh et al.[Citation9] FRAP was calculated from a calibration curve using FeSO4 as standard. The results were given as mmol FeSO4 equivalents per g (mmol ISE/g).
Total anthocyanin
The determination of the total anthocyanins (TACN) of R. pimpinellifolia extracts was performed using the modified pH differential method reported by Zannou et al.[Citation18] Briefly, .05 mL of the methanolic extract was diluted with 1.95 mL of a buffer (made of 1.86 g of KCl, 6.3 mL HCl and 980 mL of distilled water) with a pH value equal to 1.0. An aliquot of .05 ml of another extract was diluted with 1.95 mL of a buffer with a pH value of 4.5 (made of 54.43 g of sodium acetate, 20 mL HCl, and 960 mL distilled water). The absorbances of the two solutions read at 510 and 700 nm. The TACN was calculated following Equationequation 8(8)
(8) and expressed as mg Cyanidin- 3- glucoside (c3g) equivalent /100 g.
where At = (Absorbance518 nm – Absorbance700 nm) pH 1.0 – (Absorbance518 nm – Absorbance700 nm) pH 4.5; MW (molecular weight) = 449.2 g.mol−1 for D3S, Df = dilution factor established in D, l = pathlength in cm and Ԑ = 26900 molar extinction coefficient, in L x mol−1 x cm−1, for c3g.
Rehydration curves and modeling
The rehydration test of the optimum drying parameters of R. pimpinellifolia was carried out at three different temperatures (20, 40, and 60°C). Next, 5 g of randomly selected dried samples were mixed with 500 mL distilled water and preheated at the defined temperatures. The fruit was removed from the liquid once every 1 h, gently blotted, and then weighed. The rehydration experiment was performed for 12 h., and all measurements were performed in triplicate. The moisture content during the hydration process was calculated on a dry basis using the expression shown in Ghellam and Koca[Citation19] and Pramiu et al.[Citation20]
where Mc is the moisture content at any time, Wst the weight of the sample at any time and Wdm is the dry weight for their treatment. The rehydration ratio (Rr) of dried R. pimpinellifolia fruit was determined following Benseddik et al.[Citation21]
where Mt, M0, and Me are the water contents at time t, before rehydration and at the end of rehydration, respectively. The kinetics modeling of rehydrated R. pimpinellifolia fruit was conducted by comparing 6 existing models: Exponential, Weibull, First-order kinetic, Exponential related, Peleg, and Vegas-gálves (). In the present study, the data of rehydration kinetics of dried R. pimpinellifolia fruit were statistically analyzed based on the values of chi-square (X2), root means square error (RMSE), and coefficient of determination (R2), using MATLAB software (R2016d). Accordingly, the models with higher R2 and the lowest X2 and RMSE were selected as the optimum models to interpret the hydration abilities of dried R. pimpinellifolia fruit.
Table 4. Models used to describe the rehydration kinetics of R. pimpinellifolia.
where Rrp,i and Rre,i, represent the ith predicted and experimental rehydration ratios, respectively. is the mean of the experimental rehydration ratio. N and n represent the number of observations and the number of constants in the models, respectively.
Instrumental texture analysis
The textural characteristics of R. pimpinellifolia were performed at room temperature using a texture analyzer (TA-TX plus, Stable Micro System, UK) following the modified method of Zielinska et al.[Citation24] The texture profile included hardness, springiness, cohesiveness, gumminess, chewiness, and resilience and was measured from the force-displacement graph of two compression-decompression cycles. The texture analyzer was placed on a 75 mm-diameter flat aluminum plunger (SMS P/75) before employing it in a compression-depression test cycle. The used parameters were: 1 s time lag between two compressions, strain to be 10% of sample height, pretest speed (.33 mm s−1), test speed (.33 mm s−1) posttest speed (.33 mm s−1). The texture analyzer software macro automatically computed the texture attributes. The results were averaged over 5 measurements.
Microstructure analysis
The microstructure analysis was performed using a scanning electron microscope (SEM, JEOL JSM-7001 F). Briefly, a small specimen was taken from the samples and attached to a stainless stub with double sticky tape. Then, the assembly was immediately sputtered with a gold/palladium target (60/40) in approximately 10 nm using a sputter coater, functioning with argon and plasma current for 2 min. The images were recorded at an acceleration voltage of 10 kV and 15 kV.
Shrinkage
The shrinkage of fresh and dried fruit was evaluated by taking pictures of the fruit in the projected areas using a smartphone. The images containing the targeted areas were loaded on the AutoCAD software, and the volumes were automatically calculated. The shrinkage of the samples’ volume change was expressed as the sample volume ratio at the end of the drying process (V) to the initial volume of fresh fruit (V0) following Equationequation 15(15)
(15) .
