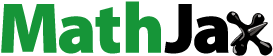
ABSTRACT
Cordyceps militaris (CM) is a critical fungus for promoting health, while the cordycepin has been recognized as an indicator compound with bioactive functions such as induction of apoptosis, anti-inflammation, inhibition of angiogenesis, and antioxidant properties. However, the cultivation of CM required appropriate light to induce the fruiting body into regular growth. Light-emitting diodes (LED), which combine different wavelengths and conserve energy, offer promising potential for applications in CM cultivation. Therefore, this study involved using low-power and low-illumination LED light sources of different wavelengths for intervention in the photoperiod of CM cultures to observe the effects on the growth pattern, bioactive substance content, and antioxidant activity. This study showed that the growth patterns of CM developed normally when irradiated with low-brightness LED light sources of different wavelengths. Moreover, the total phenolic content (TPC), cordycepin, and relative antioxidant activity levels in CM fruiting body water extract were significantly elevated upon exposure irradiated with a glare blue light wavelength range compared to other groups (p < .05). Hence, this study proved a high potential for LED irradiation to be applicable in CM industrial development and exploration of practical knowledge in industrial biotechnology with facilitated CM products that can be economically produced.
Introduction
Cordyceps militaris (CM; also called Yong Chong Cao) has been cultivated and developed artificially for various functional foods (no additional safety risks exist), particularly in Asian countries [Citation2–7] The bioactive components of CM are primarily Cordyceps polysaccharides, D-mannitol, carotenoid, adenosine, superoxide dismutase (SOD), guanosine, pentostatin, ergosterol, γ-aminobutyric acid (GABA), β-glucan and cordycepin, which are effective as antioxidants, enhancing performance during aerobic exercise, liver protection, radioprotection, immunomodulators, therapy of asthma, relief of fatigue, improvement of sex dysfunction, anti-depressants, anti-virus, anti-tumor agents, anti-diabetic, anti-hyperlipidemic, anti-atherosclerotic and eye care agents.[Citation1,Citation8,Citation9] The booming industry is in the Chinese market, where the CM mycelium powder and capsules (dosages from 100 to 1,000 mg) have been licensed as health food, which can alleviate coughs and asthma, reduce phlegm, nourish the lungs, and rehabilitate the kidneys.[Citation2,Citation8] Unfortunately, the industrialization of CM is accompanied by many issues, such as the life cycle of CM, the instability of the propagated strains (such as the natural decline), and the optimization of cultivation conditions in line with costs, which hinder the application of CM on an industrial scale.[Citation10] Therefore, there is a need to enhance CM proliferation, regulate and control bioactive component yield, and automate related processes, which have become the current focus of availability and sustainability in the industries.[Citation11]
Recent advancements in photovoltaic technology have significantly improved the brightness and efficiency of light-emitting diodes (LEDs). Studies indicate that these artificial lights have been extensively used in indoor cultivation under controlled settings, increasing agricultural productivity. [Citation12–14] Moreover, due to the low radiation, low-energy consumption, broad spectral matching, low-energy efficiency, long lifetime, and flexibility of use, these LEDs have a great potential to be used in various applications [Citation12–15] Light plays a crucial role in the environment, and for plants that require light to grow, red light is crucial for photosynthesis and can enhance starch accumulation in various species.[Citation12,Citation16] Conversely, blue light is vital for developing chloroplasts, forming chlorophyll, and opening stomata.[Citation12,Citation16] According to reports, the irradiation of mushroom mycorrhizal species has the potential to influence the biosignature of mycelium, affecting both growth rate and color appearance, as well as the morphology of fruiting bodies. At the same time, the process modulates the metabolic pathways and metabolite accumulation in edible mushrooms.[Citation12,Citation17] In addition, fungi acclimate to environmental conditions by using photoreceptors and signaling cascades to sense near-ultraviolet, blue, green, red, and far-red light to modulate gene expression involved in the development, stress response, secondary metabolite synthesis, and the biological clock [Citation18–20] In particular, Fungi possess blue light photoreceptors that serve as transcriptional regulators in the nucleus, which sense red and far-red light, and initiate signaling pathways that transmit signals from the cytoplasm to the nucleus.[Citation19] Moreover, the green light can be perceived through retinal binding proteins (known as opsins), which differ in plants.[Citation19]
The benefits of using LED lights in crop cultivation are numerous, and LED lights are the leading research focus in modern agriculture techniques. However, current plant factories and lights that use only red and blue LEDs do not provide optimal conditions for CM cultivation. Therefore, the purpose of this study was to design various wavelengths of LEDs to irradiate the CM fruiting bodies during cultivation and investigate the effects of different wavelengths and colors on the fruiting bodies, which included changes in the fruiting body weight, protein, TPC, total carbohydrate and cordycepin contents, respectively, while evaluating the antioxidant ability of the CM fruiting bodies. Thus, it can establish the quality control indexes for various LED light sources, which serve as the reference for developing CM products and improving quality.
