ABSTRACT
Vitamin C is a compound that is widely used in food processing as a preservative and antioxidant. The weakness of vitamin C is that it is unstable and very reactive to environmental conditions. To maintain the stability of vitamin C during processing and storage, emulsification was carried out with a double emulsion Pickering water in oil in water (W/O/W) system. The research method used was descriptive analysis method which consists of two stages. The first stage was to determine the right amount of emulsifier in the manufacture of W/O emulsion and the second stage is the manufacture of W/O/W emulsion which consists of four different treatments with microcrystalline cellulose (MCC) as the Pickering component. The results showed that the use of 5% sorbitan tristearate produced a stable W/O vitamin C emulsion at 14 days of storage with a coalescence index of 3.2% and a droplet size of 0.698 μm. The combination of polysorbate 80 and MCC produced a W/O/W emulsion with a droplet size of 0.952 μm, creaming index 2.615%, serum index 2.899%, and a lowest increase in IC50 of 0.32 ppm/day.
Introduction
Vitamin C is a common descriptor for all compounds that show qualitative biological activity of ascorbic acid, and in low doses, vitamin C is often used in food processing to maintain shelf life and improve taste.[Citation1] The metabolic functions of vitamin C can be categorized based on its properties as an antioxidant and as an enzyme co-substrate. Vitamin C could lose electrons easily due to its reversible monovalent oxidation to ascorbyl radicals; this functions as a biochemical redox system. Properties of vitamin C are unstable and reactive to environmental conditions.[Citation2] Therefore, the food manufacturing industry must find the right strategy to increase the shelf-life stability of Vitamin C-fortified functional foods and beverages .[Citation3]
The water-in-oil-in-water (W1/O/W2) double emulsion encapsulation system is a method for coating a hydrophilic bioactive compound such as vitamin C. W1/O/W2 consists of an internal aqueous phase (W1), an intermediate phase (O), and an external aqueous phase (W2). The right emulsifier needs to be considered in the formation of W1/O/W2 double emulsions. Sorbitan tristearate as an emulsifier in the W1/O phase has good potential because it shows a strong viscoelastic effect when spread on the surface.[Citation4] The use of the right amount of sorbitan tristearate emulsifier in the W1/O phase is important to know how to produce a stable double emulsion during processing and storage of vitamin C products.
Biopolymers such as proteins and polysaccharides are high molecular weight hydrophilic emulsifiers which can be applied in the external aqueous phase in W1/O/W2 double emulsions. Whey protein has excellent emulsifying properties and can be used alone or as a complex with polysaccharides such as pectin in the external aqueous phase of W1/O/W2 emulsions. Low molecular weight emulsifiers such as polysorbate 80 are generally used as suitable synthetic emulsifiers in multiple emulsions.[Citation5]
One method to produce emulsions with high stability is through the use of Pickering-type emulsions.[Citation6] Microcrystalline cellulose (MCC) can be used in beverage products as a thickener because of its high viscosity, so it can also be used to stabilize Pickering emulsions.[Citation7] The use of MCC as a Pickering component in the vitamin C double emulsion was studied in this research to determine the stability of the antioxidant activity of the vitamin C double emulsion during storage.
Materials and methods
The materials used in this research include Vitamin C (Merck), Aqua demineral (Brataco), commercial soybean oil (Salim Ivomas), sodium chloride (Brataco), magnesium sulfate (Brataco), microcrystalline cellulose (Vivapur), emulsifier polysorbate 80 (Tween 80–Croda), sorbitan tristearate (Span 65–Futura), pectin, and whey protein concentrate (Avonlac). The materials used to test the stability of the antioxidant activity of the emulsion were 1,1-diphenyl-2-picrylhydrazy (DPPH—Sigma Aldrich) and Methanol (Merck).
Preparation of W1/O Emulsion
Phase 1 research aimed to determine the use of sorbitan tristearate as an emulsifier on the stability of the W1/O vitamin C emulsion. The aqueous phase 1 (W1) was prepared with reference to Ref. [Citation8], where 10% (w/v) of vitamin C was dissolved and 2% (w/v) of MgSO4 was added to maintain osmotic pressure. A W1/O emulsion was prepared using the aqueous phase 1 and an oil (O) phase containing the emulsifier Sorbitan tristearate in five concentrations 1%, 2%, 3%, 4%, and 5% (w/v). Demineralized water was used as a medium for all aqueous phases. A 30% aqueous phase was added to 70% oil phase at room temperature (about 25°C), followed by first-stage emulsification at 10,000 rpm for 5 min using a homogenizer (DragonLab D500, China).