LC/MS/MS analysis
The phenolic compounds were determined using liquid chromatography coupled to a mass spectrometer detector (LC-MS/MS, Shimadzu LC-MS 8040) via electrospray ionization (ESI) and two pumps (LC-30 AD), a column oven (CTO-10AS VP), an autosampler (SIL- 30AC) and a degassing unit (DGU-20A 3 R). The MS system functioned with an ESI ionizing, nebulizing gas flow (3 L/min), 250°C of DL temperature, 400°C of heat block temperature, and 15 L/min of Drying Gas Flow: 15 L/min. A .45 µm nylon filter was used to filter the samples and standards before injecting 15 µL into a C18 reversed-phase column (Inertsil ODS-4.3 µm, 4.6 × 50 mm, GL Sciences Kat No: 5020–04042). The column temperature was set at 30°C. The mobile phase was constituted of water: formic acid in 99.9:.1 v:v (Mobile Phase A), methanol: formic acid in 99.9:.1 v:v (Mobile Phase B), and water: methanol in 50:50 v:v (Mobile Phase C). The flow rate of the solvents was .4 mL/min, and the following gradient solution was used: 0 min 14% B; 34 min, 37% B; 36 min, 37 B; 51 min, 38% B; 53 min, 38% B; 67 min, 44%B; 69 min, 44% B; 69–90 min, 14% B; 95 min, 14% B. The phenolic compounds were identified based on their elution time and quantified from their peak area. The identified compounds were quantified using a mixture of external standards prepared by dissolving methanol standards at concentrations of 0, 50, 75, 100, 150, and 200 ppm. The standards encompass (-)-epicatechin, quercetin-3-glucoside, protocatechuic acid, chlorogenic acid, tannic acid, p-coumaric acid, rutin, naringenin, gallic acid, catechin, fumaric acid, and quercetin.
Data analysis
Modeling analysis of rehydration and drying kinetics was performed using MATLAB software (R2016d). The model of the RSM, the 3D graphics, and the optimization plan were generated using the Trial Version of the Design-Expert software 9.0 (Stat-Ease Inc., Minneapolis, USA). ANOVA was used to evaluate the statistical significance of independent variables and the correlation between them. The adequacy of the optimization model was determined based on the coefficient of variation (CV), adjusted coefficient of determination (adj. R2), coefficient of determination (R2), and Fisher’s test value (F-value). The regression coefficients were considered significant at p < .05. The optimum conditions for total anthocyanins and phenolic contents and DPPH radical scavenging activity were obtained following the desirability function.
Results and discussion
Characteristics of fresh R. pimpinellifolia fruit
The physicochemical properties, along with total phenolic content, total anthocyanins, and DPPH radical scavenging of the fresh fruit of R. pimpinellifolia, are presented in . Accordingly, the color properties of fresh R. pimpinellifolia were investigated in the present study and detected to be a mixture color of brightness (L*:30.35), red (a*:2.53), and yellow (b*:2.20). The fruit flesh to seed ratio per fruit was 3.09, and dry matter was 39.06%. In contrast, the total phenolic content, total anthocyanins, DPPH radical scavenging and FRAP values of the fresh R. pimpinellifolia fruit were 1331.10 mg GAE/100 g, 675.05 mg c3g E/100 g, 170.86 mmol TE/g and 896.81 ± 50.12, respectively. Pashazadeh et al.[Citation9] have reported similar TPC and TACN of 13938.57 mg GAE/kg; 9652.79 mg c3g E/kg in Turkish fresh R. pimpinellifolia, respectively, but they found the higher antioxidant activity of 311.97 mmol TE/g, which could be probably due to the harvest season. Similar values of total phenolic content and antioxidant values have been reported in R. pimpinellifolia from different locations.[Citation3]
Table 5. Physical and chemical characteristics of fresh R. pimpinellifolia fruits
Optimization with RSM
Effect temperature and air velocity on drying time
The responses and coded values of the independents variables of each experiment are represented in . As can be seen, the effects of temperature and air velocity were shown to influence the investigated responses. The drying time and experimental points (temperature and air velocity) are displayed in . A total of 13 runs of drying was carried out, and the drying time for these runs ranged from 195 min to 1020 min. The lowest drying time was registered at 84.14°C and 1.25 m.s−1 air velocity, while the maximum drying time was found at 55.86°C and 1.25 m.s−1. The maximum and minimum drying times were obtained at the same air velocity (1.25 m.s−1) but two different temperatures (55.86 and 84.14°C). A similar phenomenon occurred with other drying techniques, as an increase in the drying temperature accelerated the operation by reducing the time independently of the air velocity.[Citation13,Citation25,Citation26]
Effect of drying parameters on color
The color of the fresh R. pimpinellifolia fruit investigated in the present study is the result of a mixture of brightness (L*:30.35), red (a*:2.53), and yellow (b*:2.20) (). The drying process has been reported to induce a considerable color change in the final products.[Citation27] The total color change (ΔE) registered at each experimental point of the optimization process is presented in . ΔE expresses the variations in the fruit color which occurs during the drying process. A total of 13 runs of the drying process were carried out. The values of ΔE were ranged from 3.16 to 7.01, while the highest value was recorded at run 12 (60°C; .75 m.s−1; 690 min), respectively. The higher temperature combined with a higher air velocity and long drying time caused the color degradation in fresh R. pimpinellifolia fruit at 60–70°C, .54–1.25 m.s−1, and 420–1020 min. Similarly, Koca et al.[Citation27] showed that the highest color degradation occurred at the highest temperature and air velocity of 60°C and 1.75 m.s−1.
Analysis of the optimization model
The coded values and responses of the independent variables for each experimental run are shown in . Among 13 drying test runs, the highest total phenolic compound was detected at run 12 (60°C and .75 m.s−1), while the lowest value was obtained at run 2 (70°C and 1.96 m s−1). The maximum value of total anthocyanin was found at run 2 (70°C and 1.96 m.s−1) and the minimum at run 1 (84.14°C and 1.25 m s−1). The most significant and lowest DPPH values were observed at run 13 (70°C and 1.25 m.s−1) and run 1 (84.14°C and 1.25 m s−1), respectively. The highest FRAP was found at run 1 (84.14°C and 1.25 m s−1) while the lowest value was found at run 9 (55.86 and 1.25 m s−1).