Materials and methods
Materials
CM strains were gifts from the Beneficial Microbe Museum (Yilan, Taiwan). All chemicals were purchased from Sigma-Aldrich® (Merck KGaA, Darmstadt, Germany) and used as were unless specified otherwise. Brown rice was purchased from Union Rice Enterprise Co. (Changhua, Taiwan).
Designing the cultivation tanks, light-emitting diode (LED) wavelength, and incubation conditions
The CM cultivation tanks for this study were modified using refrigerated cabinets (TD0460, DAYTIME Co., Ltd., Pingtung, Taiwan) with humidity, lighting, and ventilation control components except for temperature control. In addition, the internal space was divided evenly by an opaque plastic partition. Each lay was designed as a 30 cm high area for fluorescent- (positive control), plant- (negative control), warm white-, red-, glare blue-, blue-, and cyan-light. LED lights source (). The AC rectifier controlled by the actuator converts AC to DC and stabilizes the voltage to provide a stable current that produces the LED’s luminance and color.
Figure 1. This study designed the culture tanks of Cordyceps militaris (CM) with various light-emitting diode (LED) compartments and settings.
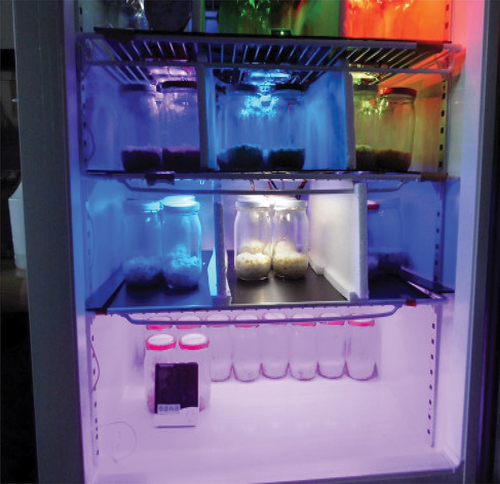
All the light sources were uniformly illuminated by a light source located 18 cm overhead. Moreover, the various irradiated light sources used in this study were determined at the top of the culture jar using a handheld LED meter (Rainbow Light Technology Co., Ltd., Taoyuan, Taiwan) to identify the wavelength, color temperature, and chromaticity coordinate characteristics. The illuminance (Lux) at the beginning and end of the study was measured using an illuminance meter (TES Electrical Electronic Co., Taiwan, Taipei), while the percentage difference between the pre- and post-illuminance was used to calculate the depreciation of the light.
In this study, cooked brown rice was used as the solid fermentation medium in glass jars, which was inoculated with 1% CM mycelium and then cultivated in the above tank under 18°C, 60–70% relative humidity, without light at the initial stage. All CM were exposed to 12 h of continuous light daily from the beginning of the protoplast differentiation stage until the formation of fruiting bodies.[Citation14] Simultaneously, the culture conditions were altered to 20°C, 80–90% relative humidity, and 14 h of continuous light exposure daily. Eventually, the cultivated CM fruiting body weight was measured.
Water extract CM fruiting bodies
Water extraction from CM fruiting bodies was performed as Wu et al.[Citation6] described with minor modifications. All samples were freeze-dried and ground into powder (passed through the 100-mesh sieve), then 0.5 g of each sample was added with 10 mL of deionized water (20 folds of weight by volume, w/v), and the extraction was assisted by 120 W ultrasound at 35 kHz for 20 min at room temperature (25°C). Afterward, centrifugation was performed at 1,200 × g for 20 min, followed by the supernatant being collected, freeze-dried, and stored at -20°C until used. In addition, the extraction yields of various CM samples incubated with different wavelengths of LED light source were calculated, while the total solid contents were determined.
Determination of CM compositions
Protein contents
The protein content was determined based on the dye-binding method, as described by Bradford.[Citation21] Each sample was taken 10 μL into a 96-well dish, added 200 μL of Bio-Rad protein analyzing dye (Bio-Rad Laboratories, Inc., Hercules, CA, USA) (deionized water diluted 5-fold), and allowed to react for 5 min, followed by measurement of absorbance at a wavelength of 595 nm using a micro-spectrometer (SPECTRO star Nano, BMG LABTECH GmbH, Ortenberg, Germany). The standard curve was prepared with different concentrations of bovine serum albumin (BSA), and the samples’ protein content was calculated using the regression equation obtained.
Total phenolic content (TPC)
The TPC of the sample was performed as described in Li et al.[Citation22] with minor modifications. Briefly, the sample 100 μL was mixed with 100 μL of 60% methanol (acidified with 0.3% HCl), and then 100 μL of the sample was mixed with 2 mL of 2% Na2CO3 solution, and the reaction was allowed for 2 min at room temperature (25°C). Next, 100 μL of 50% Folin-Ciocalteu reagent was added to mixing evenly and then stood at room temperature (25°C) for 30 min. Then, the absorbance of the reaction solution was determined at 750 nm using a micro-spectrometer (BMG LABTECH GmbH). In addition, the TPC in the sample was calculated by obtaining a regression equation from standard curves prepared with gallic acid (GA) methanol solutions at different concentrations and expressed as GA equivalents (GAE μg/mL).
