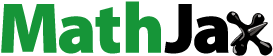
ABSTRACT
The objective of the study was to determine the geometric, gravimetric, aerodynamic, and frictional properties of maize grain as affected by varietal differences and moisture content. Honampa, Omankwa, Abontem, Timtim, and Obatanpa were the five maize grain varieties studied. Moisture content, geometric properties, gravimetric properties, aerodynamic properties, and frictional properties were studied. The geometric mean diameters ranged between 6.65 and 7.55 mm, while projected areas ranged between 52.98 and 73.12 m2. The surface area was between 139.69 and 180.29 mm2, and sphericity was from 0.71 to 0.80. The following ranges were obtained as thousand grain weight (213.74 and 292.89 g), and bulk density (709.50 and 746.00 kg/m3). The volume of a single seed was between 1.71 × 10−7 and 2.34 × 10−7 m3 while true density was 1250.0 kg/m3. Porosity ranged from 40.32% to 43.24% and terminal velocity ranged between 2.12 and 2.98 m/s. Drag coefficient of 7.18 to 14.60, drag force of 0.002 to 0.003, and Reynolds number between 992.71 and 1579.20 were similarly obtained. The static angle of repose varied with material surfaces while the dynamic angle of repose varied with grain variety. The grain demonstrated 19° static angle of repose, which portrays a very free flow. All the geometric properties increased with moisture besides sphericity although insignificant. The thousand grain weight, volume of single grain, and porosity increased with moisture content. Nonetheless, bulk density decreased and true density remained the same as moisture content increased. Terminal velocity, Reynolds number, and drag force increased as drag coefficient decreased with moisture content. The frictional properties increased with moisture content, and the Reynolds number was <2100, hence, the linear flow of grain. Geometric, gravimetric, aerodynamic, and frictional properties of maize grain were dependent on both grain variety and moisture content.
Introduction
Undoubtedly, maize is globally among the important staple food crops. There are over 20 improved varieties of maize in Ghana, however low adoption and low replacement rate has been the challenge. This is partly because plant breeders generally fail to address the preferences and production challenges of farmers.[Citation1,Citation2 Plant breeders mainly center on; distinctness, uniformity, and stability; value for cultivation and use; preferred ecology, etc., but not the engineering and physical aspects of seeds. The anatomy of all cereal grains is similar, however, differences exist between various grains that can influence grain handling processes.
Mechanizing the different operations of grain handling like threshing, cleaning, grading, among others from harvesting through to storage can minimize operational cost. The design process of silos, bins, and hoppers is frequently thought of as “black art.” The flow properties and characteristics of the bulk material are required before bins and hoppers are chosen or designed.[Citation3,Citation4 The features of biological materials with air as the medium depends greatly on the aerodynamic properties of the materials.[Citation5–7] Also, when designing pneumatic separators, screen cleaners, gravity tables, harvesters, conveyors, and general pneumatic equipment, the aerodynamic properties of biomaterials are important preconditions.[Citation6,Citation8 This helps to optimize processing output and reduce inefficiencies associated with these types of equipment. Knowing the aerodynamic, gravimetric, geometric, and frictional properties of granular materials helps in transportation and separation.
The efficiency of agricultural machines is influenced by the axial dimensions of the agricultural material. Moreover, physical properties such as shape, size, mass, bulk density, true density, porosity, terminal velocity, sphericity, friction properties among others are all moisture-dependent.[Citation9–11] Although these physical properties are moisture-dependent, the effect that varietal differences may cause should not be glossed over.
To maintain machine efficiency and reduce grain loss or damage, different varieties of maize grains with similar moisture content and physical properties can be handled together. The design of similar equipment purposely for different maize grain varieties can be refrained when their physical characteristics are similar. Surprisingly, most farmers tend to simultaneously cultivate different maize varieties without taking cognizance of their physical differences, and mix up the grain. In the era of mechanization of grain handling, knowledge of maize grain varieties’ physical properties is cardinal. Most research conducted aimed only at moisture-dependent physical properties of maize grain but not variety-dependent properties. Hence, this study sought to determine the geometric, gravimetric, aerodynamic, and frictional properties of maize grain as affected by varietal differences and moisture content.
Materials and methods
Sample preparation
Honampa and Omankwa were bought from the Crop Research Institute of the Council for Scientific and Industrial Research in Fumesua, Ghana, while Abontem, Timtim, and Obatanpa were bought from Agrimat Ltd, Accra, Ghana. Timtim and Honampa (the moisture content not significantly different) and Abontem and Obatanpa (the moisture content not significantly different), and Omankwa had a different moisture content from the other four. Both Crop Research Institute and Agrimat Ltd are certified outlets for seed sales in Ghana.
