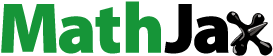
ABSTRACT
Bovine colostrum is the initial milk produced after birth that contains high levels of immunoglobulins and antimicrobial peptides. This study examined the production of bovine colostrum powder using two methods including spray- and freeze-drying. Spray-drying involves quickly drying a liquid or slurry with hot gas, while freeze-drying is a low-temperature dehydration process that preserves the product by freezing it and at reduced pressure. The aim was to compare the effectiveness of spray-drying versus freeze-drying in producing colostrum powder, focusing on protein and defensive protein content. The freeze-drying sample contained higher levels of protein and defensive proteins (IgG and IgA) compared to spray-drying. The DPPH value was high in freeze-dried samples, while higher values of FRAP, TPC, and ABTS were found in spray-dried samples. Microbiological analysis indicated freeze drying generally yielding lower counts. Sensory evaluation suggested that spray-dried colostrum scored higher for flavor and aroma, while freeze-dried samples were preferred in appearance. Overall, the study underscored the distinction between spray=drying and freeze-drying in producing bovine colostrum powder, with implications for nutritional content and sensory attributes.
Introduction
The initial milk that mammals generate after giving birth is called colostrum, considered to be the best diet and medicine to shield newborns from infections they may encounter throughout their lives. Colostrum, also known as beestings or bisnings,[Citation1] is the first milk produced during 3–4,[Citation2] 5–7 days postpartum[Citation3] and immune and growth factors are available in abundant amounts.[Citation4] A thick, gooey, yellowish liquid with a higher concentration of antibodies than regular milk.[Citation5] According to Godhia and Patel,[Citation6] there are many variables that can affect the composition and physical characteristics of colostrum, including the health of the animal,[Citation7] preterm feeding, calving frequency, and individual calving patterns. The most important colostrum is that collected in the first 24 to 48 h. According to Kehoe et al.[Citation8] Stelwagen et al.[Citation9] Kumar et al.[Citation10] immunoglobulin immunity is provided by IgG and IgA, which are abundant in colostrum. Proteins, lipids, lactose, vitamins, amino acids, essential fatty acids, hormones, growth factors, and cytokines can all be found in colostrum. Colostrum is a dietary supplement that strengthens the body’s defenses, promotes growth, and promotes development since it contains immune and growth-promoting substances. Immunoglobulin, which is found in high concentrations in colostrum and is crucial for the host’s defense against pathogenic organisms,[Citation11] is present in the colostrum. In colostrum, immunoglobulins, growth factors, lysozymes, lactoferrin, lactoperoxidase are regarded as significant bioactive components. Immunoglobulin G (IgG) is present in high concentrations in colostrum.
Spray-drying is a method that is frequently employed. This technique, which diminishes the water content, has a number of benefits, including a reduction of the rate of microbiological degradation of the spray-dried products, a minimization of lipid oxidation, and preferable retention of the original emulsion structure.[Citation12] To prevent heat damage to the bioactive components, this approach uses controlled evaporation to maintain low droplet temperatures that are lower than the outlet air temperatures in the adjacent system.[Citation13] Colostrum powder was produced using a glass spray dryer. Prior to spray-drying skim milk, some thermal processes are used, such as pasteurization and vacuum concentration, which frequently cause colostrum to gel due to denaturation of the protein in the colostrum. At feed temperature, colostrum was spray-dried while drying airflow rate was used to keep the inlet and output air temperatures constant. In order to contain moisture on a dry basis, spray-dried material was dried using a desiccator. When maintained in PET pouches and aluminum laminated polythene bags, colostrum showed a steady decline in the IgG activity.[Citation14]
Freeze-drying is a method in which chilling is combined with dehydration and ice crystal symbolization. Freeze-drying lowers water activity, preventing the formation of bacteria and slowing harmful enzymatic reactions. In addition to preserving the natural food product characteristics include taste, shade, form, biological anatomy, and, most significantly, nutritive content, freeze-drying is an effective method for maintaining all natural substances.[Citation15] Freeze-drying is the most preferred dehydration method for turning colostrum into colostrum powder since it involves a quick change from a hydrated to a dehydrated state of the item. This method helps to minimize damage to thermally vulnerable components, mainly IgG, while prolonging shelf life without changing its original properties.[Citation16] Heat-sensitive materials are best dehydrated by freeze-drying. The freeze-dryer system created the powder. The dried powder, which had a moisture level of less than 5% was gathered, packaged, and kept.[Citation17] The aim of this work was to investigate the drying performance and colostrum quality with two different drying methods including spray- and freeze-drying.
Materials and methods
Raw material and reagents
Cows’ colostrum was obtained from dairy farms in Faisalabad, Punjab, Pakistan. All cows were healthy and received a similar living arrangement with proper feed. The colostrum samples were taken from all the animals and transported to the laboratory at 4°C. This bovine colostrum (BC) was stored at −20°C for the first 3 days of collection and analyzed further. All of the chemicals and reagents were obtained from Sigma Aldrich (Tokyo, Japan).
Drying procedures
The stored frozen bovine colostrum (BC) was defrosted at room temperature and filtered using centrifugal separator SE 02.0 V (Seital S.r.l., Via delle Prese, Santorso (VI, Italy)). To homogenize the melted bovine colostrum (BC), centrifugation was carried out at 1000 revolutions per minute (rpm) for of 5 min. Total five treatments were prepared with different concentrations of BC emulsions for spray-drying and freeze-drying process as shown in .
Table 1. Treatment plan for bovine colostrum of spray and freeze dryer.