Total carbohydrate content
The total carbohydrate content of the sample was performed as described in Nielsen,[Citation23] with minor modifications. The sample was 200 μL, and 200 μL of 5% phenol solution were mixed uniformly; then, 1 mL of sulfuric acid was added, followed by standing at room temperature (25°C) for 10 min. Next, vortex-induced oscillation remixed the reaction, allowing it to stand for 30 min. Finally, the absorbance at a wavelength of 490 nm was measured using a micro-spectrometer (BMG LABTECH GmbH). The standard curve was prepared with various concentrations of glucose solutions, and the obtained regression equation was used to calculate the total carbohydrate content of the sample.
Cordycepin contents
The bioactive substance cordycepin content in the sample was measured based on an approach described by Liu et al.[Citation24] with minor modifications. In this study, a high-performance liquid chromatography (HPLC) system (L-2130, Hitachi, Ltd., Tokyo, Japan) was used and equipped with a reverse chromatography column (Reprosil-Pur 120 C18-AQ, 5 μm, 250 × 4.6 mm, Dr. Maisch Beim Brü ckle, Germany). The analysis conditions were as follows: all samples were filtered by 0.45 μm microfiltration membrane, and the injection volume was 10 μL. The mobile phase was a mixture of phosphoric acid buffer solution (PBS; pH 6.5) and methanol in the ratio of 23:2 at a flow rate of 1 mL/min, and the operation was performed at room temperature (25°C) to detect absorbance signal at a wavelength of 260 nm. In addition, the cordycepin standard was used to prepare standard curves in different concentrations, while the cordycepin content in each sample was calculated by interpolation. The fingerprint profiles of cordycepin contents were established by culturing CM at different light source wavelengths, as shown in the information above.
Determination of antioxidant capacity
2,2-diphenyl-1-picrylhydrazyl (DPPH) free radical scavenging ability: The DPPH radical scavenging ability of the samples in this study was followed as described in Wang et al.[Citation25] with some modifications. The sample (50 mg/mL) was taken 50 μL mixed with 3 mL of 0.1 mM of DPPH methanol solution. It was incubated for 30 min at room temperature (25°C) in a dark room, and then the absorbance of the sample solution at 517 nm was measured with a micro-spectrometer (BMG LABTECH GmbH). The DPPH radical scavenging capacity was calculated as follows.
Reducing power
The reducing power of this study sample was measured based on the method described by Huang et al.[Citation26] with minor modifications. All sample solutions of 0.2–1.0 mg/mL (500 μL) were mixed with 500 μL of 200 mM PBS (pH 6.6) and 2.5 mL of 1% potassium ferricyanide. Next, the samples were incubated at 50°C for 20 min, then 0.5 mL of 10% trichloroacetic acid was added, followed by centrifugation at 937 ×g for 10 min. The upper clarified solution (1 mL) was mixed with 1 mL of deionized water and 1 mL of 0.1% ferric chloride, which was allowed to stand for 10 min; ultimately, the absorbance at 700 nm was measured by the micro-spectrometer (BMG LABTECH GmbH). The reducing power was expressed as the absorbance value, which was higher to indicate that the sample was more reducing.
Statistical analysis
This study’s data was presented as mean ± standard deviation (SD) with triple replicates for all trials. All data were analyzed using one-way analysis of variance (ANOVA) analysis of differences between groups, and statistical analyses were performed using SPSS software (V26, IBM Co., Armonk, New York, USA). Duncan’s multiple range test analyzed the differences among the groups, and p < .05 was regarded as a significant difference.
Results and discussion
Performance of different LED light sources on CM fruiting bodies growth
This study measured the properties and color coordinates of each light source and showed that fluorescent light contains various wavelengths of light, while plant light was composed of two wavelengths, red (665 nm) and blue (450 nm), respectively, with a higher proportion of the blue (). The warm-white light source consists of two wavelengths of blue- (445 nm) and yellow-light (581 nm), where yellow light accounts for a higher proportion. In contrast, the red light source has a wavelength of 632 nm, the glare blue light source of 461 nm, and the blue light source has a single wavelength of 464 nm, but the luminous intensity varies. The cyan light source was composed of blue light with a wavelength of 447 nm and green ones with a wavelength of 515 nm. Notably, the blue light accounted for a higher proportion of the total light emitted by the source. Regarding the color coordinates of each light source, except for the fluorescent, plant, and glare blue light sources, the illumination exceeds 1,000 Lux, while the others were less than 700 Lux ( and ). As per the reports by Chiang et al.[Citation27] and Feng et al.[Citation20] the adenosine content did not change even with varying light conditions. However, upon being subjected to 1,000 Lux of red light, CM fruiting bodies showed a notable increase, contradicting this study’s results. Yet, it confirmed that exposure to red light adversely affects the accumulation of cordycepin content,[Citation20] which was agreed with this study. However, as stated in the report by Wu et al.[Citation28] it has been found that the implementation of varying light cycles with different intensities of 1,250 ± 250 and 1,750 ± 250 Lux can significantly promote the differentiation of CM fruiting bodies. It has also been observed to increase the contents of bioactive components, namely mannitol and polysaccharides.[Citation27] In terms of the power consumption (Watt; W) of each light source, the fluorescent light was the highest (20.0 W), followed by the plant light (10.2 W), while other LED light sources consumed only 2.3 W (). In depreciation rate, from the beginning to the end of cultivation, the light depreciation of fluorescent light was 10%, while the cyan light source was as high as 43.6%, and the other LED light sources were at most 3%.