Determination of moisture content of grain
Triplicate samples of 5.0 g of ground grain were put in Petri dishes and then placed in an air convection oven for 2 hours at 130°C.[Citation12 After cooling, the percent moisture content (wet-based) was then calculated.
Reconstitution of grain moisture content
The mass of distilled water to be added (Q, in kg) was calculated using the equation[Citation13:
where Wi = initial mass of sample in kg, Mf = final moisture content of the sample in % d.b., and Mi = initial moisture content of the sample in % d.b. The samples were then kept in separate polyethylene bags and sealed tightly and kept at 5°C for 1 week for moisture to be evenly.[Citation14,Citation15
Determination of geometric properties of maize grain
The dimensions [width (W), length (L), thickness (T)] of 100 grain were measured using a digital caliper. (i) geometric mean diameter, Dg in mm; from the average of axial dimensions, the geometric mean diameter Dg in mm was determined based on the equation[Citation16:
(ii) sphericity, Ø; calculated based on the equation[Citation16:
(iii) surface area, S in mm2; calculated from the equation[Citation17]:
(iv) projected area, Ap in m2; calculated using the equation[Citation18]:
Determination of gravimetric properties of maize grain
The following gravimetric properties of the maize grain were determined. Thousand-grain weight (TGW), grams; hundred grain in four replicates were weighed and the mass was multiplied by 10. Bulk density (ρb) in kgm−3; the modified method described by[Citation19] was used. An empty graduated cylinder was weighed (M0) and then filled with the grain to a volume. The mass of grain and cylinder was taken (M1). The bulk density was calculated as:
True density (ρt) in kgm−3 was determined by the fluid (Toluene) displacement method[Citation20,Citation21] with a known mass of grain in three replications. The volume of the grain was calculated from its mass and density. Volume of a single grain (V) in m3 was determined from the equation:
where TGM was the thousand-grain mass in kg, and ρt was the true density in kgm−3. Porosity (ε) in percentage was calculated from the equation[Citation9]:
where ρb was the bulk density in kg m−3.
Determination of aerodynamic properties of maize grain
The following aerodynamic properties of the maize grain were determined. Terminal velocity (Vt) in ms−1 was measured using a cylindrical air column.[Citation22] A single grain was dropped into the air stream from the top of the air column, and air with varying velocity was blown until the grain stayed suspended in the air stream. The air velocity near the location of the grain suspension was measured with an anemometer. The grain mass had 10 replications. Drag coefficient (Cd) was calculated based on the equation[Citation16:]
where m was the mass of grain in kg, g was the gravitational acceleration in ms−2 and ρa was the air density of 1.184 kgm−3, and ρt was the true density in kgm−3. Drag force (Fd) in N was computed using the equation:
where Cd was drag coefficient (dimensionless), la was air density (1.25, kgm−3), Ap was projected area of grain to air (m2), Vt was terminal velocity (ms−1). Reynolds number (Re) was calculated using the terminal velocity of each grain sample from the equation[Citation23]:
where Dg (in Equationequation 2)(2)
(2) was the geometric mean diameter of grain in m, μ was air viscosity (1.816 × 10–5 Nsm2 at room temperature).
Determination of frictional properties of maize grain
All the frictional properties were determined using stainless steel, aluminum, cardboard, and plywood surfaces. These materials are commonly used in handling equipment and storing structures. Static coefficient of friction (μ): a polyvinylchloride cylindrical pipe (50 mm diameter and 50 mm high) was placed on an adjustable tilting plate and filled with the grain sample. The cylinder was raised slightly so as not to touch the surface. The structural surface with the cylinder resting on it was raised gradually until the cylinder just started to slide down, and the angle of tilt was read from a graduated scale.[Citation24–26] Then μ was calculated using:
where α was the angle of tilt in degrees. Static angle of repose () in degrees: a polyvinylchloride cylindrical pipe (100 mm diameter, 150 mm high) was used. The cylinder was placed at the center of a raised circular plate and then filled with grain. The cylinder was raised slowly until the grain formed a cone on the plate. The height of the cone was measured, and the static angle of repose was calculated based on the equation[Citation27]:
where H was the height of the cone in mm and D was the diameter of the cone in mm. Dynamic angle of repose () in degrees: a hard cardboard box of dimensions 100*100*100 mm, which had a front sliding panel was filled with the grain, and the front sliding panel was quickly slid upward, allowing the grain to flow out and form a natural heap. The
was determined from measurements of grain height at two points (h1 and h2) in the sloping grain heap, and the horizontal distance between the two points (x1 and x2) based on the equation[Citation28]:
Statistical analysis
Mean values were compared using a one-way analysis of variance (ANOVA, Tukey-Kramer HSD at 5%), and graphs plotted using mean values ± standard deviation. Statgraphics Centurion 18 software and Microsoft Excel Spreadsheet 2013 were used for the statistical analysis and graphs, respectively.