Spray- and freeze-drying conditions
The homogenized bovine colostrum (BC) emulsion powders were prepared using a laboratory spray dryer (TP-S15, Xi’an China) according to spray-drying described by Rahim et al.[Citation18] with some modifications. The spary dryer was inlet air temperature (IAT) of 125 ± 2°C and an outlet air temperature (OAT) of 185 ± 2°C. Other parameters were wall material (20%), pump speed (6), and needle speed (9 s). To ensure consistent drying kinetics, both the drying airflow rate and the pressurized airflow at the sprayer nozzle remained constant. The sample’s moisture content was below the permitted threshold of 5%.
Freeze-drying is the most favorable technique for the drying of heat-sensitive products. The entire batch of bovine colostrum (BC) emulsions on petri dishes were freezed at −20°C in refrigerator prior to freeze-drying process according to modified method by Zhang et al.[Citation19] Frozen forms of emulsions were lyophilized using freeze dryer (VaCo2, Zirbus, Germany) at 1.60 mBar vacuum for the complete removal of water. After drying of 18 h, the lyophilized powders (freeze-dried materials) were removed from the petri plates and softly crushed with a glass rod to create homogeneous powder. Following production, all powder samples from spray-drying and freeze-drying process were promptly stored in sterilized dry glass vials at 4°C till further use.
Chemical analysis of spray and freeze dryer colostrum powder
The water content, protein, fat, ash, total solid, and the ash percentage of spray- and freeze-dried colostrum powder were determined utilizing methods mentioned in AOAC 2006. The detection of lactose percentage was performed using refractometer (RI2031 plus, Jasco, Germany) according to method as described by Aburjaile et al.[Citation20] The IgG and IgA levels in the spray- and freeze-dried colostrum powder samples were assessed using the Bovine IgG ELISA pink-ONE Core Kit from KOMA BIOTECH, Seoul, South Korea, following the method provided by Borad et al. (14). The spray- and freeze-dried colostrum powder samples were diluted to contain the respective immunoglobulin within the range of 500–15.625 ng/mL using phosphate-buffered saline (pH 7.4). The ELISA was conducted at 25°C, and absorbance readings were taken at 450 nm using Multimode Plate Readers (TECAN Infinite M200 PRO, Switzerland).
Bovine colostrum Powder yield (%)
All the samples of colostrum Powder yield (%) of spray- and freeze-drying method were calculated using the following formula given by Lee et al.[Citation21]
Yield (%) = Mass of powder obtained (g)/Mass of the bovine colostrum (g) x 100%
In vitro evaluation of anti-oxidant content
Total phenolic contents (TPC)
The Folin–Ciocalteu method was used to determine the total phenolic contents (TPC) of extracts concentrated at 1 mg/mL.[Citation22] A Ultraviolet-Visible Spectrophotometer (Shimadzu UV-160A, Kyoto, Japan) was used to measure the development of the blue-colored complex between molybdenum and tungsten in the Folin-Ciocalteu reagent. After 2 h, absorbance was measured at 765 nm against control that has all reaction reagents except sample extract. Total polyphenols were estimated and the results were represented as gallic acid equivalent (mg gallic acid/100 g) of spray- and freeze-dried colostrum powder samples.[Citation23]
Free radical scavenging activity (DPPH assay)
DPPH (1, 1-diphenyl-1-picrylhydrazyl) is a stable and intensely colored oxidizing radical that produces a yellow hydrazine (DPPH-H) through the abstraction of free hydrogen atoms from phenolic antioxidants. The method outlined by Oussaief et al.[Citation24] was employed to assess the DPPH free radical scavenging activity of spray- and freeze-dried colostrum sample. A sample solution was prepared by .0 mL of colostrum sample and 1.0 mL of an ethanol solution of DPPH (125 mM) were combined. The absorbance was measured at 517 nm using a UV/visible light spectrophotometer (Shimadzu UV-160A, Kyoto, Japan). Similarly, a blank sample with the same solvent and DPPH solution but lacking spray- and freeze-dried colostrum samples was prepared, and its absorbance was measured at the same wavelength. The samples were prepared and measured three times for each. The free radical-scavenging activity of each spray- and freeze-dried colostrum sample extract can be expressed as a percentage reduction in DPPH. The amount of each spray- and freeze-dried colostrum sample, calculated using the following formula:
where ( is the absorbance of DPPH solution without sample at t = 0 min,
is the absorbance of DPPH solution with spray- and freeze-dried colostrum sample extract at t = 60 min. The reducing power of the sample extracts was evaluated by assessing their ability to convert ferric tri-pyridyltriazine into a blue-colored ferrous compound, detectable at 593 nm, following the method outlined by Jastrzebski et al.[Citation25] The FRAP reagent was prepared by combining 10 volumes of acetate buffer (300 mM at pH 3.6), 2.5 ml of 2,4,6-tripyridyl-s-triazine (TPTZ) solution (10 mM), and 1 volume of 20 mM ferric chloride (FeCl3), incubated at 25°C for 10 min. To assess the reducing power of the colostrum samples, 1.5 ml of the FRAP reagent was immediately mixed with 20 ml of the colostrum samples extract or standard. The absorbance was then measured at 593 nm using a UV/visible light spectrophotometer (Shimadzu UV-160A, Kyoto, Japan).
Where: Abs is the solvent of extract and b is the coefficient of the FRAP.