Figure 2. The full-wavelength spectra and the coordinates of the light colors of the light sources illuminated by the fluorescent light and light-emitting diodes (LEDs), while the wavelengths of each light source with the highest intensity in the full-wavelength spectrum are shown as 435 nm for fluorescent light (a), 665 nm for plant light (b), 581 nm for warm white light (c), 632 nm for red light (d), 461 nm for glare blue light (e), 464 nm for blue light (f), and 447 nm for cyan light (g).
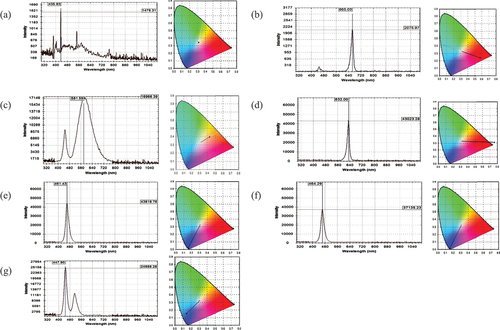
Table 1. Different irradiation light sources of the characteristics of analysis and the depreciation of the light.
During irradiation with various light sources, the observation of CM cultivation went through different stages, including mycelium formation, prototrophy, mycelium bud development, and mycelium maturation into fruiting bodies. At the same time, it was revealed that the cultivation process was consistent with the cultivation of normal ones. Therefore, this also implied that the appearance of CM fruiting bodies was not significantly affected by the different light sources used. Despite the simultaneous treatment of all groups during cultivation, there were significant differences (p < .05) () in CM fruiting body weights under different light irradiation. The weights in descending order were glare blue-, fluorescent-, plant-, blue-, cyan-, red-, and warm white- light. This study finding suggested that using specific wavelengths of light could be an effective approach for improving the yield of CM fruiting bodies (). In contrast, there was an insignificant effect on insoluble solids content except for the red light group (). Yi et al.[Citation14] reported that blue LEDs are necessary to induce the CM primordial and increase the carotenoid levels in their fruiting bodies. Moreover, Dong et al.[Citation29] reported different wavelengths of light, namely pink light (1/3, 450–460 nm with 2/3, 620–630 nm), red light (620–630 nm), and blue light (450–460 nm), were found to promote the weight increase in CM fruiting bodies significantly. It was hypothesized that the differences in the wavelength ranges used in the published results and this study were responsible for the slight differences. Therefore, it is worth noting that further research is needed to investigate the underlying mechanisms and to optimize the light conditions for the cultivation of CM fruiting bodies.
Table 2. Effect of different irradiation light sources on Cordyceps militaris (CM) fruiting bodies, and insoluble solids content and extraction yield of CM fruiting bodies water extracts.
Performance of different LED light sources on the bioactive components of CM fruiting bodies
CM has been proven to have multiple biological activities, such as cordycepin, carbohydrates, proteins, TPC and its derivatives, etc.[Citation24,Citation30,Citation31] In particular, cordycepin is considered an essential active indicator ingredient, and it is generally used as a vital quality control indicator for CM fruiting bodies in the production process of related companies. This study examined the protein, TPC, total carbohydrate, and cordycepin contents of water extracts of CM fruiting bodies. It was found that the highest protein content was present in the glare blue LED treatment group, and there was a significant difference (p < .05) compared to all other groups (). However, no significant differences were found between the other treatment groups, except for the fluorescent light treatment group. Regarding TPC, the order from highest to lowest showed glare blue-, cyan-, blue-, fluorescent-, warm white-, plant-, and red-light (p < .05). Conversely, regarding total carbohydrate contents, the red-light source was significantly higher than all groups (p < .05) (). These phenomena were attributed to the effects of different color light sources and wavelengths on protein, TPC, and total carbohydrate contents in CM fruiting bodies, namely, the beneficial effects on the metabolic mechanisms of CM.[Citation16] In particular, glare blue light enhances the accumulation of proteins and TPC in CM fruiting bodies,[Citation14,Citation17] whereas red light demonstrated the opposite trend.[Citation14]
Table 3. Performance of different LED light sources on the bioactive components and antioxidant ability of Cordyceps militaris (CM) fruiting bodies water extracts.