Results and discussions
shows the similarity in initial moisture contents between the five varieties of maize grain studied. Timtim (hybrid) and Honampa (normal) had moisture contents that were not significantly different, and Abontem (hybrid) and Obatanpa (normal) similarly had moisture contents that were not significantly different. Therefore, varietal differences were discussed based on the four varieties.
Table 1. Initial moisture content (MCi) of five maize varieties used in the study
Effects of grain varietal differences (similar moisture content) on physical properties
The required energy for efficient processing during milling, conduction of heat, and selection of dehydrators rely on the geometrical properties of the material.[Citation29] Geometric properties such as geometric mean diameter, sphericity, surface area, and projected area are shown in . Grain varieties with the same moisture content had significantly different geometric mean diameters. The geometric mean diameters of Timtim and Honampa were different from each other, and Abontem and Obatanpa were also not similar. This is an indication that the different varieties although having similar moisture content exhibited geometric mean diameters differently from each other. This shows that the different varieties have different internal cell arrangements, and the screening or separating machine may not be the same.[Citation30] Grain geometric mean diameter is critical in determining the shape and aperture (screen holes sizes) in the engineering design of separating or screening equipment. Separating grain of similar moisture content but different geometric mean diameters may not be cost-effective and efficient since each variety may be separated with a differently designed shape and aperture. The sphericity of the studied maize varieties was within the range (0.32–1.00) as stated by[Citation16] of most agricultural produce. The tendency for grain to roll depends on its sphericity. Timtim and Honampa showed different sphericity, and surface areas while Abontem and Obatanpa had similar sphericity and surface area. A grain with higher sphericity rolls easily on any of its three axes, hence Timtim can roll easily on any of its axes compared with Honampa. Since the grain sphericity was between 70% and 80%, it can be argued that there are no vast differences between the hybrid and normal varieties. The surface area and other physical parameters are required for designing threshing cylinder, threshing element, thresher concave clearance, hoppers, sieves, etc., with regard to size and slope.[Citation31] The surface area and porosity help in assessing the rate of heat transfer during heating and drying, hence required in designing heat exchangers and dryers. Varieties of similar moisture content expressed different projected areas but the surface area was similar for Abontem and Obatanpa. Since the projected and surface areas were not uniform, the agricultural equipment may be designed or adjusted even when dealing with grain varieties with similar moisture content. According to,[Citation3] uniformity of bulk storage (particle size, shape, moisture content, etc.) is necessary because it influences particle segregation, production, and final product are affected.
Table 2. Geometric properties of five maize varieties
The gravimetric properties of the varieties are shown in . The thousand grain weight, bulk density, volume of single grain, and porosity differed among grain with similar moisture content (Timtim and Honampa, and Abontem and Obatanpa). It is worthy to note that, true density which is an important gravimetric property was not different among all the varieties. Gravimetric properties such as bulk density, true density, and porosity enable agricultural engineers to size grain hoppers and storage facilities.[Citation30] Hence the different varieties depicting different thousand grain weight, bulk densities, and volume of a single grain might require adjusting the sizes of hoppers and storage facilities for different varieties. Nonetheless, seed properties may vary from each other depending on the variety, growing conditions, etc., however, the design of planters are based on typical data.[Citation32]
Table 3. Gravimetric properties of five maize varieties
The aerodynamic properties of the grain varieties can be found in . Timtim and Honampa, and Abontem and Obatanpa expressed significant differences in terminal velocity, drag coefficient, drag force, and Reynolds numbers although for each pair the moisture contents were similar. The aerodynamic properties of the different varieties differed irrespective of the varieties having similar moisture content. This implies that in the design of pneumatic equipment, the air stream should be adjustable to control the air velocity depending on the grain variety been handled.