Quadratic polynomial models were developed with regression coefficients identified as statistically significant at p < .05. The simplified second-order models of fundamental factors of the total phenolic content, total anthocyanins, and DPPH values of R. pimpinellifolia found by considering independent variables such as drying temperature (A) and air velocity (B) were as follows:
where A and B are the coded values of drying temperature and air velocity. The ANOVA of the total phenolic content revealed that the model had good R2 (.91), adjusted R2 (.85), CV (8.77%), and adequate precision (13.77) which were significant at p < .0013. The R2 and adjusted R2 were close and higher than .80 which means that the experimental and predicted values were in higher conformity. The CV (8.77%) indicated a better replication of the model as the lower CV is desired and represents the standard deviation as a percentage, although an adequate precision higher than 4 is preferred.
The model generated for the total phenol compound had an acceptable accuracy of 13.77, which means that the model can navigate the experimentation space. The lack of fit was non-significant, which indicates that the model fitted well. The model produced for the total anthocyanins was significant with desirable R2 (.89), adjusted R2 (.81), CV (9.62%), and adequate precision (11.60) at p < .00. The anthocyanins results showed a non-significant lack of fit, implying that the model was a good fit. Concerning the model developed for DPPH radical scavenging of R. pimpinellifolia, the model was significant with reasonable R2 (.84), adjusted R2 (.72), CV (4.15%) and adequate precision (7.64) which were significant at p < .01. The lack of fit of the model of DPPH was non-significant and had a good fit. The RSM, together with models developed for the total phenolic content, total anthocyanins, and DPPH radical scavenging of R. pimpinellifolia extracts, was considered to be successful. The model developed for FRAP had reasonable R2 (.85), adjusted R2 (.74), CV (6.43%) and adequate precision (9.73) which were significant at p < .01. The lack of fit of the model of FRAP was non-significant and had a good fit.
Effect temperature and air velocity on responses
The effects of the linear and quadratic terms on total phenolic content, total anthocyanins, and DPPH values obtained from R. pimpinellifolia extracts are shown in . The linear term of temperature did not affect the total phenol content with F value .13 (p ˂ .73), but the quadratic term of temperature significantly impacted the response. Meanwhile, the linear and quadratic terms of air velocity showed the most significant effect on the total phenolic content with F values of 29.42 (p ˂ .00) and 17.05 (p ˂ .00), respectively. Regarding the total anthocyanins, the linear term of air velocity was the most significant with an F value of 13.07 (p ˂ .01). The quadratic term of temperature was significant at F value 33.55 (p ˂ .00), but the quadratic term of air velocity did not affect the total anthocyanins at F value .25 (p ˂ .63). For the model developed for DPPH radical scavenging of R. pimpinellifolia, only the quadratic term of temperature had a significant effect with F value 32.11 at p ˂ .00. Whereas, the linear terms of terms of temperature (p ˂ .00) and air velocity (p ˂ .02) showed significant effects for the model developed for FRAP. The three-dimensional graphics generated through the RSM are shown in . The analysis of RSM revealed that the total phenolic content increased with the increase in temperature and decreased with increased air velocity. The highest total phenolic content of R. pimpinellifolia was obtained at .75 m.s−1 and 65 and 70°C (). Beyond this temperature, a loss of phenolic compounds occurred, resulting in a decrease in the total phenolic content.
Table 6. Results of statistical analysis of the thirteen selected drying models applied to experimental drying data of R. pimpinellifolia fruits
The increase in drying air velocity and temperature induced an expansion of the total anthocyanin and reached the maximum peak at the temperatrures between 60°C and75°C. This result might be related to prolonged contact of the anthocyanins with oxygen and activity of the oxidative enzymes during the long drying period.[Citation28] At temperatures beyond 75°C, the total anthocyanin value decreased (), indicating a loss at this experimental point. Enzymatic or non-enzymatic reactions might cause this loss of anthocyanin during drying. The antioxidant activity increased significantly with the rise of both drying temperature and air velocity (). The DPPH radical scavenging and FRAPof R. pimpinellifolia reached the maximum values at the air velocity of 1.75 m s−1 and temperature of 80°C. The higher air velocity and temperature were applied, the higher the antioxidant capacity generated. These findings indicated that the applied drying conditions had significant effects on the antioxidant properties of R. pimpinellifolia fruit.
Multi-RSM
The air velocity and temperature significantly affected the total phenolic compounds, total anthocyanins, and antioxidant activity of R. pimpinellifolia fruit. Considering data in , the optimum drying conditions were figured at run 7 and consisted of 1.75 m.s−1 and 60°C. Under these optimum experimental conditions, the total phenolic compounds, total anthocyanins, DPPH radical scavenging and FRAP were 1312 mg GAE/100 g, 444 mg c3g E/100 g, 119 mmol TE/g and 585 mmol ISE/g, respectively. The desirability function allowed to determine the theoretical optimum drying conditions as 1.75 m.s−1 and 67.21°C giving the predicted optimum total phenolic compounds, total anthocyanins, DPPH radical scavenging and FRAP values of 1327 ± 5 mg GAE/100 g, 490 ± 2 mg c3g E/100 g, 110 ± 2 mmol TE/g and 698 ± 15.21 mmol ISE/g, respectively. Further analyses proceeded for confirmation, and the results found to be 915 ± 3 mg GAE/100 g, 418 ± 3 mg c3d E/100 g, 146 ± 1 mmol TE/g and 717 ± 12 mmol ISE/g for the total phenolic compounds, total anthocyanins and DPPH radical scavenging and FRAP values, respectively. The perturbation plot showed that the variable temperature strongly affected the total anthocyanins as indicated by a steep slope ().