The HPLC analysis showed that cordycepin content was highest in cyan and glare blue light, followed by plant light, whereby compared to the others, there was a significant difference (p < .05). Notably, red light exhibited the lowest cordycepin content due to the reasons mentioned earlier and limitations. There have been reports that CM failed to produce cordycepin under typical cultivation conditions.[Citation30] However, in published studies that have reported an increased cordycepin content with red, green, and far-infrared LED light irradiation,[Citation14,Citation27] it was hypothesized that the possible difference is because the general research used a wide range of wavelengths. In contrast, this study used a single wavelength, and another possibility was that it resulted from the slight difference in the culture conditions and the CM species. Moreover, Dong et al.[Citation29] reported that pink-, blue-, fluorescent-, and red-light all promoted bioactive substance contents in CM, whereas pink-light showed the most significant accumulation of carotenoids and cordycepin. Red light yielded the highest adenosine content, suggesting that light wavelengths and LED lights combination could be adjusted according to the demand for the targeted compounds to be the cultured duration and stage.[Citation27] Notably, Huang et al.[Citation32] reported the expression of lysosomal ubiquitinated proteins in CM mycelium by blue light, which facilitates the development of the fruiting body. It has been documented that exposure to blue light impedes the elongation and expansion of CM cells while concurrently promoting the biosynthesis of cordycepin.[Citation29] The same authors postulated that this mechanism was associated with cordycepin’s capacity to impede DNA replication, explicitly functioning as an anti-mutagenic protective agent under short-wavelength light, resulting in elevated cordycepin yield.[Citation29] In addition to the aforementioned, adenosine and cordycepin have been reported to exhibit preventative properties against cellular reactive oxygen species damage, anti-inflammatory effects, broad-spectrum antibiotic activity, cancer cell proliferation inhibition, apoptotic induction, and antioxidant activity in CM.[Citation33]
Effect of various LED light sources on the antioxidant ability of CM fruiting bodies water extracts
Antioxidant activity of various natural biological compounds has been evaluated as one of several ways to prevent or promote disease and related adverse effects.[Citation25,Citation26] In this study, the DPPH radical scavenging ability of CM fruiting bodies water extracts by different irradiation light sources showed that the scavenging rate of 82.33 ± 1.80% in the glare blue light group exhibited a satisfactory performance (), and compared to the other groups, there was a significant difference (p < .05). In contrast, the red light group could expect the lowest scavenging rate (57.30 ± 2.50%). These phenomena can be attributed to bioactive component contents (TPC and cordycepin) as described above. Huang et al.[Citation34] who reported a cordycepin improvement in diabetes-induced testicular damage, revealed that the regulation of downstream antioxidant enzyme activity via the SIRT1/Foxo3a signaling pathway involved the expression of both Mn-SOD and CAT. In addition, Li et al.[Citation35] also reported that CM could protect mitochondria by scavenging reactive oxygen species, inhibiting mitochondrial swelling, and increasing antioxidant enzyme activities. The findings of an animal model study suggest that CM has a significant impact on the activities of peroxidase, SOD, GPx, and anti-hydroxyl radicals in the liver of mice.[Citation36] However, this implied that CM fruiting bodies could accumulate more metabolites related to antioxidant activity under the current glare blue light irradiation conditions, contributing to better antioxidant potential. According to published studies, these substances have been identified as polysaccharides, β-glucans, carotenoids, TPC, and flavonoids.[Citation10,Citation22]
The results of reducing power in this study showed a significant dose-dependence effect in all groups (). At concentrations of 0.2 and 1.0 mg/mL, it was observed that the glare blue light demonstrated superior reducing power at the lower concentration in comparison to the other groups, which exhibited significant difference (p < .05). Moreover, at the maximum concentration, the reducing power, in descending order, were cyan blue, blue, glare blue, warm white lights, fluorescent light, red light, and plant light, respectively, which were significantly different from each other (p < .05). Overall, this study indicated that different LED lights were the most beneficial and modifiable in enhancing the bioactive content of CM fruiting bodies cultured on brown rice substrates, while this was similar to the previous results.[Citation20,Citation27,Citation29]
Conclusion
This study showed that irradiating a glare blue LED light source was beneficial to the cultivation of CM fruiting bodies, which had a satisfactory content of bioactive compounds and favorable antioxidant properties. This will substantially contribute to industrial development with a high potential for application. However, this study was subject to limitations insofar as it solely investigated the effects of optimal light conditions, without being coordinated with the medium formulation, on the cultivation of CM fruiting bodies. In pursuing high-quality CM fruiting bodies, scientists are actively researching the optimal combination of LED light assistance and other cultivation factors. It’s about figuring out the ideal conditions regarding temperature, carbon and nitrogen sources in the culture media, nutrient availability, agitation, and other relevant parameters that will support the growth and development of CM fruiting bodies to their full potential and will undoubtedly have important implications for the broader CM cultivation. Subsequently, it is imperative to conduct a comprehensive investigation to clarify potential mechanisms that may impact the metabolism of CM bioactive compounds. It was highly recommended that future research endeavors focus on identifying the antioxidant mechanisms and bioactive components involved in this process, which could prove invaluable. Hence, this study’s findings represent a milestone in accumulating glare blue LED light source-assisted CM fruiting bodies’ cultivation and bioactive compounds.