Table 4. Aerodynamic properties of five maize varieties
shows the frictional properties of the five maize grain varieties on four different material surfaces. Frictional properties of grain provide information about grain flowability. Grain are stored or handled using common material surfaces including aluminum, stainless steel, plywood, and cardboard. These surfaces influence the flow of grain and are important properties worth considering. The static coefficients of friction of Timtim and Honampa on the different material surfaces were not similar using aluminum and cardboard, however, the static coefficients of friction were similar using stainless steel and plywood. A similar observation was seen for Abontem and Obatanpa. Stainless steel and plywood can be used to handle grains with similar moisture contents so as not to experience differences in static coefficients of friction. Processing grain of similar moisture content using conveyor belts, gravity separators, and hoppers of silos and storage bins made with stainless steel and plywood may be cost-effective as it can handle different maize varieties of similar moisture. The static coefficient of friction is important because it measures the flowability of grain through hoppers with minimized friction. Bulk solids with high frictional properties may require a steep hopper angle to allow mass flow while bulk solid with low frictional properties may require a shallow hopper angle.[Citation3]
Table 5. Frictional properties of five maize varieties
The angle of repose is the angle that the surface of a granular pile under gravity makes with the horizontal.[Citation33] Storage bins and silos are typically constructed from metal or reinforced concrete although other varieties of materials are used.[Citation3] The different material surfaces showed differences in static angle of repose even for the different varieties with similar moisture content. The utilization of any of the studied materials when developing silos, storage bins, and hoppers may highly be dependent on grain variety. The varied static angles of repose mean silos and bins might have different holding capacities based on the used material surface. In ensuring uniform storage capacity for Timtim and Honampa aluminum may be used to construct silos and bins. Using cardboard, the static angles of repose for Abontem and Obatanpa were not different, therefore, ensuring uniform storage capacity cardboard may be used. Metals generally used in constructing bins and silos have enormous advantages compared to concrete silos.[Citation3]
The dynamic angles of repose of the four varieties (Timtim and Honampa, and Abontem and Obatanpa) were similar when the same material surfaces were used. Hence, different varieties with similar moisture had a similar dynamic angle of repose, so the same material can be used in grain handling. Almost all the grain varieties had a static angle of repose of around 19° or less which delineates a very free-flowing angle of repose, and a 10° angle of inclination.[Citation32]
Effect of moisture content on the physical properties of the grain varieties
shows the geometric properties of the maize grain varieties as affected by increases in grain moisture content. The geometric mean diameter, surface area, and projected area of all the five varieties increased with an increase in moisture content. But grain sphericity either remained the same or decreased with an increase in grain moisture content, however, these changes were generally not significant. The increase in geometric mean diameter, surface, and projected areas with the rise in moisture levels might be linked to the absorbed moisture filling the capillaries and voids in the grain subsequently leading to swelling of the grain, volume increase, and increase in physical properties of the grain. Sphericity (rolling ability) reduces as the moisture content of the grain increases. The decrease in sphericity was due to the decrease in the thickness of the grain as the grain increased in length and width resulting from the absorbed moisture. The increase in such properties influences pricing during the grain sale, hence overdrying of grain should be discouraged to prevent being short-changed. The current results are supported by the findings of[Citation9,Citation30,Citation31] who studied mung beans, locust beans, and sorghum grain, respectively. Studies by[Citation34] reported that different varieties of locust bean seeds showed differences in geometric mean diameter at different moisture levels.
Figure 1. Geometric properties (i, ii, iii, iv) of five maize varieties at two different moisture contents. MC1 = moisture content level 1, and MC2 = moisture content level 2.
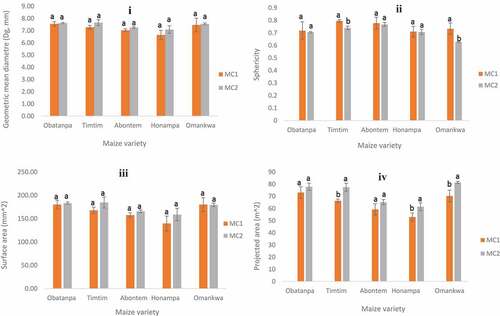
The effect of increased moisture content on gravimetric properties can be found in . Despite the increased grain moisture content, the true density of the grain did not significantly change. This could suggest that the mass gained by the grain was proportional to volume increase as moisture content increases. Therefore, foreign materials with true densities differently from maize grain can effectively be separated. Also, a significant decrease in bulk density as grain moisture increased was observed. The decrease in bulk density might be due to the increased bulk volume of grain being greater than the corresponding increase in grain mass. At increased moisture levels bulk density decreased in sorghum grain,[Citation31] and maize grain[Citation35] which support the findings of this study. There were significant increases in thousand grain weight, volume of single grain, and porosity with increased moisture content. Likewise, increases in thousand grain weight and volume of single grain were reported for maize kernel,[Citation36] and sorghum grain.[Citation31] Grain volume is practically applicable during separation and product loading, while thousand grain weight is important in the design of conveyors, transport, and storage equipment.
Figure 2. Gravimetric properties (i, ii, iii, iv, v) of five maize varieties at two different moisture contents. MC1 = moisture content level 1, and MC2 = moisture content level 2.