ABTS [2,2’-Azinobis(3-Ethylbenzothiazoline-6-Sulphonic Acid)] Free radical scavenging activity assay
The ABTS assay is a method used to assess antioxidant activity, as described by Salman et al.[Citation26] To prepare the ABTS radical, 5 ml of a 4.9 mM potassium persulfate solution (pH = 7.4) is added to 5 mL of a 14 mM ABTS solution and is left in the dark for 16 h. For the assay, in the 96-well microplate with 190 ml of ABTS and 10 ml of the prediluted sample following the inhibition period of 20 min. The absorbance is then measured at 734 nm using a UV-visible spectrophotometer (Shimadzu UV-160A, Kyoto, Japan) and compared with a control ABTS solution. A calibration curve is constructed using various concentrations of Trolox (50, 100, 150, 200, and 250 mmol/l). The ABTS radical scavenging activity is expressed as micro mol trolox equivalent antioxidant capacity (TEAC) per gram of the sample.
Microbial analysis
The spray- and freeze-dried bovine colostrum powder samples’ microbiological integrity evaluated as markers of cleanliness. Total plate count (TPC), total coliforms count (TCC), yeast and mold count (TYMC) and E. coli were enumerated as per the methods no. ISO 4833 1:2013. The amount of microorganism present was measured in log colony forming units (CFU) per milliliter (log10 CFU mL-1).[Citation27]
Instrumental color analysis
Spray- and freeze-dried samples of bovine colostrum were evaluated by instrumental color analysis using a Minolta Chromameter from Konica Minolta Co., Ltd., Osaka, Japan. The device was calibrated using a white calibration plate according to the provided protocol of instrument by manufacturer. The CIELab color scale was used to calculate the values of color parameters viz., L*, a*, and b *. The color scale CIELab for L* shows lightness from 0 to 100, the a* axis shows color from red to green, with while the b* axis indicates the transition from yellow to blue color.
Sensory evaluation of bovine colostrum samples
The evaluation of the acceptability of the effective formulation of the spray- and freeze-dried bovine colostrum powder samples took place on the same day the samples were prepared. This study followed a randomized design, where the spray- and freeze-dried bovine colostrum powder samples labeled with three-digit numerals were presented individually to participants. A hedonic scale of 9-points was used to assess the overall acceptance rate, the effectiveness of the spray- and freeze-dried bovine colostrum powder samples. The sensory parameters viz, color, aftertaste, flavor, mouth feel, texture, and overall acceptability (1 = dislike extremely and 9 = like extremely) were evaluated according to the method of Meilgaard et al.[Citation28]
Statistical analysis
The correlation between instrumental measurements and sensory properties using the Pearson Correlation Coefficient (r) was assessed. Principle Component Analysis (PCA) was employed with sensory parameters using statistical software. All the results obtained were analyzed using an analysis of variance (ANOVA) on IBM SPSS version 25 (IBM Corp., Armonk, NY, USA). The experimental data were presented as mean ± standard deviation (SD). Statistical significance was considered at a level of 5%. A value of p < .05 was considered significant, while p < .01 was considered highly significant.
Results and discussion
Chemical analysis of spray- and freeze-dried colostrum powder
The most beneficial component of colostrum is proteins. Lactoferrin, IgG, and IgA is a protein in colostrum, and its content is greater in colostrum. As shown in , there are wide variations in the protein values were ranged from 40.9 ± 0.7 to 30.8 ± 1.0 and 72.5 ± 1.7 to 68.1 ± 0.2 in spray and freeze dryer respectively. Similar research conducted by Pecka et al.[Citation29] and reported variations in protein content, with the highest levels observed in colostrum 15.21% and the lowest in milk during the week of lactation 2.05% and decreasing protein content was due to decreasing permeability of membrane of secretory cell. In the case of spray-drying, a greater denaturation of bioactive compounds occurred in comparison to freeze-drying, contributing to variations in protein levels.
Figure 1. Chemical analysis of bovine colostrum powder, percentages (%) of protein (a); and ash (b).
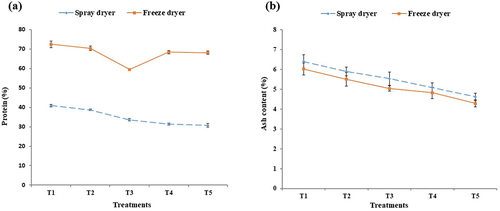
A considerable difference was seen in ash content under different treatment at p < .05. The ash content of several samples of spray-dryer ranges from 6.4 ± 0.3 to 4.6 ± 0.2 and 6.0 ± 0.3 to 4.3 ± 0.2 in freeze dryer. The maximum ash value was measured at T1 in spray-drying and minimum value was measured at T5 in freeze-drying which includes metal salts and trace minerals (). According to observations, the ash value of all samples decreased as water concentration increased. Rashid et al.[Citation30] investigated that the ash contents of colostrum decrease with the passage of time up to 3 days. The ash percentage decreases from 1.05% w/v to 0.87% w/v. Third day’s colostrum ash content becomes equal to the ash content of milk, according to resultant value the ash content of colostrum also decreases due to the addition of water.
There was a significant variation in total solid under different treatments at p < .05. As for the total solid content, spray and freeze dryer samples generally contain 92.6 ± 0.1 to 90.6 ± 0.3 and 94.2 ± 0.3 to 92.6 ± 0.2, respectively. The total solid value was present in the largest amount in freeze-drying with 94.2%. According to observations, the total solid value of all samples decreased as water concentration increased as shown in . The similar study was conducted by Zahraddeen et al.[Citation31] on the total solids, the breed effect was statistically significant (p ≤.05). There was decrease in total solid with the breed, and in colostrum there was decrease in total solid due to the addition of water.