Impacts of evaluating the optimization process
The efficiency of the optimization process was evaluated considering the values of the responses of the experimental optimum point and fresh fruit. TPC, TACN and DPPH radical scavenging slightly decreased from 1331 to 1328 mg GAE/100 g (.002%), 675 to 491 mg c3d E/100 g (.27%) and 171 to 110 (.36%). These losses were not significant and are supported by the findings of Pashazadeh et al.[Citation9] who reported slight losses of TPC, TACN and antioxidant activities in R. pimpinellifolia after convective drying at 50°C, 70°C, and 90°C. Koca et al.[Citation25] recorded the highest loss of total phenolic content at 70°C. Chang & Liu[Citation29] also mentioned the decrease of phenolic compounds in tomatoes dried at 40, 80, and 120°C for 140 min. Therefore, it can be assumed that the application of the convective drying at 67. 21°C; 1.75 m.s−1 better preserved the TPC, TACN, and antioxidant activity of R. pimpinellifolia.
Drying kinetic and modeling of optimum point
The dehydration kinetics of drying parameters (67.21°C; 1.75 m.s−1) giving the optimum total phenolic content, total anthocyanin, and antioxidant activity are presented in . The moisture continuously decreased as the drying period increased, reaching a constant moisture rate at 330 min which was similar to the agricultural drifted products. This result would explaine the diffusion in the primary physical mechanism directing the movement of moisture.[Citation7,Citation13] The shape of the drying curve for R. pimpinellifolia fruit indicated that the suppression of moisture from the fruit was faster during the early drying periods and then decreased as the drying time increased (.). This could be explained by the fact that a higher drying temperature might induce a greater drying rate.[Citation13]
Figure 5. Moisture content versus time for drying curve of R. pimpinellifolia fruits at 67. 21°C; 1.75 m.s−1
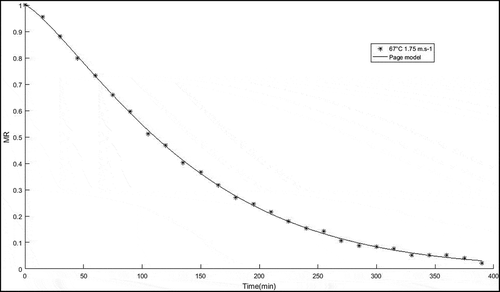
Thirteen models developed for the drying of agricultural products were tested for their correlation with the drying data for R. pimpinellifolia fruit (). The results from analyzing these models for the connective drying of R. pimpinellifolia fruit are noted in . The best model describing R. pimpinellifolia fruit was selected based on the values of constants, coefficient of determination (R2), root mean square error (RMSE), and chi-square (X2). All the tested models were acceptable with at least R2 = .98.[Citation13] However, the best-fit model was the Page model with the highest R2 and the lowest RMSE and X2. The R2, RMSE, and X2 values of the Page model were .99, .01, and .00, respectively (). Accordingly, the Page model was chosen as the best model per the model’s results in predicting the drying performance of R. pimpinellifolia fruit. Similarly, the Page model has been reported to explain the drying behavior of many food products such as tomatoes.[Citation30]
Table 7. Textural and shrinkage characteristics
Effects of drying on fruit microstructure
Scanning electron microscopy (SEM) analysis was undertaken in order to view the effect of dehydration on the dried fruit tissues (). The new fruit tissues showed small and clear, irregular but well-organized cell walls. The drying treatments caused essential changes that were related to water migration cells and turgor.[Citation31] The cells of the dried fruit presented huge pores with a deformed, irregular, and wrinkled structure. Similarly, the hot air-drying of mushrooms had caused severe shrinkage and collapse of the tissues[Citation32] as the microstructure of dried fruit is associated with transient thermal, water loss, and chemical changes.
Textural characteristics of dried fruit
Changes in moisture content occurring during the drying process lead to mechanical or textural transformation. The texture attributes of both fresh and dried R. pimpinellifolia obtained at the optimum point are given in . The hardness, springiness, cohesiveness, gumminess, chewiness, and resilience of new dried R. pimpinellifolia were determined in the averages of 7.63 and 47.85 N, .91 and .78, .30 and .65, 6.40 and 31.03, 5.85 and 24.31, and .43 and .26, respectively. The data presented in show that hot air-drying significantly increased the hardness, cohesiveness, gumminess, and chewiness. However, springiness and resilience were relatively low compared to the fresh fruit. In the hot air convective drying system, water was evaporated first from the surface and then from the interior of R. pimpinellifolia. This process produces significant shrinkage and collapsed surfaces leading to rigid textural characteristics.[Citation24] Thus, the increase in the hardness, cohesiveness, gumminess, and chewiness in R. pimpinellifolia might probably be due to the moisture removal and the concentration of other components, as was observed for the hardness and chewiness in dried bananas and mushrooms.[Citation33] Likewise, Chong et al.[Citation34] associated the significant increase in hardness during sun-drying with the depolymerization of cell wall components such as pectin. The springiness was reduced in the dried R. pimpinellifolia, suggesting a decrease in elasticity during the drying process since the springiness depends on the gelling agent in the fruit. Kotwaliwale et al.[Citation33] and Chong et al.[Citation34] reported a decrease in springiness and cohesiveness in dried mushrooms and chempedak, respectively. The reduction of springiness and resilience during hot air drying was associated with water removal, particularly at higher temperatures.[Citation34]
Shrinkage
The shrinkage of R. pimpinellifolia dried under the optimum conditions (67.21°C; 1.75 m.s−1) was 56.32% (). Koç et al.[Citation35] reported ranges of shrinkage of 25.80% and 62.40% and determined that the shrinkage is not the only function of moisture content but also depends on the drying method. Similarly, Maskan[Citation36] found that the shrinkage was 85%, 81%, and 76% in kiwifruit samples for microwave, hot air, and hot air-microwave drying, respectively. The author noticed that the hot air drying promoted a moderate shrinkage, which was revealed to be related to the long drying time which allowed more time for the product to shrink.[Citation37] The findings of the present study are consistent with those obtained in previous studies.