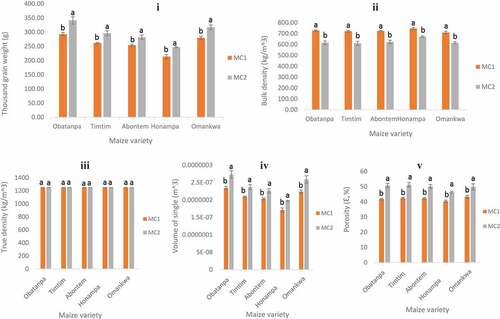
Porosity affects the resistance to airflow through a grain, therefore the high moisture grain with increased porosity can have a lower resistance to the escape of water vapor during drying. Hence, requires lower power to drive the aeration fans[Citation30] resulting in a lower drying cost.[Citation9] similarly reported increases in volume, porosity, and decrease in bulk density in mung beans when the moisture of the beans was increased. According to,[Citation30] the true density of locust beans was not affected but the bulk density was affected when the moisture was increased, which is in agreement with the current findings. Contrasting results of decreases in porosity of locust beans seeds, maize kernel, and sorghum grains were presented by,[Citation30,Citation31,Citation36] respectively. The significant increases in thousand grain weight, and volume of a single grain at a moisture content just above 14% may enhance profit gain. Moreover, the decrease in bulk density may enhance profit gain when grain moisture is 14% or a little above 14%. This is because at the grain elevator, notably, grain with higher moisture content than the market basis (>15%) attracts a discount either as shrink and drying fees or moisture discounts, while there is no premium paid for overdrying the grain.[Citation32]
Aerodynamic properties as influenced by the increased moisture content of the five grain varieties are shown in . At a higher grain moisture content, the terminal velocity increased significantly. Terminal velocity is important in the design of pneumatic conveyors, and for grain separation and transport using air. According to,[Citation37] terminal velocity is affected by the mass of the particle, projected surface area, drag coefficient, moisture content, and other constants (gravity and density). The increase in terminal velocity with increasing moisture content may be related to the increase in grain mass, projected area, and drag coefficient. The increases in the aforementioned parameters require higher airspeed to achieve terminal velocity, hence the obtained higher terminal velocity at elevated moisture levels. This suggests that in the design of pneumatic equipment, the source of air stream can be designed allowing for air velocity to be adjusted according to the moisture content of grain. In support,[Citation6,Citation8,Citation37] reported respectively, that the terminal velocity of watermelon seeds, safflower seeds, and tef grain increased with increased moisture content. Additionally, terminal velocity increased with a corresponding increase in moisture content for sorghum grain,[Citation31] and seeds of Makhobeli, triticale and wheat.[Citation5]
Figure 3. Aerodynamic properties (i, ii, iii, iv) of five maize varieties at two different moisture contents. MC1 = moisture content level 1, and MC2 = moisture content level 2.
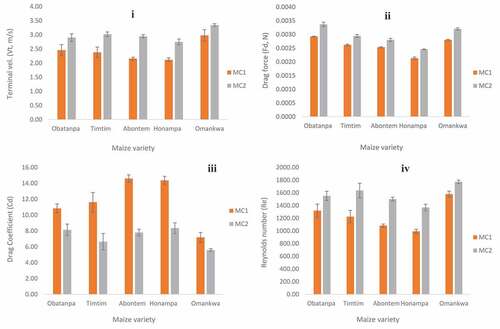
Reynolds number (Re) is a dimensionless number that correlates the inertia forces to the viscous forces.[Citation38] The Re obtained were less than 2100 demonstrating a laminar fluid flow. Thus, viscous force dominated over the inertia force, hence the grain flow was smooth and at a lower velocity. Although the grain moisture increased, the fluid flow did not reach the turbulent level (>2100). The Re increased significantly as moisture content increased. An increase in Re was also reported by[Citation6] for watermelon seeds. The increased terminal velocity and Re are indications that the cleaning and separation in an air column of all the grain varieties can smoothly be done at a fairly lower velocity.