Figure 2. Chemical analysis of bovine colostrum powder, percentages (%) of total solids (a); fat (b); and moisture (c).
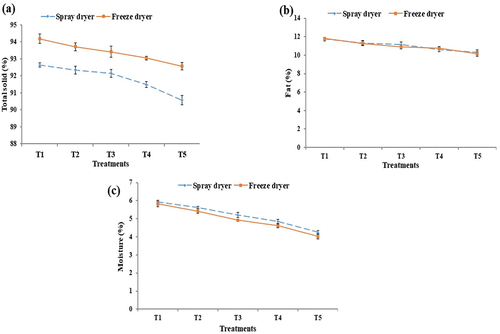
The amount of fat was significantly different depending on the treatment viz., spray and freeze-drying method. Significant variation was observed in fat under different treatments at p < .05. The fat content of several samples ranges from 11.7 ± 0.2 to 10.2 ± 0.2 in spray-dryer a nd from 11.8 ± 0.1 to 10.1 ± 0.2 in freeze dryer as shown in . Resembled with some previous study with Addis et al.[Citation32] compared to mature goat milk, colostrum had lower proportions of fatty acids, triglycerides, phospholipids, cholesterol, and its ester, which contributed to the lower fat content, and in the resultant value of colostrum powder, there was decrease in fat value when water was added.
Moisture content in colostrum powders is commonly measured using a moisture analyzer. Moisture content of the samples was significantly different among treatments at p < .05. The moisture content of several samples ranges from 5.9 ± 0.1 to 4.2 ± 0.1 in spray-dryer, while the moisture values were 5.8 ± 0.1, 4.0 ± 0.1 in freeze dryer (). The maximum moisture value was measured at T1 in freeze-drying and minimum value was measured at T5. The moisture values were 5.4 ± 0.1, 4.9 ± 0.06, and 4.6 ± 0.1 at T2, T3, and T4 treatment after being treated with freeze-drying. The low content of moisture in the samples suggested that, they have high non-perishability property. Similar study was conducted by Habtegebriel et al.[Citation33] in which colostrum powders were used, and observed that moisture content generally correlates with the outlet temperature. Moisture was lower with lower outlet temperature and higher with higher outlet temperature, therefore resultant colostrum powder moisture decreases due to the spray- and freeze-drying temperature.
The amount of IgG and IgA was significantly different depending on the treatments at p < .05 as shown in . The IgG content of several samples in spray and freeze dryer ranges from 42.1% ± 0.9% to 31.6% ± 1.1 and from 43.8% ± 0.4% to 32.8% ± 0.3, respectively. On the other hand, the percentage of IgA content of several colostrum powder samples in spray dryer ranges from 3.3 ± 0.1 to 2.3 ± 0.2, while in freeze dryer from 3.6% ± 0.2% to 2.4% ± 0.1, respectively (). All the results suggested that adding additional water to colostrum reduces its IgG and IgA concentration, same research was carried out by Mistry et al.[Citation34], Vermeer & Norde[Citation35] decrease of IgG and IgA contents of colostrum with the passage of temperature and time up to 3 days.
Figure 3. Chemical analysis of bovine colostrum powder, percentages (%) of IgG (a); IgA (b); and lactose (c).
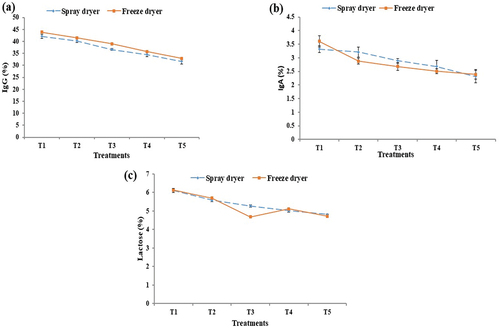
The lactose content of several samples of colostrum powder ranges from 6.1% ± 0.1% to 4.8% ± 0.1 in spray dryer as shown in . The lactose values were 6.1% ± 0.3 to 4.7% ± 0.2 in freeze dryer. The maximum lactose value was measured at T1 and minimum value was measured at T5 in both spray- and freeze-drying. A similar investigation was carried out by Mehra et al.[Citation36] colostrum is more viscous than milk because of the low lactose content, which distinguishes it from milk. In this study, the amount of lactose detected in colostrum was shown to be as low as 1.2 g. Other studies have also noted that during times of heat stress, the blood’s concentration of non-esterified fatty acids and the generation of hepatic glucose both decreased, which resulted in a lesser supply of glucose to the mammary glands and a lower lactose output.
Overall, results showed that in comparison to spray-dried colostrum, freeze-dried colostrum had significantly increased in protein, total solid, IgG, and IgA contents. There was slightly increased in fat, moisture, ash, lactose value of spray-dryer rather than freeze one. The results, however, were comparable to earlier findings of published results El-Fattah et al.[Citation37], Abdel-Ghany & Zaki.[Citation38]
Bovine colostrum powder yield
shows the bovine colostrum powder yield obtained from spray- and freeze-drying methods. Based on , it could be seen that the bovine colostrum powder yield obtained from freeze-drying was the highest from T1 (83.67% ± 1.27) to T5 (79.8% ± 0.65), respectively, while the yield was obtained by spray-drying at T1 (82.93% ± 1.65), and T5 (69.94% ± 0.65). A very low yield by spray-drying was calculated at T4 (47.88% ± 0.53).