CRediT authorship contribution statement
Yu-Wen Lin: Conceptualization, Validation, Formal analysis, Supervision, Project administration, Funding acquisition, Resources, Writing – original draft, review & editing. Te-Ming Lee: Conceptualization, Formal analysis, Methodology, Data curation, Validation. Cheng-Pei Chung: Investigation, Project administration, Resources. Yi-Chieh Tsai: Investigation, Validation, Project administration. Da-Wei Huang: Methodology, Visualization, Validation. Ping-Hsiu Huang: Software, Visualization, Writing – original draft, writing – review & editing. All authors contributed to the article and approved the submitted version.
Acknowledgments
All authors appreciate SinYen Technology Co., Ltd. for the financial support of this study.
Disclosure statement
No potential conflict of interest was reported by the author(s).
Data availability statement
The data that has been used is confidential.
Additional information
Funding
References
- Chen, B.-Y.; Huang, H.-S.; Tsai, K.-J.; Wu, J.-L.; Chang, Y.-T.; Chang, M.-C.; Lu, C.-M.; Yang, S.-L.; Huang, H.-S. Protective Effect of a Water-Soluble Carotenoid-Rich Extract of Cordyceps Militaris Against Light-Evoked Functional Vision Deterioration in Mice. Nutrients 2022, 14(8), 1675. DOI: 10.3390/nu14081675.
- Nxumalo, W.; Elateeq, A. A.; Sun, Y. Can Cordyceps Cicadae Be Used as an Alternative to Cordyceps Militaris and Cordyceps sinensis? – a Review. J. Ethnopharmacol. 2020, 257, 112879. DOI: 10.1016/j.jep.2020.112879.
- Duan, X.; Yang, H.; Wang, C.; Liu, H.; Lu, X.; Tian, Y. Microbial Synthesis of Cordycepin, Current Systems and Future Perspectives. Trends Food Sci. Technol. 2023, 132, 162–170. DOI: 10.1016/j.tifs.2023.01.006.
- Phull, A.-R.; Ahmed, M.; Park, H.-J. Cordyceps Militaris As a Bio Functional Food Source: Pharmacological Potential, Anti-Inflammatory Actions and Related Molecular Mechanisms. Microorganisms. 2022, 10(2), 405. DOI: 10.3390/microorganisms10020405.
- Liu, W.; Dun, M.; Liu, X.; Zhang, G.; Ling, J. Effects on Total Phenolic and Flavonoid Content, Antioxidant Properties, and Angiotensin I-Converting Enzyme Inhibitory Activity of Beans by Solid-State Fermentation with Cordyceps Militaris. Int. J. Food Prop. 2022, 25(1), 477–491. DOI: 10.1080/10942912.2022.2048009.
- Wu, C.-Y.; Liang, C.-H.; Liang, Z.-C. Enhanced Production of Fruiting Bodies and Bioactive Compounds of Cordyceps Militaris with Grain Substrates and Cultivation Patterns. J. Taiwan Inst. Chem. Eng. 2022, 132, 104138. DOI: 10.1016/j.jtice.2021.11.005.
- Long, L.; Liu, Z.; Wang, Y.; Lin, Q.; Ding, S.; Li, C.; Deng, C. High-Level Production of Cordycepin by the Xylose-Utilising Cordyceps Militaris Strain 147 in an Optimised Medium. Bioresour. Technol. 2023, 388, 129742. DOI: 10.1016/j.biortech.2023.129742.
- Raethong, N.; Thananusak, R.; Cheawchanlertfa, P.; Prabhakaran, P.; Rattanaporn, K.; Laoteng, K.; Koffas, M.; Vongsangnak, W. Functional Genomics and Systems Biology of Cordyceps Species for Biotechnological Applications. Curr. Opin. Biotechnol. 2023, 81, 102939. DOI: 10.1016/j.copbio.2023.102939.
- Cao, C.; Yang, S.; Zhou, Z. The Potential Application of Cordyceps in Metabolic-Related Disorders. Phytotherapy Res. 2020, 34(2), 295–305. DOI: 10.1002/ptr.6536.
- Lin, S.-P.; Sung, T.-H.; Angkawijaya, A. E.; Go, A. W.; Hsieh, C.-W.; Hsu, H.-Y.; Santoso, S. P.; Cheng, K.-C. Enhanced Exopolysaccharide Production of Cordyceps Militaris via Mycelial Cell Immobilization on Plastic Composite Support in Repeated-Batch Fermentation. Int. J. Biol. Macromol. 2023, 250, 126267. DOI: 10.1016/j.ijbiomac.2023.126267.