W2 phase preparation
Phase 2 of the study aimed to determine the effect of the addition of MCC on the stability and antioxidant activity of the W1/O/W2 double Pickering emulsion during storage. The DPPH analysis was performed to measure the peroxyl radical scavenging activity of the samples. Whey protein concentrate (WPC)–pectin and WPC–MCC solutions were prepared with reference to Ref. [Citation9], WPC and pectin were dissolved in aqua demineral at 50°C for 30 min, then allowed to stand for 24 h at room temperature for complete hydration. The final concentration of the biopolymer solution consisted of 2.5% WPC and 1.25% pectin [w/v]. The preparation of polysorbate 80 and polysorbate–MCC solutions refers to Ref. [Citation10], with minor modifications in MCC addition. A 3.5% (w/v) polysorbate 80 and 1.25% MCC were dissolved into demineralized water at room temperature, 3.75% (w/v) NaCl was added to maintain the osmotic pressure of the emulsion system to be formed. The solution was then stored at 4°C 12 h before being used.
Preparation of W1/O/W2 emulsion
The W1/O/W2 emulsion was obtained by dispersing the best W1/O emulsion from the first phase into the outer phase (W2). MCC was added as much as 1% w/v of the total volume of the W1/O/W2 emulsion. The volume of the W1/O emulsion fraction was set at 20%. The W1/O emulsion phase was added to the aqueous phase at a total volume of 100 mL at room temperature, followed by emulsification at 1200 rpm for 5 min followed by sonication (QSonica 500, Newton, USA) with a frequency of 20 kHz and an amplitude of 70%, pulse 3 s on, 3 s off for 15 min. The W1/O/W2 emulsion formed was stored in a dark glass bottle at 4°C to be analyzed for stability in 28 days. Detailed material for every treatment are showed in .
Table 1. Emulsion material for each treatment.
P80–MCC: Polysorbate 80–microcrystalline cellulose
P80: Polysorbate 80
WPC–MCC: Whey protein concentrate–microcrystalline cellulose
WPC–P: Whey protein concentrate–pectin
Analysis
Analysis was made on the W1/O emulsion to determine the stability of the emulsion during storage and the W1/O/W2 double Pickering emulsion to determine the stability and antioxidant activity during storage. Particle size was measured using the particle size analyzer (Beckman Coulter LS 13 320). The measurements were done on the same day with emulsion preparation. One milliliter emulsion sample was diluted in the glass cell filled with distilled water (50 mL). Creaming index measured according to the W1/O/W2 vitamin C emulsion was transferred in a 50-mL test tube to a height of 6 cm approximately and stored at 4°C. A weekly height of the cream at the upper emulsion was measured (ht) and compared to the initial emulsion height (h0) to determine the creaming index (CI) with equation.
Creaming index (%) = (h0 − h1) × 100
Flow behavior index was evaluated using viscometer (RAYPA Viscometer RP1) at 50, 100, and 200 rpm. The n value was calculated using equation:
μApp = (4πN/n) × n − 1
where μApp is the apparent viscosity, N is the rpm data from viscometer, and n is the flow behavior index. Emulsion structure image was captured using transmission electron microscopy (TEM, JEOL JEM 1010) with uranyl acetate staining according to method.[Citation11] Five microliter of sample was placed into a 400-mesh grid for 10 min before uranyl acetate staining. The grid was then loaded into the instrument to observe at 20,000–30,000× of magnification. Zeta potential of the emulsion was measured using a zeta potential measurement [Horiba SZ 100] according to Ref. Citation[12], samples were diluted 1:100 using distilled water 1 day after emulsion preparation.
Antioxidant activity was analyzed using 1,1-diphenyl-2-picrylhydrazyl [DPPH] assay according to the method of,Citation13 A reference cuvette was prepared by mixing 2 mL methanol and 0.5 mL DPPH stock solution (160 ppm) and the measuring cuvettes (five dilution solutions, e.g., 40, 20, 10, 5, 2.5 ppm) were prepared by mixing the sample, DPPH stock solution (160 ppm), and methanol with a total volume 2.5 mL. Measurements using spectrophotometer UV-9200 (Beijing Rayleigh Analytical Instrument Co., Ltd., China) with wavelength 517 nm were performed after the reference and measuring cuvettes were kept in the dark at room temperature.