In vitro evaluation of anti-oxidant content
Total phenolic compound (TPC)
Bovine colostrum made from feed contains sizable levels of phenolic compounds, which are good sources of natural antioxidants in human diets. The TPC content in spray and freeze dryer bovine colostrum samples ranges from 62 mg GAE/g ±1 to 49.3 mg GAE/g ±0.8 and 22.1 mg GAE/g ±0.9 to 18.5 mg GAE/g ±0.6, respectively. The T1 sample showed the highest values of TPC when compared to those for T2, T3, T4, and T5 treatments both in spray and freeze dryer samples as mentioned in . The findings were comparable with Yilmaz et al.[Citation39] the TPC in the samples of cow and ewe kefir varied from 59.1 to 85.7 mg of GAE/100 mL and 77.7 to 84.8 mg of GAE/100 mL, respectively. Kefir milk with commercial starter culture added had slightly higher TPC values than milk with kefir grains. On day 7, C-SC and C-GR’s TPC values both decreased.
Table 2. Total phenolic contents and in vitro antioxidant contents of bovine colostrum samples.
Free radical scavenging property of spray- and freeze-drying samples of bovine colostrum
Diphenyl-1-picrylhydrazyl a stable dark-purple, crystalline powder of organic-free radical (DPPH) demonstrates the antioxidants’ ability to give hydrogen or electrons by scavenging proton-radicals. The DPPH content of several samples ranges from 5.38% ± 0.14% to 4.24% ± 0.13 in spray dryer. The DPPH value was 9.22% ± 0.31% to 7.23% ± 0.34 in freeze dryer. The T1 sample showed the highest values of TPC when compared to those for T2, T3, T4, and T5 treatments both in spray and freeze dryer samples as mentioned in . A comparable investigation was conducted by Qureshi et al.[Citation40] the DPPH values of colostrum were significantly affected by postpartum milking (p ≤ 05). Significant quantities of DPPH (2199–2661 mol) were discovered to exist up until the conclusion of the third postpartum milking, but after that, values dropped.
Confirmation of radical scavenging property
Ferric reducing anti-oxidant power the FRAP assay is a straightforward, practical, and repeatable procedure that reduced Fe3+ to Fe2+ in the presence of ferrictripyridyltriazine (TPTZ) complex to produce a blue color, which was used to assess the antioxidative activity of the spray- and freeze-drying samples of bovine colostrum. The reducing powers of different treatments are shown in . The highest value of reducing power shows stronger antioxidant properties of the treated samples. In the present research work, we determined that the FRAP content of spray- and freeze-drying several samples of bovine colostrum ranges from 8.12 mM Fe2+/g ±0.09 to 7.11 mM Fe2+/g ±0.09 and from 1.14 mM Fe2+/g ±0.06 to 0.26 mM Fe2+/g ±0.02, respectively. The T1 sample had a higher value, whereas the T5 sample had a lower value in spray dryer as mentioned in Table 4.5. The reducing power was significantly higher (p > .05) at T1 and minimum at T5 both in spray and freeze dryer sample treatments. So, these results also stated that by adding the more water in colostrum there is a decrease in the concentration of FRAP. Similar findings were also reported in Tu et al.[Citation41] on losses of bioactive components and reduce FRAP index in various colostrum samples during heat treatment.
Antioxidant activity using radical cationic ABTS+ scavenging property
The ABTS method was used to gauge the sample’s capacity to smother a radical. This technique can exert many substances, including trolox, urate, ascorbate, cysteine, glutathione, and bilirubin. The ABTS content of several samples ranges from 11.21 mM ± 0.06 to 10.31 mM ± 0.05 in spray dryer. The T1 sample had a higher value, whereas the T5 sample had a lower value in spray dryer as mentioned in . The ABTS value was 11.09 mM ±0.11 to 9.78 mM ±0.13 in freeze dryer. The maximum ABTS value was measured at T1 in freeze-drying, and minimum value was measured at T5. A comparable investigation with Erel[Citation42] when the acetate buffer with the highest pH value was employed, the results showed the maximum decrease rate. Acetate buffer’s ideal pH for the production of ABTS+ was 3.6, while for the reduction procedures was 5.8.
According to overall results of in vitro anti-oxidant contents, spray- and freeze-dried colostrum in Table 4.3 displayed a variance by treatment. According to findings, spray drying is more effective than freeze-drying at increasing the values of FRAP, ABTS, and TPC, whereas freeze-drying increases the value of DPPH. Numerous studies have also claimed the same (Suetsuna et al.[Citation43]; Correia et al.[Citation44]).
:10%
+90% Colostrum;
: 20%
+80% Colostrum;
: 30%
+70% Colostrum;
: 40%
+60% Colostrum;
: 50%
+50% Values are mean ± SE (n = 3). Means sharing similar letter in a row or in a column are statistically non-significant (p > .05).
Microbiological evaluation of different treatments of spray and freeze dryer samples of bovine colostrum powder
Total coliform count (TCC)
Total coliform count (TCC) is a crucial parameter used to evaluate the microbial quality of colostrum. TCC is used as an indicator of hygiene and potential contamination in bovine colostrum. The TCC content of several samples of spray- and freeze-drying ranges from 0.41 ± 0.02 to 0.58 ± 0.01 and from 0.52 ± 0.03 to 0.93 ± 0.05, respectively. As shown in , the minimum TCC value was measured at T1 and maximum value was measured at T5 both in spray and in freeze-drying treatments. The study of Johnson et al.[Citation45] and the studies reported previously have done similar work. Two groups of raw bovine colostrum and heat-treated colostrum were compared. At the time of feeding, total coliform count increased in both groups but after heat treating procedure the value of TCC in heat treated colostrum decreased.