Rehydration characteristics and modeling
The rehydration capacity of the dried R. pimpinellifolia fruit with higher total phenolic content, total anthocyanins, and antioxidant activity was determined at three different temperatures of 20°C (), 40°C (), and 60°C (). The rehydration capacity of the dried R. pimpinellifolia fruit results is presented in . The rehydration analysis showed a rapid water absorption that decreased as the rehydration progressed. This phenomenon usually occurs in rehydration systems of agricultural products and has been reported to be related to the mass transfer decrease and equilibrium closeness.[Citation38] The rehydration was faster at 60°C, followed by 40°C and 20°C. Consequently, these findings support the results issued from RSM for the drying of R. pimpinellifolia fruit. With regard to rehydration capacity, 60°C could be considered as the optimum temperature for the drying of R. pimpinellifolia fruit. A similar optimum rehydration capacity temperature has been previously reported for tomatoes.[Citation30]
The rehydration kinetics were statistically analyzed with Peleg, First-order kinetic, Weibull, Exponential, Exponential related, and Vegas-gálves models using MATLAB software. The results generated by the statistical analyses of these models are recorded in . These models were appropriate for depicting the rehydration kinetics of dried R. pimpinellifolia fruit since they displayed R2 = .95–.99, RMSE = .02–.08, and X2 = .00–.03. However, these rehydration models behaved differently with regard to the rehydration temperature. The accuracy of the rehydration models is dependent on rehydration temperature and capacity.[Citation39] In the present study, Peleg and Vegas-gálves models were the best-fit models for predicting the rehydration kinetics of R. pimpinellifolia fruit at 20, 40, and 60°C. The R2, RMSE and X2 values ranged from .98 to .99, from .02 to .04 and from .00 to .03 for the Vegas-gálves model and .9933, from .03 to .05 and from .00 to .00 for the Peleg model, respectively. These values indicated that the Vegas-gálves and Peleg equation was adequate for describing the rehydration behavior of R. pimpinellifolia at 20, 40, and 60°C. Nonetheless, these models fitted better with the rehydration performed at 60°C. Moreover, the Weibull and Exponential models fitted well at 60°C, displaying high R2 and lower RMSE and X2 (). Our results were in agreement with those from previous research in which the Peleg model was shown to be ideal for the rehydration of many food products.[Citation21–23]
Table 8. Results of statistical analysis of the modeling of rehydration data
Table 9. LC/MS/MS quantification of phenolic compounds identified in fresh and dried fruit (ppm)
Effects of drying on phenolic composition
Analysis of the data on phenolic compounds showed that the application of hot air drying at 67.21°C/1 m.s−1 had positive effects on the retention of R. pimpinellifolia phenolic compounds () which were found to be in higher concentrations in dried fruit. This finding agrees with those from previous studies in which the application of thermal and non-thermal drying might promote the retention of bioactive compounds and contribute to the release of compounds primarily present in bound form and linked to the cell walls.[Citation40]
In , twelve major phenolic compounds were identified from R. pimpinellifolia, giving a total of 752.51 mg/kg and 1987.10 mg/kg in fresh and dried samples, respectively. The identified compounds included catechin, epicatechin, quercetin-3-glucoside, protocatechuic acid, fumaric acid, gallic acid, chlorogenic acid, tannic acid, p-coumaric acid, rutin, naringenin, and quercetin. An overall increase in R. pimpinellifolia phenolic compounds was observed after the application of hot air drying at 67.21°C/1.75 m.s−1 for 375 min. Dewanto et al.[Citation41] reported the same observations in sweet corn dried at 115°C for 10, 25, and 50 min. Moreover, Esparza-Martínez et al.[Citation42] and Valadez-Carmona et al.[Citation43] mentioned the increases in phenolic compounds of mandarin waste and cacao at the end of drying processes performed at 120°C and 60°C, respectively. In , the concentration of rutin slightly decreased during the drying process, suggesting that rutin may be converted into other compounds or maybe destroyed either upon the application of actual drying parameters or under phenolic oxidase actions. Similar observations have been done by Asami et al.[Citation40] and Sun et al.,[Citation44] who ascertained that hot air drying might be harmful to phenolic compounds due to oxidation and the release of free phenolic compounds.[Citation44] Finally, catechin was the most abundant phenolic compound detected in both fresh (609.35 mg/kg) and dried (1581.58 mg/kg) fruit. Epicatechin, quercetin-3-glucoside, protocatechuic acid, chlorogenic acid, quercetin, rutin, fumaric acid, and gallic acid were other significant compounds identified in dried products (). These major phenolic compounds are biologically active and have many beneficial effects on human health. Catechin and its derivatives produce physiological results such as antioxidative, anticancer, anti-obesity, and hypolipidaemic effects.[Citation45]
Conclusion
R. pimpinellifolia fruit was dried with a convective drying system in order to determine the effects of drying parameters such as temperature and air velocity on total phenolic compounds, total anthocyanins, and antioxidant activity values. The optimization operation using RSM showed that the optimum drying temperature was 67.21°C and the air velocity was 1.75 m.s−1. Under this optimum condition, the maximum total phenolic compounds, total anthocyanins, DPPH radical scavenging and FRAPvalues were 1328 ± 5 mg GAE/100 g, 491 ± 2 mg c3g E/100 g, 110 ± 2 mmol TE/g and 698 ± 15 mmol ISE/g, respectively. This optimum drying condition was subjected to mathematical modeling using thirteen existing drying models. All the models fitted well. However, the Page model was revealed to be the most adequate drying model for describing and estimating the mass transfer of coefficients. The rehydration capacity of dried R. pimpinellifolia fruit was tested at 20, 40, and 60°C. The rehydration findings indicated that water absorption of the dried fruit was faster at 60°C, followed by 40°C and 20°C. Six rehydration models including Peleg, First-order kinetic, Weibull, Exponential, Exponential related, and Vegas-gálves models, were tested to the experimental data of rehydration. The Vegas-gálves and Peleg models were the most successful in predicting the rehydration behavior of dried R. pimpinellifolia fruit at 20, 40, and 60°C. Moreover, Weibull and exponential models were found to be well- fitted at 60°C. The application of convective drying increased hardness, cohesiveness, gumminess, and chewiness while decreasing the springiness and resilience. Catechin, epicatechin, quercetin-3-glucoside, protocatechuic acid, chlorogenic acid, quercetin, rutin, fumaric acid, and gallic acid were the primary phenolic compounds identified. The dried fruit of R. pimpinellifolia has thus been shown to have promising potential as a healthy and nutritional product for the food and pharmaceutical industries.