Result and discussion
W1/O/W2 emulsion structure
The structure image of the W1/O/W2 vitamin C emulsion was taken using. Observations were made at a magnification of 20,000–30,000× to capture the emulsion structure clearly. is an image of the emulsion structure with Polysorbate 80 emulsifier, the large black area is a W1/O emulsion system, the white droplets contained in the W1/O system are probably vitamin C solution which is still stable in system. Around the droplet there are also oil droplets which are separated from the system and form an O/W2 system. A similar structure is also shown in where there is a W1/O system, the difference is the presence of black spots around the droplets which are suspected to be MCC. MCC appears to fill the space between droplets and strengthens the emulsion structure formed. showed the WPC–MCC emulsifier appears to form a large biopolymer complex in the system and protects the trapped droplets. The formation of the biopolymer complex causes the particle measurement results to produce high numbers. Emulsions with WPC-pectin in were also seen to form biopolymer complexes with less irregular shapes such as WPC–MCC.
Figure 1. W1/O/W2 emulsion structure a. Emulsion with Polysorbate 80 b. Emulsion with polysorbate–MCC c. Emulsion with WPC–MCC d. Emulsion with WPC–pectin.
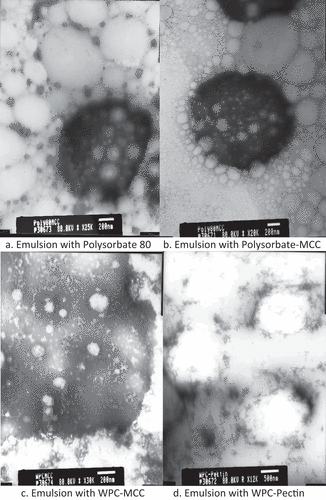
The mass thickness contrast method is the principle of taking image using TEM, where the higher the molecular mass of a compound, the darker the visible color will be.[Citation14] The image shows that the W1/O system in the emulsion has a higher mass than the W2 phase making a difference in the color of the dark and light colors. The same results were also found by Ref. [Citation15], where the results of the TEM test showed large dark-colored droplets with clear droplets in a W1/O/W2 emulsion system containing arbutin and emulsified using WPC–pectin, dark-colored droplets indicating the oil phase and clear droplets in it indicate the aqueous phase containing arbutin.
The W1/O/W2 system also showed the presence of clear droplets scattered among the W1/O droplets, these droplets were most likely oil released from the W1/O phase due to the use of energy from ultrasound during emulsion formation. Ref. [Citation16] stated that the greater the amplitude used, the ultrasonic waves will change the protein to be more polar and the fat-binding strength of the protein will decrease. Proteins can also be damaged during sonication due to large amplitudes producing large amounts of heat, thereby accelerating the denaturation process of the protein and causing the protein to be unable to bind to the W1/O emulsion formed.[Citation17] W1/O/W2 emulsion using polysorbate 80–MCC and polysorbate 80 had a clearer emulsion system than WPC–MCC and WPC–pectin based on TEM observations.
Stability of W1/O emulsion
The use of sorbitan tristearate at five different concentrations resulted in emulsions with different stabilities. The 5% concentration of sorbitan tristearate showed a stable W1/O emulsion at room temperature storage for 14 days. shows the oil separation (coalescence) occurring on day 7 for the concentration of sorbitan tristearate less than 4%, while at a concentration of 5% oil separation began to occur on day 14. Separation of oil from the emulsion is called coalescence as shown in . Coalescence is also called a separation process where the emulsion droplets combine to form larger droplets and can separate from the external phase of the system.[Citation18] The coalescence process in emulsions is an irreversible process where droplets join where the droplets are close to each other, the use of a low emulsifier can also cause high intersurface tension so that it reduces the stability of the emulsion.[Citation19]
Table 2. Coalescence of W1/O emulsion.
The increase in the concentration of sorbitan tristearate is directly proportional to the viscosity of the emulsion produced; this caused the emulsion to be more stable with Brownian movement due to gravity that can be minimized.[Citation20] Another factor that increases the stability of the emulsion is the droplet size of the emulsion. Small droplet size can increase the stability of the emulsion against separation by gravity (Stoke’s law) and prevent coalescence.[Citation12] Sufficient amount of emulsifier combined with the right emulsification technique can produce smaller droplet sizes so that it has an impact on preventing creaming and coalescence.[Citation21]
Based on , the results showed that the use of an 5% emulsifier resulted in the lowest emulsion particle size of 0.698 μm. The particle size of the emulsion tends to decrease with the addition of the emulsifier sorbitan tristearate used. Increasing the concentration of the emulsifier can decrease the particle size along with the increase in the surface area that captures the dispersed phase.[Citation22] In the emulsification process using a homogenizer, the water-phase droplets break down into smaller sizes and are distributed into the oil phase.