Table 3. Microbial analysis of spray- and freeze-drying bovine colostrum powder samples.
Total plate count (TPC)
Total plate count refers to microbiological testing method used to estimate the total number of viable bacteria present in sample. In bovine colostrum, TPC analysis can be conducted to assess its microbial quality and potential safety for consumption. In spray dryer, TPC content of several samples of bovine colostrum ranges from 0.27 ± 0.02 to 0.46 ± 0.03, while in freeze dryer the TPC value was 0.72 ± 0.13 to 0.98 ± 0.01, respectively (). The same work was done in the previous study by Elizondo-Salazar et al.[Citation46] In this study, different temperature was taken to kill the bacterial group in comparison to bacterial counts in untreated colostrum, and numbers for all categories of bacteria decreased as time and temperature rose.
Escherichia coli (E. coli)
The presence of E. coli in bovine colostrum is an indicator of poor hygiene practices during collection, storage, and handling. The E. coli content of several samples ranges from 0.51 ± 0.02 to 0.68 ± 0.02 in spray dryer. The E. coli value was 0.52 ± 0.03 to 0.95 ± 0.03 in the freeze dryer. The minimum E. coli value was measured at T1 in freeze-drying and maximum value was measured at T5. According to observations, the E. coli value of all samples increased as water concentration increased as shown in . Similar results were observed in this study conducted Sotudeh et al.[Citation47] Four different treatments were used to lower the amount of E. coli in the colostrum, but freeze and spray were always more successful.
Yeast and Mold (Y and M)
The presence of yeast and mold can indicate the presence of contamination. Yeast and mold are type of fungi, some are harmless and some cause spoilage and illness. The Y and M content of several samples ranges from 0.21 ± 0.02 to 0.34 ± 0.04 in spray dryer. The T1 sample had a lower value, whereas the T5 sample had a higher value in spray dryer as mentioned in Table 4.4. The Y and M value was 0.14 ± 0.01 to 0.26 ± 0.02 in freeze dryer. There is no difference between this study and the earlier ones Godden et al.[Citation48] After studying various methods to kill bacteria and fungi, they noticed that after 30 min of pasteurization, the amount of mold and yeast was less than 1 log. According to the investigation, showed that spray- and freeze-dried bovine colostrum showed a treatment difference. The results indicate, spray-drying is more successful at raising the values of TCC, TPC, E. coli, Y and M rather than freeze-drying. Numerous studies made the same claim Borad et al.[Citation49]; Hesami et al.[Citation50]; Hayes et al.[Citation51]
:10%
+90% Colostrum;
: 20%
+80% Colostrum;
: 30%
+70% Colostrum;
: 40%
+60% Colostrum;
: 50%
+50% Values are mean ± SE (n = 3). Means sharing similar letter in a row or in a column are statistically non-significant (p > .05).
Sensory evaluation
Sensory evaluation of bovine colostrum that has been processed using spray- and freeze-drying involves assessing various attributes related to appearance, aroma, and flavor. The appearance value peaked at 6.11 ± 0.21 and low at 3.93 ± 0.11 in the spray dryer and as for freeze dryer the appearance value was 7.08 ± 0.12 to 5.28 ± 0.21, respectively. In aroma smell was noticed, the inhaled aroma of the dried colostrum notes any changes in aroma compared to fresh colostrum. The aroma value in spray and freeze dryer peaked at 6.38 ± 0.19 and low at 4.64 ± 0.12 and from 5.69 ± 0.07 to 3.82 ± 0.15, respectively. Taste the dried colostrum was tasted and evaluated the flavor profile. In all attributes, the T1 sample had a higher value, whereas the T5 sample had a lower value in both spray- and freeze-drying colostrum powder as shown in . Same study conducted by Goyal et al.[Citation52] and Nazir et al.[Citation53]
Figure 5. Effect of spray- and freeze-drying in different treatments on sensorial properties of bovine colostrum.
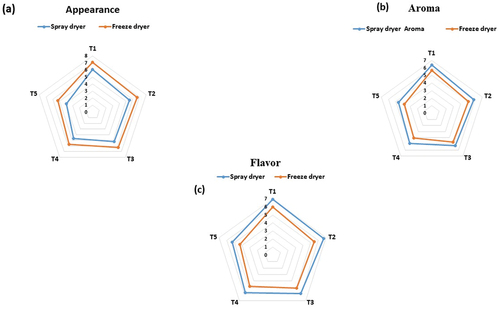
Research revealed a treatment difference between the spray- and freeze-dried colostrum in Table 4.6. According to research, spray-drying is more effective than freeze-drying at increasing the values of aroma and flavor and the value of appearance is increased in freeze dryer. The same claim was made in numerous studies Desai et al.[Citation54], Hayes et al.[Citation51] they took a sample of fermented colostrum product with different milk quantities and showed a decreasing trend as the proportion of milk increased.
Confirmation of color analysis
The color of colostrum samples dried in spray and freeze dryer is very important quality parameter as for consumer acceptability. Detailed results from the instrumental color analysis are provided in . Results revealed that spray- and freeze-drying treatments statistically did not have a significant (p > .05) effect on the color parameters. The L* values of all samples showed the lightness in spray- and freeze-dried samples of bovine colostrum. The a* values, with all treatments of spray- and freeze-dried bovine colostrum samples showed that red color is dominating over the red in all treated samples from T1 to T5. The b* values, with all treatments with positive value, confirm that the yellow coloration is dominating over the blue of all samples of spray and freeze-dried bovine colostrum. The possible explanation for the decrease in lightness, yellowness, and greenness is because of the low dry matter content of spray- and freeze-dried samples of bovine colostrum. Similar results were depicted by Yilmaz-Ersan and Topcuoglu.[Citation55]
Table 4. The color values of spray- and freeze-dried bovine colostrum.