Acknowledgments
The participation of authors Turki M.S. Aldawoud and Charis M. Galanakis in this work was supported by the Researchers Supporting Project number (RSP-2021/197) of King Saud University, Riyadh, Saudi Arabia. Author (S.A.I.) would also like to acknowledge the support of the Agricultural Research Station at North Carolina Agricultural and Technical State University (Greensboro, NC 27411, USA). This research was funded, in part, by Grants (project Number NC.X337-5-21-170-1 and NC.X341-5-21-170-1) from the National Institute of Food and Agriculture (NIFA). Its contents are solely the responsibility of the authors and do not necessarily represent the official views of NIFA.
Additional information
Funding
References
- Galanakis, C. M. The Food Systems in the Era of the Coronavirus (COVID-19) Pandemic Crisis. Foods. 2020, 9(4), 523. DOI: https://doi.org/10.3390/foods9040523.
- Galanakis, C. M.; Aldawoud, T. M. S.; Rizou, M.; Rowan, N.; Ibrahim, S. Food Ingredients and Active Compounds against the Coronavirus Disease (COVID-19) Pandemic: A Comprehensive Review. Foods. 2020, 9(11), 1701. DOI: https://doi.org/10.3390/foods9111701.
- Murathan, Z. T.; Zarifikhosroshahi, M.; Kafkas, E. Characterizatıon of Bioactive Compounds in Rosehip Species. Ital. J. Food Sci. 2016, 28, 314–325.
- Galanakis, C. M. Separation of Functional Macromolecules and Micromolecules: From Ultrafiltration to the Border of Nanofiltration. Trends Food Sci. Technol. 2015, 42(1), 44–63. DOI: https://doi.org/10.1016/j.tifs.2014.11.005.
- Galanakis, C. M.; Tsatalas, P.; Galanakis, I. M. Implementation of Phenols Recovered from Olive Mill Wastewater as UV Booster in Cosmetics. Ind. Crops Prod. 2018, 111, 30–37. DOI: https://doi.org/10.1016/j.indcrop.2017.09.058.
- Kovacevic, D. B.; Barba, F. J.; Granato, D.; Galanakis, C. M.; Herceg, Z.; Dragovic-Uzelac, V.; Prutnik, P. Pressurized Hot Water Extraction (PHWE) for the Green Recovery of Bioactive Compounds from Steviol Glycosides from Stevis Rebaudiana Bertoni Leaves. Food Chem. 2018, 254, 150–157. DOI: https://doi.org/10.1016/j.foodchem.2018.01.192.
- Doymaz, I. Convective Drying Kinetics of Strawberry. Chem. Eng. Process. Process Intensif. 2008, 47(5), 914–919. DOI: https://doi.org/10.1016/j.cep.2007.02.003.
- Erbay, Z.; Icier, F. Optimization of Hot Air Drying of Olive Leaves Using Response Surface Methodology. J. Food Eng. 2009, 91(4), 533–541. DOI: https://doi.org/10.1016/j.jfoodeng.2008.10.004.
- Pashazadeh, H.; Zannou, O.; Koca, I. Modeling of Drying and Rehydration Kinetics of Rosa Pimpinellifolia Fruits: Towards Formulation and Optimization of a New Tea with High Antioxidant Properties. J. Food Process. Eng. 2020, 43(10), 13486. DOI: https://doi.org/10.1111/jfpe.13486.
- Tunde-Akintunde, T. Y. Mathematical Modeling of Sun and Solar Drying of Chilli Pepper. Renew. Energy. 2011, 36(8), 2139–2145. DOI: https://doi.org/10.1016/j.renene.2011.01.017.
- Nurafifah, F.; Chuah, A. L.; Wahida, M. A. P. F. Drying of Plectranthus Amboinicus (Lour) Spreng Leaves by Using Oven Dryer. Eng. Agric. Environ. Food. 2018, 11(4), 239–244. DOI: https://doi.org/10.1016/j.eaef.2018.08.002.
- Chielle, D. P.; Bertuol, D. A.; Meili, L.; Tanabe, E. H.; Dotto, G. L. Convective Drying of Papaya Seeds (Carica Papaya L.) And Optimization of Oil Extraction. Ind. Crops Prod. 2016, 85, 221–228. DOI: https://doi.org/10.1016/j.indcrop.2016.03.010.