Table 3. Particle size of W1/O emulsion.
Stability of W1/O/W2 emulsion
Stability of the W1/O/W2 emulsion was seen from the creaming index and serum index values. Creaming index can be calculated by comparing the height of the cream layer formed with the overall emulsion height and becomes an indicator of the stability of the emulsion during storage.[Citation12] showed W1/O/W2 vitamin C emulsion made with nonionic polysorbate 80–MCC emulsifier had the lowest creaming index value after 28 days of storage at 2.615%, followed by WPC–MCC emulsifier 4.131%, WPC–pectin 5.256%, and polysorbate 80 8,519%.
The formation of a cream layer began to form since the seventh day of storage for all types of emulsifiers which indicated the incorporation of W1/O emulsion droplets in the system which formed a new emulsion with a higher density. The particle droplet size also affects the cream layer formed; from observations, it can be seen that the addition of MCC in the W2 phase can produce smaller droplet sizes in both polysorbate 80 and WPC emulsifiers, implicating slower creaming process that occurs during storage and affected the value of creaming index. MCC plays a role in forming a matrix in the emulsion system so it can prevent droplets from moving freely and joining other droplets.[Citation23] The increase in the concentration of MCC as a Pickering component can be evaluated to adjust the droplet size to produce and it has a direct impact on the stability of the emulsion.[Citation24]
High value of creaming index in WPC–pectin emulsifier can be caused by flocculation in the components of the emulsion system formed. Ref. [Citation8] stated that the speed of creaming is influenced by the flocculation that occurs in the components of the W1/O emulsion system. Flocculation happened due to the droplet aggregation process, thus accelerating the process of creaming.[Citation25] The flocculation process is the process that most influences the occurrence of creaming in an emulsion system, it has a big role because the flocculated droplets move faster than individual droplets in the emulsion solution.[Citation26]
(a: P80–MCC, b: P80, c: WPC–MCC, d: WPC–pectin)
showed the layer formed on the W1/O/W2 emulsion is divided into three, namely the cream layer at the top, the emulsion in the second layer, and the serum in the base layer. Emulsions with polysorbate 80 have a lower serum layer and cloudiness when compared to WPC emulsifiers. This is another indication of the instability of the emulsion during storage. The serum index value in also showed the emulsion with MCC added was lower when compared to the emulsion without the addition of either the polysorbate 80 or WPC emulsifier.
The increase in the serum index value was also influenced by the droplet particle size factor which caused coalescence and separation during emulsion storage. According to Ref. [Citation27], the phenomenon of serum formation is due to the emulsifier with hydrocolloid (pectin/MCC) which is sensitive to gravity separation after flocculation occurs, the cloudy layer at the bottom (serum) is water which has a heavier molecular weight than oil. The flocculation impacts the emulsifier bonds within oil droplets causing the formation of serum.
Emulsion instability is influenced by the amount of surfactant used. The low concentration of WPC cannot bind the two components as a whole, resulting in separation and flocculation which eventually results in the creaming and seruming process.[Citation10] The lack of WPC to meet the formed W1/O emulsion droplet surface causes pectin and MCC as polysaccharides or hydrocolloids to be unable to stabilize electrostatically.[Citation26] Due to the lack of protein concentration, protein can act as a bridge for oil droplets to flocculate.
Flow behavior index and zeta potential
Measurement of flow behavior index was carried out on the sample on day 0 to determine the flow properties of the emulsion formed. shows the results of the n-value calculation which were tested at a speed of 50 rpm using an L1 spindle. W1/O/W2 emulsion with polysorbate 80–MCC had the highest n value followed by WPC–MCC, WPC–P, and polysorbate 80 treatments, respectively. The higher the viscosity of an emulsion system, the emulsion formed has a more stable potential.[Citation28]
Table 4. N value of W1/O/W2 emulsion.