Pearson’s correlation coefficients in all variables of instrumental measurements and sensory parameters are shown in . Protein was significantly positively correlated (p < .05) with total solids (r = 84), redness (r = 72), TCC (r = 65), DPPH (r = 96) and one of the sensorial parameter i.e., appearance (r = 73). Ash was significantly positively correlated with fat, IgG, IgA, moisture, lactose, yellowness, ABTS, aroma, and flavor, while negatively correlated with TCC and E. coli r = −0.79, r = −0.85, respectively, at p < .05. Total solids were also significantly correlated with IgG (r = 0.76), redness (r = 0.93), yield (r = 0.65), DPPH (r = 0.91) and appearance (r = 0.97). A significant positive correlation among fat, IgG, IgA, moisture, and sensorial parameters were observed as shown in . As for microbial analysis, the values for TCC and E. coli showed a significant negative correlation with TPC (r = −0.81, r = −0.68, p < .05) FRAP (r = −0.81, r = −0.68, p < .05), ABTS (r = −0.88, r = −0.89, p < .05) and aroma (r = −0.91, r = −0.94, p < .05) as well as flavor (r = −0.94, r = −0.93, p < .05), respectively. The nonlinear correlations observed in these parameters may be due to disproportions between the oral perception process and the instrumental methodology employed.
Table 5. Pearson’s correlation coefficients in all variables of instrumental measurements and sensory attributes.
The Principal Component Analysis revealed (), that the first component (PC1) explained 54.32% of the total variance, while the second one (PC2) explained 35.93% of the data variation. The spray-dried samples were grouped in the upper quadrants, while the freeze-dried samples were located in the bottom of the graphic. PC1 was associated with ash, moisture, IgA, lactose, fat, yellowness, IgG, while PC2 was associated with protein, DPPH, FRAP, TPC. Significant correlations (p < .05) were observed between some of the chemical and sensory and color characteristics. PC1 highlights opposition between Y and M and E-coli and TCC, FRAP and protein, Flavor and appearance, while PC2 underscores oppositions between Y and M and total solid, E-coli and flavor, TCC and Aroma.
Conclusion
Colostrum powder has been proven effective in adults and children with vital nutrients, antibodies, and growth factors. Both techniques, spray- and freeze-drying, were beneficial for colostrum. The proximate, antioxidant, microbial, and sensory analyses were done at different treatments. The high values were observed at 10% water and 90% colostrum, and the minimum value was observed at 50% water and 50% colostrum treatment except microbial analysis in which the minimum value was observed at l0% water and 90% colostrum due to the less addition of water. A similar trend was observed in antioxidant analysis and sensory analysis at the same treatment level. Protein, total solid, IgG, and IgA were higher in the freeze-drying technique, and fat, lactose, ash, and moisture were higher in spray-drying. In antioxidant analysis, the same treatment was done. Antioxidants showed a maximum value of 10% colostrum and 90% water and a minimum value of 50% colostrum and 50% water. In spray-drying, the value of FRAP, ABTS, and TPC was at a higher rate than in the freeze dryer, and the value of DPPH was higher in the freeze-drying technique. In microbial analysis, the minimum value was found at 10% water and 90% colostrum, and a higher value was observed at 50% water and 50% colostrum due to the more water addition. Spray drying shows less microbial growth than freeze-drying. In sensory analysis, appearance was more stable in freeze-drying, while aroma and flavor were stable in spray-drying. The correlations analysis was assessed between instrumental characterization and sensory evaluation. The arrangement of variables in PCA plots revealed distinct properties for potential commercial or industrial applications of the spray- and freeze-dried colostrum samples. According to the results, the conclusion was that freeze-drying is more effective and stable for the bioactive component of bovine colostrum. Further studies are necessary to explore the novel applications of bovine colostrum in the food industry, including the development of dietary supplements, fortified functional foods, and therapeutic formulations that utilize the preserved bioactive components of dried colostrum.
Acknowledgments
The authors acknowledge support from the Department of Food Science, GCUF, for carrying out all research activities. This project was supported by Researchers Supporting Project Number [RSP2025R5] King Saud University, Riyadh, Saudi Arabia.
Disclosure statement
No potential conflict of interest was reported by the author(s).
Data availability statement
The authors confirm that the data supporting the findings of this study are available within the article.
Additional information
Funding
References
- Bagwe, S.; Tharappel, L. J.; Kaur, G.; Buttar, H. S. Bovine Colostrum: An Emerging Nutraceutical. J. Complement. Integr. Med. 2015, 12(3), 175–185. DOI: 10.1515/jcim-2014-0039.
- Marchbank, T.; Davison, G.; Oakes, J. R.; Ghatei, M. A.; Patterson, M.; Moyer, M. P.; Playford, R. J. The Nutriceutical Bovine Colostrum Truncates the Increase in Gut Permeability Caused by Heavy Exercise in Athletes. Am. J. Physiol.-Gastrointestinal Liver Physiol. 2011, 300(3), G477–G484. DOI: 10.1152/ajpgi.00281.2010.