The drag coefficient decreased while and drag force increased with grain moisture. Drag coefficient and drag force have fundamental significance in the design and sizing of equipment and structures.[Citation5] reported that the drag coefficient of Makhobeli, triticale and wheat seeds decreased with increasing moisture content. Similarly,[Citation37] indicated that the drag coefficient of tef grain decreased as moisture content increased. Both studies buttress the finding of this study. The decrease in drag coefficient contradicts the findings that showed the drag coefficient of coffee cherries and beans increased with increased moisture content.[Citation39] The increased grain moisture might have caused the magnitude of drag force to increase, and therefore the gravitational force was equaled. Consequently, a net force of zero was produced, and the grain velocity remained constant reaching the terminal velocity.[Citation40] Hence, the higher the grain drag force, the higher the terminal velocity at elevated moisture levels. Like friction, the drag force always opposes the motion of an object and depends on the shape, size, and velocity of the grain, and the fluid the grain is within.[Citation40]
The frictional properties of the five maize varieties are found in . The dynamic and static effects of friction of grain on material surfaces help to predict grain motion during the equipment design. The static coefficient of friction determines the angle at which chutes are positioned to attain consistent flow of materials through it,[Citation30] hence important for equipment designed for bulk flow of grainy materials. The static coefficient of friction, static angle of repose, and dynamic angle of repose were significantly high in grain with increased moisture content. The increased moisture content might have increased the static coefficient of friction owing to the increased adhesion between the grain and material surface.[Citation9]
Figure 4. Frictional properties (i, ii, iii) of five maize varieties at two different moisture contents. MC1 = moisture content level 1, and MC2 = moisture content level 2. the surfaces used were al = aluminum, ss = stainless steel, pl = plywood, and ca = cardboard.
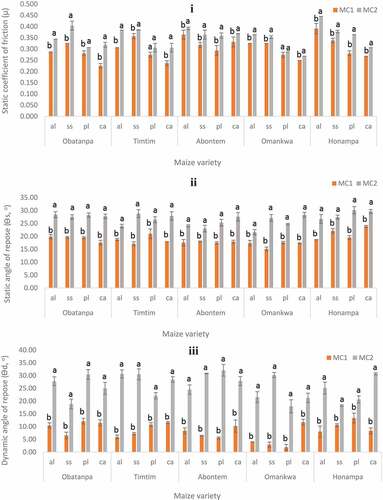
The lower the sphericity, the lower the ability of grain to roll over their three axes on material surfaces. Also, high moisture grain are sticky and difficult to slide and roll over one another. The hindrance to free flow and lower sphericity of grain might have therefore contributed to the higher angle of repose (static and dynamic). Generally, the angle of repose at increased moisture content was between 20° and 30°, representing a free-flowing angle of repose.[Citation32] Aluminum and stainless steel generally had a higher static coefficient of friction compared to the other surfaces. The increase in frictional properties on the material surfaces can maximize energy usage during grain cleaning and separation. Grain movement within storage containers, material surfaces, or through hoppers might be hindered as well at higher moisture, and that can affect equipment’s efficiency and output. Similarly, the storage capacity of silos and bins might reduce because of the increased angles of repose (static and dynamic). The coefficient of friction and angle of repose of sorghum grain[Citation31] and maize kernel[Citation36] increased with grain moisture, which are in agreement with the findings of this study.
Conclusion
The varieties with similar moisture content mostly differed in geometric properties, gravimetric properties, and aerodynamic properties. The Reynolds number and drag force increased while drag coefficient decreased as grain moisture content was increased. Generally, the different varieties exhibited varied static angle of repose based on the different material surfaces. However, the dynamic angle of repose dependent on the varietal type rather than material surface. The geometric properties, gravimetric properties, aerodynamic properties and frictional properties of the studied grain were dependent on grain variety and moisture content. Therefore, handling of grain of different varieties and moisture content may necessitate equipment adjustment.
Acknowledgments
The authors acknowledge the supports and efforts of all the national service personnel who helped in various ways during the laboratory analyses of this research.
Disclosure statement
No potential conflict of interest was reported by the author(s).
References
- Ragasa, C.; Dankyi, A.; Acheampong, P.; Wiredu, A. N.; Chapoto, A.; Asamoah, M.; Tripp, R. Patterns of Adoption of Improved Rice Technologies in Ghana. IFPRI Working Paper N 2013July, 35.
- Melomey, L. D.; Ayenan, M. A. T.; Marechera, G.; Abu, P.; Danquah, A.; Tarus, D.; Danquah, E. Y. Pre- and Post-Harvest Practices and Varietal Preferences of Tomato in Ghana. Sustainability. 2022, 14(3), 1436. Retrieved from. DOI: 10.3390/su14031436.
- Maynard, E. Ten Steps to an Effective Bin Design. CEP Magazine. 2013, November( November), 25–32. November http://www.aiche.org/resources/publications/cep/2013/november/ten-steps-effective-bin-design. Accessed 3 November 2021.