The fusion of droplets can be inhibited due to slow droplet movement in high viscosity emulsion.[Citation29] Emulsion systems are fragile, when the viscosity is high, the droplet will change slower (flocculation, coalescence) correlating the emulsion becomes more stable.[Citation26WPC–MCC and WPC-pectin emulsifiers should produce a higher viscosity emulsion system than polysorbate 80 emulsifiers because the hydrocolloids can increase the viscosity and stabilize the emulsion system.[Citation30] The low viscosity of WPC can be caused by the lack of WPC concentration impacting poor stability of the emulsion completely and the viscosity becomes low.[Citation28]
showed samples polysorbate 80 has n value less than 1 indicating that the W1/O/W2 vitamin C emulsion formed is included in a pseudoplastic non-Newtonian solution based on the “Power Law” theory.[Citation31] Pseudoplastic solutions are non-Newtonian solutions which have a lower viscosity as the force applied to the solution.[Citation28] This means that a pseudoplastic non-Newtonian solution will become dilute the longer it is stirred. Sample polysorbate 80–MCC, WPC–MCC, and WPC–P were on opposite with n value more than 1 indicate the solution is dilatant, dilatant solutions have a higher viscosity when force applied and impact on the stability of the emulsion during storage
Zeta potential is the electrical surface property of a colloidal solid suspended in a liquid and indicates the criteria for electric attraction and repulsion at the interface between solids. A large zeta potential value indicates a stable solid without agglomeration, when coagulation between solids occurs due to gravity, the liquid stability decreases and the repulsion between solids decreases impacting zeta potential value decreases.[Citation32] In general, the higher the absolute value of the zeta potential (±30 mV) will increase the repulsion between surfaces and have a direct impact on better emulsion stability.[Citation21]
showed W1/O/W2 vitamin C emulsions with WPC–MCC and WPC–pectin have zeta potential values closer to absolute values, followed by polysorbate 80 and polysorbate 80–MCC treatments. All treatments experienced a change in the zeta potential value toward its absolute value at 28 days of storage. The zeta potential value getting closer to the absolute value indicates that the repulsion between droplets is getting higher with increasing stability.[Citation9] WPC-based emulsifiers have a good zeta potential value caused by the protein isoelectric point being higher at pH 5.2,[Citation33] in contrast to polysorbate 80 which is classified as a nonionic emulsifier does not have a positive/negative effect on the value of zeta potential.[Citation10] The isoelectric point of MCC is in the pH range of 2.8, where at a pH below that MCC is negatively charged and has better repulsion.[Citation34] The zeta potential value that is not close to the absolute value was also obtained in the research of Ref. [Citation23], which used MCC in the manufacture of Pickering emulsions, the use of higher MCC compared to CMC increased the emulsion repulsion and stability during storage but did not reach the absolute zeta potential value.
Table 5. Zeta potential of W1/O/W2 emulsion.
Antioxidant activity of W1/O/W2 emulsion
The antioxidant activity analysis was carried out on the W1/O/W2 vitamin C emulsion during storage for 35 days at 4°C, as a control was also carried out on vitamin C solution with the same concentration for further testing of the IC50 value. showed the control treatment had the lowest IC50 value on day 0 followed by polysorbate 80–MCC, WPC–pectin, polysorbate 80, and WPC–MCC treatments. The control treatment had the lowest IC50 value on day 0 compared to other treatments due to the presence of energy in the form of homogenization and ultrasound during the emulsification process, causing some damaged vitamin C in the W1/O/W2 emulsion system.[Citation35] Emulsion with polysorbate 80–MCC had the lowest IC50 value on day 0 compared to other treatments, which was 30,886 ppm, followed by WPC–pectin, polysorbate 80, and WPC–MCC treatments.
The use of hydrocolloid components such as pectin and MCC can protect the active components in the emulsion system, also reducing damage during the energizing process during emulsion formation.[Citation36,Citation37] The selection of low energy emulsification techniques such as membranes can be used to prevent damage to active components, increase droplet uniformity, and prolong emulsion stability.[Citation38]
The use of emulsifier polysorbate 80 and MCC showed the best stability compared to other treatments. This can be seen from the increase in IC50 value after 35 days of storage in . Emulsion with polysorbate 80–MCC increased IC50 value by 0.32 ppm/day then WPC–MCC by 0.42 ppm/day, WPC–pectin 0.63 ppm/day, and polysorbate 80 1.85 ppm/day. The increase in the low IC50 value indicates that the emulsifier polysorbate 80 and MCC have the best ability in terms of maintaining the active components contained in the W1/O/W2 emulsion system. The ability of MCC to resist damage to the active components in the system due to the addition of hydrocolloids has protective properties against oxidation during storage.[Citation33] Pickering components can slow down the transfer of active components from the W1/O system to the outer phase, this property being a good potential to regulate the release of active components compared to using an emulsifier without the addition of MCC.[Citation10,Citation24,Citation39] The combination of polysorbate 80 and MCC was able to inhibit the increase in IC50 values better. MCC produces emulsions with lower droplet sizes, better creaming, and serum index, so that it has a direct impact on emulsion stability and protection of the active components in it.[Citation7,Citation40]
–Conclusion
Based on the results of the study, it can be concluded that the use of 5% sorbitan tristearate can produce a W1/O vitamin C emulsion which is stable during 14 days of storage with low particle size and coalescence index. W1/O/W2 vitamin C emulsion with polysorbate 80–MCC emulsifier had the best stability, in terms of the emulsion structure formed, creaming index, low serum index values, and highest zeta potential value. The use of polysorbate 80–MCC as an emulsifier can protect the antioxidant activity of the W1/O/W2 vitamin C emulsion after storage for 28 days, with an increase in IC50 value of 0.3216 ppm/day.