- Zhang, J.; Jian, T.; Zhang, Y.; Zhang, G.; Ling, J. Dynamic Content Changes of Cordycepin and Adenosine and Transcriptome in Cordyceps Kyushuensis Kob at Different Fermentation Stages. Bioprocess Biosyst. Eng. 2021, 44(8), 1793–1803. DOI: 10.1007/s00449-021-02561-3.
- Hamedalla, A. M.; Ali, M. M.; Ali, W. M.; Ahmed, M. A. A.; Kaseb, M. O.; Kalaji, H. M.; Gajc-Wolska, J.; Yousef, A. F. Increasing the Performance of Cucumber (Cucumis Sativus L.) Seedlings by LED Illumination. Sci. Rep. 2022, 12(1), 852. DOI: 10.1038/s41598-022-04859-y.
- Wu, M.-C.; Hou, C.-Y.; Jiang, C.-M.; Wang, Y.-T.; Wang, C.-Y.; Chen, H.-H.; Chang, H.-M. A Novel Approach of LED Light Radiation Improves the Antioxidant Activity of Pea Seedlings. Food Chem. 2007, 101(4), 1753–1758. DOI: 10.1016/j.foodchem.2006.02.010.
- Yi, Z.-L.; Huang, W.-F.; Ren, Y.; Onac, E.; Zhou, G.-F.; Peng, S.; Wang, X.-J.; Li, H.-H. LED Lights Increase Bioactive Substances at Low Energy Costs in Culturing Fruiting Bodies of Cordyceps Militaris. Sci. Hortic. 2014, 175, 139–143. DOI: 10.1016/j.scienta.2014.06.006.
- Schubert, E. F.; Kim, J. K. Solid-State Light Sources Getting Smart. Science. 2005, 308(5726), 1274–1278. DOI: 10.1126/science.1108712.
- Kho, C.-H.; Kan, S.-C.; Chang, C.-Y.; Cheng, H.-Y.; Lin, C.-C.; Chiou, P.-C.; Shieh, C.-J.; Liu, Y.-C. Analysis of Exopolysaccharide Production Patterns of Cordyceps Militaris Under Various Light-Emitting Diodes. Biochem. Eng. J. 2016, 112, 226–232. DOI: 10.1016/j.bej.2016.04.028.
- Feng, Y.; Xu, H.; Sun, Y.; Xia, R.; Hou, Z.; Li, Y.; Wang, Y.; Pan, S.; Li, L.; Zhao, C., et al. Effect of Light on Quality of Preharvest and Postharvest Edible Mushrooms and Its Action Mechanism: A Review. Trends Food Sci. Technol. 2023, 139, 104119. DOI: 10.1016/j.tifs.2023.104119.
- In-On, A.; Thananusak, R.; Ruengjitchatchawalya, M.; Vongsangnak, W.; Laomettachit, T. Construction of Light-Responsive Gene Regulatory Network for Growth, Development and Secondary Metabolite Production in Cordyceps Militaris. Biology. 2022, 11(1), 71. DOI: 10.3390/biology11010071.
- Yu, Z.; Fischer, R. Light Sensing and Responses in Fungi. Nat. Rev. Microbiol. 2019, 17(1), 25–36. DOI: 10.1038/s41579-018-0109-x.
- Feng, Y.-J.; Zhu, Y.; Li, Y.-M.; Li, J.; Sun, Y.-F.; Shen, H.-T.; Wang, A.-Y.; Lin, Z.-P.; Zhu, J.-B. Effect of Strain Separated Parts, Solid-State Substrates and Light Condition on Yield and Bioactive Compounds of Cordyceps Militaris Fruiting Bodies. CyTA - J. Food. 2018, 16(1), 916–922. DOI: 10.1080/19476337.2018.1498130.
- Bradford, M. M. A Rapid and Sensitive Method for the Quantitation of Microgram Quantities of Protein Utilizing the Principle of Protein-Dye Binding. Anal. Biochem. 1976, 72(1), 248–254. DOI: 10.1016/0003-2697(76)90527-3.
- Li, P.-H.; Shih, Y.-J.; Lu, W.-C.; Huang, P.-H.; Wang, C.-C. R. Antioxidant, Antibacterial, Anti-Inflammatory, and Anticancer Properties of Cinnamomum Kanehirae Hayata Leaves Extracts. Arabian J. Chem. 2023, 16(7), 104873. DOI: 10.1016/j.arabjc.2023.104873.
- Nielsen, S. Total Carbohydrate by Phenol-Sulfuric Acid Method. In Food Analysis Laboratory Manual, Nielsen, S. S., Ed.; Springer International Publishing: Cham, Schweiz, 2017; pp 137–141.