- Mehos, G.; Eggleston, M.; Grenier, S.; Malanga, C.; Shrestha, G., and Trautman, T. (2018). Designing Hoppers, Bins, and Silos for Reliable Flow. American Institute of Chemical Engineers (AIChE®), 501(c)(3). EIN: 13-1623892. Retrieved from https://www.aiche.org/resources/publications/cep/2018/april/designing-hoppers-bins-and-silos-reliable-flow. Accessed 3 November 2021.
- Shahbazi, F.; Valizadeh, S., and Dowlatshah, A. (2014). Aerodynamic Properties of Makhobeli, Triticale and Wheat Seeds, (2005), 389–394. Retrieved from DOI: 10.2478/intag-2014-0029. Accessed 20 October 2021.
- Obi, O. F. Evaluation and Modeling of the Aerodynamic Characteristics of Watermelon Seed of Different Varieties. Int. J. Food Prop. 2016, 19(10), 2165–2174. Retrieved from. DOI: 10.1080/10942912.2015.1113181.
- Audu, J. Development of a Measuring Apparatus for Aerodynamic Properties of Granular Materials. Mod. concepts dev. agron. 2018, 3(2), 1–5.
- Kara, M.; Bastaban, S.; Öztürk, I.; Kalkan, F.; Yildiz, C. Note Moisture-dependent Frictional and Aerodynamic Properties of Safflower Seeds. Int. Agrophys. 2012, 203–205. DOI: 10.2478/v10247-029-3.
- Unal, H.; Isk, E.; Izli, N.; Tekin, Y. Geometric and Mechanical Properties of Mung Bean (Vigna Radiata L.) Grain: Effect of Moisture. Int. J. Food Prop. 2008, 11(3), 585–599. Retrieved from. DOI: 10.1080/10942910701573024.
- Vashishth, R.; Semwal, A. D.; Murugan, M. P.; Raj, T. G.; Sharma, G. K. Engineering Properties of Horse Gram (Macrotyloma Uniflorum) Varieties as a Function of Moisture Content and Structure of Grain. J. Food Sci. Technol. 2020, 57(4), 1477–1485. Retrieved from. DOI: 10.1007/s13197-019-04183-w.
- Khanahmadzadeh, A.; Goli, H.; Ghamari, S. Moisture Dependent Physical Properties of Wild Safflower (Carthamus Oxyacanthus) Seeds. Agric Eng Int CIGR J. 2021, 23(3), 201–210.
- ISTA. International Rules for Seed Testing; Bassersdorf: Switzerland, 2005.
- Sacilik, K.; Ozturk, R.; Keskin, R. Some Physical Properties of Hemp Seed. Biosyst. Eng. 2003, 86(2), 191–198. DOI: 10.1016/S1537-5110(03)00130-2.
- Özarslan, C. Physical Properties of Cotton Seed. Biosyst. Eng. 2002, 83(2), 169–174 . DOI:10.1006/bioe.2002.0105.
- Baümler, E.; Cuniberti, A.; Nolasco, S. M.; Riccobene, I. C. Moisture Dependent Physical and Compression Properties of Safflower Seed. J. Food Eng. 2006, 72(2), 134–140. DOI: 10.1016/j.jfoodeng.2004.11.029.
- Mohsenin, N. N.;. Physical Properties of Plant and Animal Materials, 2nd ed. ed.; Gordon and Breach Science Publishers: New York, 1986.
- Baryeh, E. A., and Mangope, B. K. Some Physical Properties of QP-38 Variety Pigeon Pea. J. Food Eng. 2002, 56, 341–347. https://scialert.net/abstract/?doi=pjbs.2006.1799.1806. Accessed 1 August 2021.
- Asoiro, F. U., and Chidebelu, J. C. Effect of Moisture Content on Aerodynamic Properties of Corn Seed (Zea Mays). J. Agric. Eng. 2014, 22(4), 56–65. Retrieved from https://moam.info/nigerian-institution-of-agricultural-_5ba9efe8097c47e73e8b45c2.html. Accessed 4 August 2021.
- Okaka, J. C.; Potter, N. N. Physico-chemical and Functional Properties of Cowpea Powders Processed to Reduce Beany Flavour. J. Food Sci. 1979, 44(4), 1235–1240. Retrieved from. DOI: 10.1111/j.1365-2621.1979.tb03488.x.
- Mohsenin, N. N.;. Physical Properties of Plant and Animal Materials, 2nd ed. ed.; Gordon and Breach Science Publishers: New York, 1970.
- Yalçin, I.; Özarslan, C. Physical Properties of Vetch Seed Biosyst. Eng. 2004, 88(4), 507–512. DOI:10.1016/j.biosystemseng.2004.04.011.