- do Nascimento Silveira Dorneles, L.; Luís, A.; Goneli, D.; Andrea, C.; Cardoso, L.; Bezerra, C.; Rosemari, M.; Cardoso, G.; Schoeninger, V. Effect of Air Temperature and Velocity on Drying Kinetics and Essential Oil Composition of Piper Umbellatum L. Leaves. Ind. Crop. Prod. 2019, 142, 111846. DOI: https://doi.org/10.1016/j.indcrop.2019.111846.
- Hosseinzadeh Samani, B.; Gudarzi, H.; Rostami, S.; Lorigooini, Z.; Esmaeili, Z.; Jamshidi-kia, F. Development and Optimization of the New Ultrasonic-infrared-vacuum Dryer in Drying Kelussia Odoratissima and Its Comparison with Conventional Methods. Ind. Crops Prod. 2018, 123, 46–54. DOI: https://doi.org/10.1016/j.indcrop.2018.06.053.
- Kumar, S.; Mahanand, S. S.; Kumar, P. Mathematical Modelling and Experimental Analysis of Broccoli (Brassica Oleracea I.) In Tray Dryer. J. Pharmacog. Phytochem. 2017, 6(6), 1049–1052.
- Zannou, O.; Koca, I. Optimization and Stabilization of the Antioxidant Properties from Alkanet (Alkanna Tinctoria) with Natural Deep Eutectic Solvents. Arab. J. Chem. 2020, 13(8), 6437–6450. DOI: https://doi.org/10.1016/j.arabjc.2020.06.002.
- Odabaş, I. H.; Koca, I. Application of Response Surface Methodology for Optimizing the Recovery of Phenolic Compounds from Hazelnut Skin Using Different Extraction Methods. J. Ind. Crops Prod. 2016, 91, 114–124. DOI: https://doi.org/10.1016/j.indcrop.2016.05.033.
- Zannou, O.; Koca, I.; Aldawoud, T. M. S.; Galanakis, C. M. Recovery and Stabilization of Anthocyanins and Phenolic Antioxidants of Roselle (Hibiscus Sabdariffa L.) With Hydrophilic Deep Eutectic Solvents. Molecules. 2020, 25(16), 3715. DOI: https://doi.org/10.3390/molecules25163715.
- Ghellam, M.; Koca, I. Modelling of Rehydration Kinetics of Desert Truffles (Terfezia Spp.) Dried by Microwave Oven. Turk. J. Agric. Food Sci. Technol. 2020, 8(2), 407–415. DOI:https://doi.org/10.24925/turjaf.v8i2.407-415.3083.
- Pramiu, P. V.; Rizzi, R. L.; Do Prado, N. V.; Coelho, S. R. M.; Bassinello, P. Z. Numerical Modeling of Chickpea (Cicer Arietinum) Hydration: The Effects of Temperature and Low Pressure. J. Food Eng. 2015, 165, 112–123. DOI: https://doi.org/10.1016/j.jfoodeng.2015.05.020.
- Benseddik, A.; Azzi, A.; Zidoune, M. N.; Khanniche, R.; Besombes, C. Empirical and Diffusion Models of Rehydration Process of Differently Dried Pumpkin Slices. J. Saudi Soc. Agric. Sci. 2019, 18, 401–410. DOI: https://doi.org/10.1016/j.jssas.2018.01.003.
- Noshad, M.; Mohebbi, M.; Shahidi, F.; Mortazavi, S. A. L. I. Kinetic Modeling of Rehydration in Air-dried Quinces Pretreated Wıth Osmotic Dehydration and Ultrasonic. J. Food Process. Preserv. 2012, 36(5), 383–392. DOI: https://doi.org/10.1111/j.1745-4549.2011.00593.x.
- Vega-Gálvez, A.; Notte-Cuello, E.; Lemus-Mondaca, R.; Zura, L.; Miranda, M. Mathematical Modelling of Mass Transfer during Rehydration Process of Aloe Vera (Aloe Barbadensis Miller). Food Bioprod. Process. 2009, 87(4), 254–260. DOI: https://doi.org/10.1016/j.fbp.2008.10.004.
- Zielinska, M.; Sadowski, P.; Błaszczak, W. Combined Hot Air Convective Drying and Microwave-vacuum Drying of Blueberries (Vaccinium Corymbosum L.): Drying Kinetics and Quality Characteristics. Dry. Technol. 2015, 34(6), 665–684. DOI: https://doi.org/10.1080/07373937.2015.1070358.
- Koca, I.; Ustun, N. S.; Koyuncu, T. Effect of Drying Conditions on Antioxidant Properties of Rosehip Fruits (Rosa Canina Sp.). Asian J. Chem. 2009, 21(2), 1061–1068.
- Pashazadeh, H.; Zannou, O.; Koca, I. Modeling and Optimization of Drying Conditions of Dog Rose for Preparation of a Functional Tea. J. Food Process Eng. 2021, 44(3), e13632. DOI: https://doi.org/10.1111/jfpe.13632.
- Koca, I.; Yilmaz, V. A.; Odabas, I. H.; Tekguler, B. Optimization of Drying Parameters for Chestnut Fruits Using Central Composite Design. Acta Hortic. 2018, 1220(1220), 221–226. DOI: https://doi.org/10.17660/ActaHortic.2018.1220.31.
- Tontul, I.; Eroğul, E.; Topuz, A. Convective and Refractance Window Drying of Cornelian Cherry Pulp: Effect on Physicochemical Properties. J. Food Process. Eng. 2018, 41(8), e12917, 1–8. DOI: https://doi.org/10.1111/jfpe.12917.