Disclosure statement
No potential conflict of interest was reported by the author(s).
References
- Combs, G. F.; McClung, J. P. Vitamin C. Vitamin Vol. Chapter 10. 2017. DOI: 10.1016/B978-0-12-802965-7.00010-1.
- Chang, S. K.; Ismail, A.; Daud, Z. A. M. Ascorbic Acid: Properties, Determination and Uses. Encyclopedia of Food and Health, 1st; Elsevier Ltd, 2016. DOI: 10.1016/B978-0-12-384947-2.00044-1.
- Nelson, H. N.; Rush, K. L.; Wilson, T. Functions of Common Beverage Ingredients. Beverage Impacts on Health and Nutrition; Switzerland: Springer International Publishing, 2016; pp 317–329. DOI: 10.1007/978-3-319-23672-8.
- Erni, P.; Fischer, P.; Windhab, E. J. Sorbitan Tristearate Layers at the Air/Water Interface Studied by Shear and Dilatational Interfacial Rheology. Langmuir. 2005, 21(23), 10555–10563. DOI: 10.1021/la0514664.
- Tamnak, S.; Mirhosseini, H.; Tan, C. P.; Amid, B. T.; Kazemi, M.; Hedayatnia, S. Food Hydrocolloids Encapsulation Properties, Release Behavior and Physicochemical Characteristics of Water-in-Oil-in-Water (W/O/W) Emulsion Stabilized with Pectin E Pea Protein Isolate Conjugate and Tween 80. Food Hydrocolloids. 2016, 61, 599–608. DOI: 10.1016/j.foodhyd.2016.06.023.
- Matos, M.; Anna, T.; Malin, S.; Petr, D.; Marilyn, R. Preparation and Encapsulation Properties of Double Pickering Emulsions Stabilized by Quinoa Starch Granules. Colloids Surf. A. 2013. 2010. 10.1016/j.colsurfa.2013.01.060.
- Xu, D.; Zhang, J.; Cao, Y.; Wang, J.; Xiao, J. Influence of Microcrystalline Cellulose on the Microrheological Property and Freeze-Thaw Stability of Soybean Protein Hydrolysate Stabilized Curcumin Emulsion. LWT - Food Sci. Technol. 2016, 66, 590–597. DOI: 10.1016/j.lwt.2015.11.002.
- Khalid, N.; Isao Kobayashi, M. A.; Neves, K. U.; Nakajima, M. Preparation and Characterization of Water-in-Oil-in-Water Emulsions Containing a High Concentration of L-Ascorbic Acid. Biosci. Biotechnol. Biochem. 2013, 77(6), 1171–1178. DOI: 10.1271/bbb.120870.
- Mohammadi, A.; Jafari, S. M.; Esfanjani, A. F.; Akhavan, S. Application of Nano-Encapsulated Olive Leaf Extract in Controlling the Oxidative Stability of Soybean Oil. Food Chem. 2016, 190, 513–519. DOI: 10.1016/j.foodchem.2015.05.115.
- Gharehbeglou, P.; Mahdi, S.; Hamishekar, H.; Homayouni, A.; Mirzaei, H. Pectin-Whey Protein Complexes Vs. Small Molecule Surfactants for Stabilization of Double Nano-Emulsions as Novel Bioactive Delivery Systems. J. Food Eng. 2019, October 2018, 245, 139–148. DOI: 10.1016/j.jfoodeng.2018.10.016.
- Scarff, C. A.; Fuller, M. J. G.; Thompson, R. F.; Iadaza, M. G. Variations on Negative Stain Electron Microscopy Methods: Tools for Tackling Challenging Systems. J. Visualized Exp. 2018, 2018(132), 1–8. DOI: 10.3791/57199.