- Joshi, D. C.; Das, S. K.; Mukherji, R. K. Physical Properties of Pumpkin Seeds J. Agri. Eng. Res. 1993, 54(3), 219–229. DOI:10.1006/jaer.1993.1016.
- Grega, L.; Anderson, S.; Cheetham, M.; Clemente, M.; Colletti, A.; Moy, W.; Talarico, D.; Thatcher, S. L.; Osborn, J. M. Aerodynamic Characteristics of Saccate Pollen Grains. Int. J. Plant Sci. 2013, 174(3), 499–510. DOI: 10.1086/668694.
- Dutta, S. K.; Nema, V. K.; Bhardwaj, R. K. Physical Properties of Gram J. Agri. Eng. Res. 1988, 39(4), 259–268. DOI:10.1016/0021-8634(88)90147-3.
- Singh, K. K.; Goswami, T. K. Physical Properties of Cumin Seed J. Agri. Eng. Res. 1996, 64(2), 93–98. DOI:10.1006/jaer.1996.0049.
- Suthar, S. H.; Das, S. K. Some Physical Properties of Karingda [Citrullus Lanatus (Thumb) Mansf] Seeds J. Agri. Eng. Res. 1996, 65(1), 15–22. DOI:10.1006/jaer.1996.0075.
- Ozguven, F.; Vursavus, K. Some Physical, Mechanical and Aerodynamic Properties of Pine (Pinus Pinea) Nuts. J. Food Eng. 2005, 68(2), 191–196. DOI: 10.1016/j.jfoodeng.2004.05.031.
- Bart-Plange, A.; Baryeh, E. A. The Physical Properties of Category B Cocoa Beans J. Food Eng. 2003, 60(3), 219–227. DOI:10.1016/S0260-8774(02)00452-1.
- Singh, K. P.; Mishra, H. N.; Saha, S. Moisture-dependent Properties of Barnyard Millet Grain and Kernel. J. Food Eng. 2010, 96(4), 598–606. Retrieved from. DOI: 10.1016/j.jfoodeng.2009.09.007.
- Bamgboye, I.; Sadiku, O. A. Moisture-dependent Physical Properties of Locust Bean (Parkia Biglobosa) Seeds. Future of Food: Journal on Food, Agriculture and Society. 2015, 3(2), 27–40.
- Sabar, S. S.; Swain, S. K.; Behera, D.; Rayaguru, K.; Mohapatra, A. K.; Dash, A. K. Moisture Dependent Physical and Engineering Properties of Sorghum Grains. Agric. Eng. Int. 2020, 9(8), 2365–2375.
- Bern, C. J.; Hurburgh, C. R., Jr; Brumm, T. J. Managing Grain After Harvest. In Abe 469/569. Iowa State Bookshop: Ames, 2014.
- Mehta, A.; Barker, G. C. The Dynamics of Sand. Rep. Prog. Phys. 1994, 57(4), 383–416. Retrieved from. DOI: 10.1088/0034-4885/57/4/002.
- Sobukola, O. P.; Onwuka, V. I. Effect of Moisture Content on Some Physical Properties of Locust Bean Seed (Parkia Fillicoidea L.). J. Food Process Eng. 2011, 34(6), 1946–1964. Retrieved from. DOI: 10.1111/j.1745-4530.2009.00511.x.
- Yenge, G. B.; Kad, V. P.; Nalawade, S. M. Physical Properties of Maize (Zea Mays L.) Grain. Journal of Krishi Vigyan. 2018, 7(special), 125. Retrieved from. DOI: 10.5958/2349-4433.2018.00173.3.
- Sangamithra, A.; John, S. G.; Sorna Prema, R.; Nandini, K.; Kannan, K.; Sasikala, S.; Suganya, P. Moisture-dependent Physical Properties of Maize Kernels. Int. Food Res. J. 2016, 23(1), 109–115.
- Awgichew, A. Aerodynamic Properties of Tef for Separation from Chaff. 2019, 113, 39–43. DOI:10.7176/CER.
- Rapp, B. E. Fluids. Microfluidics: Modelling, Mechanics and Mathematics; Retrieved from Elsevier, 2017; 243–263. 10.1016/B978-1-4557-3141-1.50009-5
- Afonso Júnior, P. C.; Corrêa, P. C.; Pinto, F. A. C.; Queiroz, D. M. Aerodynamic Properties of Coffee Cherries and Beans Biosyst. Eng. 2007, 98(1), 39–46. DOI:10.1016/j.biosystemseng.2007.03.010.
- Lumen, L.; (2016). Drag Force and Terminal Speed. Retrieved October 20, 2021, from https://courses.lumenlearning.com/suny-osuniversityphysics/chapter/6-4-drag-force-and-terminal-speed/.