- Chang, C.-H.; Liu, Y.-C. Study on Lycopene and Antioxidant Contents Variations in Tomatoes under Air-drying Process. J. Food Sci. 2007, 72(9), E532–E540. DOI: https://doi.org/10.1111/j.1750-3841.2007.00570.x.
- Doymaz, I. Air-drying Characteristics of Tomatoes. J. Food Eng. 2007, 78(4), 1291–1297. DOI: https://doi.org/10.1016/j.jfoodeng.2005.12.047.
- Zhang, L.; Qiao, Y.; Wang, C.; Liao, L.; Liu, L.; Shi, D.; An, K.; Hu, J.; Xu, Q. Effects of Freeze Vacuum Drying Combined with Hot Air Drying on the Sensory Quality, Active Components, Moisture Mobility, Odors, and Microstructure of Kiwifruit. J. Food Qual. 2010. DOI: https://doi.org/10.1155/2019/8709343.
- Giri, S. K.; Prasad, S. Optimization of Microwave-vacuum Drying of Button Mushrooms Using Response-surface Methodology. Dry. Technol. 2007, 25(5), 901–911. DOI: https://doi.org/10.1080/07373930701370407.
- Kotwaliwale, N.; Bakane, P.; Verma, A. Changes in Textural and Optical Properties of Oyster Mushroom during Hot Air Drying. J. Food Eng. 2007, 78(4), 1207–1211. DOI: https://doi.org/10.1016/j.jfoodeng.2005.12.033.
- Chong, C. H.; Law, C. L.; Cloke, M.; Abdullah, L. C.; Daud, W. R. W. Drying Kinetics, Texture, Color, and Determination of Effective Diffusivities during Sun Drying of Chempedak. Dry. Technol. 2008, 26(10), 1286. DOI: https://doi.org/10.1080/07373930802307308.
- Koç, B.; Eren, I.; Ertekin, F. K. Modelling Bulk Density, Porosity and Shrinkage of Quince during Drying: The Effect of Drying Method. J. Food Eng. 2008, 85(3), 340–349. DOI: https://doi.org/10.1016/j.jfoodeng.2007.07.030.
- Maskan, M. Drying, Shrinkage and Rehydration Characteristics of Kiwifruits during Hot Air and Microwave Drying. J. Food Eng. 2001, 48(2), 177–182. DOI: https://doi.org/10.1016/S0260-8774(00)00155-2.
- Ratti, C. Shrinkage during Drying of Foods. J. Food Eng. 1994, 23(1), 91–105. DOI: https://doi.org/10.1016/0260-8774(94)90125-2.
- García-Pascual, P.; Sanjua, N.; Sanjua, N.; Sanjua, N. Morchella esculenta (morel) rehydration process modelling. J. Food Eng. 2006, 72(4), 346–353. DOI: https://doi.org/10.1016/j.jfoodeng.2004.12.014.
- Zhao, Y. Y.; Yi, J. Y.; Bi, J. F.; Chen, Q. Q.; Zhou, M.; Zhang, B. Improving of Texture and Rehydration Properties by Ultrasound Pretreatment for Infrared-dried Shiitake Mushroom Slices. Dry. Technol. 2019, 37(3), 352–362. DOI: https://doi.org/10.1080/07373937.2018.1456449.
- Asami, D.; Hong, Y.; Barrett, D.; Mitchell, A. Comparison of the Total Phenolic and Ascorbic Acid Content of Freeze-dried and Air-dried Marionberry, Strawberry, and Corn Grown Using Conventional, Organic, and Sustainable Agricultural Practices. J. Agric. Food Chem. 2003, 51(5), 1237–1241. DOI: https://doi.org/10.1021/jf020635c.
- Dewanto, V.; Wu, X. Z.; Adom, K. K.; Liu, R. H. Thermal Processing Enhances the Nutritional Value of Tomatoes by Increasing Total Antioxidant Activity. J. Agric. Food Chem. 2002, 50(10), 3010–3014. DOI: https://doi.org/10.1021/jf0115589.
- Esparza-Martínez, F. J.; Miranda-López, R.; Mata-Sánchez, S. M.;Guzmán-Maldonado, S. H. Extractable and non-extractable phenolics and antioxidant capacity of mandarin waste dried at different temperatures. Plant Food. Hum. Nutr. 2016, 71(3),294-300.
- Valadez-Carmona, L.; Plazola-Jacinto, C. P.; Hernández-Ortega, M.; Navarro, M. D. H.; Villarreal, F.; Necoechea-Mondragón, H.; Ortiz-Moreno, A.; Ceballos-Reyes, G. Effects of Microwaves, Hot Air and Freeze-drying on the Phenolic Compounds, Antioxidant Capacity, Enzyme Activity and Microstructure of Cacao Pod Husks (Theobroma Cacao L.). Innov. Food Sci. Emerging Technol. 2017, 41, 378–386. DOI: https://doi.org/10.1016/j.ifset.2017.04.012.
- Sun, Y.; Shen, Y.; Liu, D.; Ye, X. Effects of Drying Methods on Phytochemical Compounds and Antioxidant Activity of Physiologically Dropped Un-matured Citrus Fruits. LWT - Food Sci. Technol. 2014, 60(2), 1269–1275. DOI: https://doi.org/10.1016/j.lwt.2014.09.001.
- Demir, F.; Uzun, F. G.; Durak, D.; Kalender, Y. Subacute Chorpyrifos-induced Oxidative Stress in Rats Erythrocytres and the Protective Effects of Catechin and Quercetin. Pest. Biochem. Physiol. 2011, 99(1), 77–81. DOI: https://doi.org/10.1016/j.pestbp.2010.11.002.