- Liu, J.; Liu, B.; Xue, Q.; Zhang, H.; Xue, Z.; Qian, K.; Zhang, J.; Jin, Y.; Han, J.; Zhu, C. Analysis of Appearance and Active Substances of Cordyceps Militaris Stromata on Antheraea Pernyi Pupae After Optimization. Food Sci. Technol. 2023, 43, 43. DOI: 10.1590/fst.127022.
- Wang, C.-Y.; Chen, Y.-W.; Hou, C.-Y. Antioxidant and Antibacterial Activity of Seven Predominant Terpenoids. Int. J. Food Prop. 2019, 22(1), 230–238. DOI: 10.1080/10942912.2019.1582541.
- Huang, P.-H.; Lu, H.-T.; Wang, Y.-T.; Wu, M.-C. Antioxidant Activity and Emulsion-Stabilizing Effect of Pectic Enzyme Treated Pectin in Soy Protein Isolate-Stabilized Oil/Water Emulsion. J. Agric. Food Chem. 2011, 59(17), 9623–9628. DOI: 10.1021/jf202020t.
- Chiang, S.-S.; Liang, Z.-C.; Wang, Y.-C.; Liang, C.-H. Effect of Light-Emitting Diodes on the Production of Cordycepin, Mannitol and Adenosine in Solid-State Fermented Rice by Cordyceps Militaris. J. Food Compost. Anal. 2017, 60, 51–56. DOI: 10.1016/j.jfca.2017.03.007.
- Wu, C. Y.; Liang, Z. C.; Tseng, C. Y.; Hu, S. H. Effects of Illumination Pattern During Cultivation of Fruiting Body and Bioactive Compound Production by the Caterpillar Medicinal Mushroom, Cordyceps Militaris (Ascomycetes). Int. J. Med. Mushrooms. 2016, 18(7), 589–597. DOI: 10.1615/intjmedmushrooms.v18.i7.40.
- Dong, J. Z.; Lei, C.; Zheng, X. J.; Ai, X. R.; Wang, Y.; Wang, Q. Light Wavelengths Regulate Growth and Active Components of Cordyceps Militaris Fruit Bodies. J. Food Biochem. 2013, 37(5), 578–584. DOI: 10.1111/jfbc.12009.
- Wang, J.; Kan, L.; Nie, S.; Chen, H.; Cui, S. W.; Phillips, A. O.; Phillips, G. O.; Li, Y.; Xie, M. A Comparison of Chemical Composition, Bioactive Components and Antioxidant Activity of Natural and Cultured Cordyceps Sinensis. LWT - Food Sci. Technol. 2015, 63(1), 2–7. DOI: 10.1016/j.lwt.2015.03.109.
- Seelarat, W.; Sangwanna, S.; Panklai, T.; Chaosuan, N.; Bootchanont, A.; Wattanawikkam, C.; Subcharoen, A.; Subcharoen, N.; Chanchula, N.; Boonyawan, D., et al. Enhanced Fruiting Body Production and Bioactive Phytochemicals from White Cordyceps Militaris by Blending Cordyceps Militaris and Using Cold Plasma Jet. Plasma Chem. Plasma Process. 2023, 43(1), 139–162. DOI: 10.1007/s11090-022-10292-w.
- Huang, Z.-Y.; Feng, L.; Fu, M.-J.; Zhang, D.-D. Differential Ubiquitome Analysis of Cordyceps Militaris Lysine-Ubiquitinated Proteins Affected by Blue Light. Biologia. 2022, 77(7), 1965–1979. DOI: 10.1007/s11756-022-01064-0.
- Eiamthaworn, K.; Kaewkod, T.; Bovonsombut, S.; Tragoolpua, Y. Efficacy of Cordyceps Militaris Extracts Against Some Skin Pathogenic Bacteria and Antioxidant Activity. J. Fungi (Basel) 2022, 8(4), 327. DOI: 10.3390/jof8040327.
- Huang, T.; Zhou, Y.; Lu, X.; Tang, C.; Ren, C.; Bao, X.; Deng, Z.; Cao, X.; Zou, J.; Zhang, Q., et al. Cordycepin, a Major Bioactive Component of Cordyceps militaris, Ameliorates Diabetes-Induced Testicular Damage Through the Sirt1/Foxo3a Pathway. Andrologia 2022, 54(1), e14294. DOI: 10.1111/and.14294.
- Li, X. T.; Li, H. C.; Li, C. B.; Dou, D. Q.; Gao, M. B. Protective Effects on Mitochondria and Anti-Aging Activity of Polysaccharides from Cultivated Fruiting Bodies of Cordyceps Militaris. Am. J. Chin. Med. 2010, 38(6), 1093–1106. DOI: 10.1142/s0192415x10008494.
- Gu, C.; Zhang, D.; Zhai, W.; Zhang, H.; Wang, S.; Lv, S.; Bao, Y.; Zhu, D.; Feng, S.; Guo, S., et al. Research Progress on Cordyceps Militaris Polysaccharides. Food Biosci. 2022, 45, 101503. DOI: 10.1016/j.fbio.2021.101503.