- Hong, I. K.; Kim, S. I.; Lee, S. B. Effects of HLB Value on Oil-in-Water Emulsions: Droplet Size, Rheological Behavior, Zeta-Potential, and Creaming Index. J. Ind. Eng. Chem. 2018. DOI: 10.1016/j.jiec.2018.06.022.
- Molyneux, P. The Use of the Stable Free Radical Diphenylpicryl-Hydrazyl (DPPH) for Estimating Anti-Oxidant Activity. Songklanakarin J. Sci. Tech. 2004, 26(May), 211–219.
- Harris, J. R.; Bhella, D.; Adrian, M. Recent Developments in Negative Staining for Transmission Electron Microscopy. In Microscopy and Analysis; UK: Wiley Analytical Science, 2006; pp 17–21.
- Huang, H.; Belwal, T.; Aalim, H.; Li, L.; Lin, X.; Liu, S.; Qunhe, L.; Zou, Y.; Luo, Z. Protein-Polysaccharide Complex Coated W/O/W Emulsion as Secondary Micro- Capsule for Hydrophilic Arbutin and Hydrophobic Coumaric Acid. Food Chemistry. 2019, 300. DOI: 10.1016/j.foodchem.2019.125171.
- Ojha, K. S.; Tiwari, B. K.; Donnell, C. P. O. Effect of Ultrasound Technology on Food and Nutritional Quality. In Advances in Food and Nutrition Research; Elsevier, 2018. DOI: 10.1016/bs.afnr.2018.01.001.
- Vélez-erazo, E. M.; Consoli, L.; Hubinger, M. D. Mono and Double-Layer Emulsions of Chia Oil Produced with Ultrasound Mediation. Food Bioprod. Process. 2018, 112, 108–118. DOI: 10.1016/j.fbp.2018.09.007.
- Viana, C.; Bohrer, D.; Machado de Carvalho, L.; Cícero Do Nascimento, P.; da Rosa, M. B. Emulsified Systems for Metal Determination by Spectrometric Methods. TrAC - Trends Anal Chem. 2014, 53, 49–59. DOI: 10.1016/j.trac.2013.07.009.
- Yamashita, Y.; Miyahara, R.; Sakamoto, K. Emulsion and Emulsification Technology. Cosmetic Science and Technology: Theoretical Principles and Applications. In Cosmetic Science and Technology: Theoretical Principles and Applications; Elsevier Inc, 2017; pp 489–506. https://doi.org/10.1016/B978-0-12-802005-0.00028-8.
- Yildirim, M.; Sumnu, G.; Sahin, S. Rheology, Particle-Size Distribution, and Stability of Low-Fat Mayonnaise Produced via Double Emulsions. Food Science and Biotechnology. 2016, 25(6), 1613–1618. DOI: 10.1007/s10068-016-0248-7.
- Bera, B.; Khazal, R.; Karin, S. Coalescence Dynamics in Oil ‑ in ‑ Water Emulsions at Elevated Temperatures. Sci. Rep. 2021, 0123456789, 1–10. DOI: 10.1038/s41598-021-89919-5.
- Jiang, Y.; Zhang, C.; Yuan, J.; Yayun, W.; Feng, L.; Dapeng, L.; Huang, Q. E Ff Ects of Pectin Polydispersity on Zein/Pectin Composite Nanoparticles (Zaps) as High Internal-Phase Pickering Emulsion Stabilizers. Carbohydr. Polym. 2019, 219(February), 77–86. DOI: 10.1016/j.carbpol.2019.05.025.
- Xie, J.; Luo, Y.; Chen, Y.; Liu, Y.; Yueqin, M.; Zheng, Q.; Yue, P. Redispersible Pickering Emulsion Powder Stabilized by Nanocrystalline Cellulose Combining with Cellulosic Derivatives. Carbohydr. Polym. 2019, November 2018, 213, 128–137. DOI: 10.1016/j.carbpol.2019.02.064.
- Zhu, F. Starch Based Pickering Emulsions: Fabrication, Properties, and Applications. Trends Food Sci. Technol. 2019. DOI: 10.1016/j.tifs.2019.01.012.
- Schmidts, T.; Dobler, D.; Nissing, C.; Runkel, F. Journal of Colloid and Interface Science Influence of Hydrophilic Surfactants on the Properties of Multiple W/O/W Emulsions. J. Colloid Interface Sci. 2009, 338(1), 184–192. DOI: 10.1016/j.jcis.2009.06.033.
- Dickinson, E. Woodhead Publishing Limited. Hydrocolloids and emulsion stability. In Handbook of hydrocolloids, 2010. DOI: 10.1533/9781845695873.23.
- Blijdenstein, T. B. J.; Van Winden, A. J. M.; Van Vliet, T.; Van Der Linden, E.; Van Aken, G. A. “Serum Separation and Structure of Depletion- and Bridging-Flocculated Emulsions. Comp. 2004, 245, 41–48. DOI: 10.1016/j.colsurfa.2004.07.002.
- Schuch, A.; Helfenritter, C.; Funck, M.; Schuchmann, H. P. Emulsions. Colloids Surf. A. 2014. DOI: 10.1016/j.colsurfa.2014.06.012.
- Zhang, Z.; Mcclements, D. J. Overview of Nanoemulsion Properties: Stability, Rheology, and Appearance. In Nanoemulsions; Academic Press, 2018; pp 21–49. DOI: 10.1016/B978-0-12-811838-2.00002-3.
- Ghosh, S.; Rousseau, D. Emulsion Breakdown in Foods and Beverages. In Chemical Deterioration and Physical Instability of Food and Beverages; Woodhead Publishing Limited, 2010; pp 260–295. DOI: 10.1533/9781845699260.2.260.
- Wu, Y. S. Immiscible Displacement of Non-Newtonian Fluids. In Multiphase Fluid Flow in Porous and Fractured Reservoirs; Elsevier Inc, 2016; pp 127–166. DOI: 10.1016/B978-0-12-803848-2.00007-6.
- Huo, W.; Zhang, X.; Gan, K.; Chen, Y.; Jie, X.; Yang, J. Journal of the European Ceramic Society E Ff Ect of Zeta Potential on Properties of Foamed Colloidal Suspension. J. Eur. Ceram. Soc. 2019, 39(2–3), 574–583. DOI: 10.1016/j.jeurceramsoc.2018.08.035.
- Esfanjani, A. F.; Jafari, S. M.; Assadpour, E. Preparation of a Multiple Emulsion Based on Pectin-Whey Protein Complex for Encapsulation of Saffron Extract Nanodroplets. Food Chem. 2017, 221, 1962–1969. DOI: 10.1016/j.foodchem.2016.11.149.
- Tan, K. B.; Abdullah, A. Z.; Horri, B. A.; Salamatinia, B. Adsorption Mechanism of Microcrystalline Cellulose as Green Adsorbent for the Removal of Cationic Methylene Blue Dye. J. Chem. Soc. Pak. 2016, 38(4), 651–664.
- Naveed, A.; Mahmood, A.; Khan Haji, M.; Shoaib, A. J.; Gulfishan, M. A.; Muhammad, U. Formulation and Characterization of a Multiple Emulsion 1% L-ASCORBIC Acid. Bull. Chem. Soc. Ethiop. 2010, 24(1), 1–10.
- Katouzian, I.; Mahdi, S. Trends in Food Science & Technology Nano-Encapsulation as a Promising Approach for Targeted Delivery and Controlled Release of Vitamins. Trends Food Sci. Technol. 2016, 53, 34–48. DOI: 10.1016/j.tifs.2016.05.002.
- Mcclements, D. J. Design of Nano-Laminated Coatings to Control Bioavailability of Lipophilic Food Components. Journal of Food Science. 2010, 75(1), 30–42. DOI: 10.1111/j.1750-3841.2009.01452.x.
- Laouini, A.; Fessi, H.; Charcosset, C. Membrane Emulsification: A Promising Alternative for Vitamin E Encapsulation within Nano-Emulsion. J. Membr. Sci. 2012, 423–424, 85–96. DOI: 10.1016/j.memsci.2012.07.031.
- Marto, J.; Ascenso, A.; Simoes, S.; Almeida, A.; Ribeiro, M. Pickering Emulsions: Challenges and Opportunities in Topical Delivery. Expert Opinion on Drug Delivery. 2016. DOI: 10.1080/17425247.2016.1182489.
- Nsor-atindana, J.; Chen, M.; Douglas Goff, H.; Zhong, F.; Sharif, H. R.; Li, Y. Functionality and Nutritional Aspects of Microcrystalline Cellulose in Food. Carbohydr. Polym. 2017, 172, 159–174. DOI: 10.1016/j.carbpol.2017.04.021.