- Parshina, V. I.; Notina, E. A.; Petrukhina, O. A.; Terekhin, A. A.; Voronina, J.; Bondareva, I. V.; Zueva, E. A. Diagnosis of the Nutritional Value of Colostrum in Cows of Different Milk Yield. Int. J. Adv. Biotechnol. Res. 2019, 10(2), 157–172.
- Steele, J.; Sponseller, J.; Schmidt, D.; Cohen, O.; Tzipori, S. Hyperimmune Bovine Colostrum for Treatment of GI Infections: A Review and Update on Clostridium Difficile. Hum. Vaccines Immunotherapeutics. 2013, 9(7), 1565–1568. DOI: 10.4161/hv.24078.
- Shah, N. P. Probiotic Bacteria: Selective Enumeration and Survival in Dairy Foods. J. Dairy Sci. 2000, 83(4), 894–907. DOI: 10.3168/jds.S0022-0302(00)74953-8.
- Godhia, M. L.; Patel, N. Colostrum–Its Composition, Benefits as a Nutraceutical–A Review. Curr. Res. Nutr. Food Sci. 2013, 1(1), 37–47. DOI: 10.12944/CRNFSJ.1.1.04.
- Mero, A.; Nykänen, T.; Keinänen, O.; Knuutinen, J.; Lahti, K.; Alen, M.; Rasi, S.; Leppäluoto, J. Protein Metabolism and Strength Performance After Bovine Colostrum Supplementation. Amino Acids. 2005, 28(3), 327–335. DOI: 10.1007/s00726-005-0179-8.
- Kehoe, S. I.; Jayarao, B. M.; Heinrichs, A. J. A Survey of Bovine Colostrum Composition and Colostrum Management Practices on Pennsylvania Dairy Farms. J. Dairy Sci. 2007, 90(9), 4108–4116. DOI: 10.3168/jds.2007-0040.
- Stelwagen, K.; Carpenter, E.; Haigh, B.; Hodgkinson, A.; Wheeler, T. T. Immune Components of Bovine Colostrum and Milk. J. Anim. Sci. 2009, 87(suppl_13), 3–9. DOI: 10.2527/jas.2008-1377.
- Kumar, H.; Kumar, N.; Seth, R.; Goyal, A. Chemical and Immunological Quality of Goat Colostrum: Effect of Breed and Milking Frequency. Indian J. Dairy Sci. 2014, 67(6), 482–486.
- Mehra, R.; Singh, R.; Kumar, N.; Kumar, S.; Kumar, H. Composition, Properties and Health Attributes of Bovine Colostrum. UGC Sponsored National Conference On: Food Safety, Nutritional Security and Sustainability. New Delhi, Mar, 2020.
- Gaiani, C.; Morand, M.; Sanchez, C.; Tehrany, E. A.; Jacquot, M.; Schuck, P.; Scher, J.; Scher, J. How Surface Composition of High Milk Proteins Powders Is Influenced by Spray-Drying Temperature. Colloids And Surfaces B: Biointerfaces 2010, 75(1), 377–384. DOI: 10.1016/j.colsurfb.2009.09.016.
- Sahana, N.; Ramasamy, D.; Pugazhenthi, T. R.; Manikkavasagan, I. Studies on Physical and Microbial Parameters of Bovine Colostrum Powder. Int. J. Curr. Microbiol. Appl. Sci. 2018, 7(8), 2766–2771. DOI: 10.20546/ijcmas.2018.708.290.
- Borad, S. G.; Singh, A. K.; Meena, G. S.; Raghu, H. V. Storage Related Changes in Spray Dried Colostrum Preparations. LWT 2020, 118, 108719.
- Martysiak-Żurowska, D.; Rożek, P.; Puta, M. The Effect of Freeze-Drying and Storage on Lysozyme Activity, Lactoferrin Content, Superoxide Dismutase Activity, Total Antioxidant Capacity and Fatty Acid Profile of Freeze-Dried Human Milk. Drying Technol. 2022, 40(3), 615–625. DOI: 10.1080/07373937.2020.1824188.
- Zouari, A.; Briard-Bion, V.; Schuck, P.; Gaucheron, F.; Delaplace, G.; Attia, H.; Ayadi, M. A. Changes in Physical and Biochemical Properties of Spray Dried Camel and Bovine Milk Powders. LWT 2020, 128, Article 109437.10.1016/j.lwt.2020.109437.
- Salar, S.; Jafarian, S.; Mortazavi, A.; Nasiraie, L. R. Effect of Hurdle Technology of Gentle Pasteurisation and Drying Process on Bioactive Proteins, Antioxidant Activity and Microbial Quality of Cow and Buffalo Colostrum. Int. Dairy J. 2021, 121, 105138. DOI: 10.1016/j.idairyj.2021.105138.
- Rahim, M. A.; Imran, M.; Khan, M. K.; Ahmad, M. H.; Ahmad, R. S. Impact of Spray Drying Operating Conditions on Encapsulation Efficiency, Oxidative Quality, and Sensorial Evaluation of Chia and Fish Oil Blends. J. Food Process. Preserv. 2022, 46(2), e16248. DOI: 10.1111/jfpp.16248.
- Zhang, Y.; Zheng, Z.; Liu, C.; Tan, C. P.; Xie, K.; Liu, Y. A Comparative Study Between Freeze-Dried and Spray-Dried Goat Milk on Lipid Profiling and Digestibility. Food Chem. 2022, 387, 132844. DOI: 10.1016/j.foodchem.2022.132844.
- Aburjaile, F. F.; Rohmer, M.; Parrinello, H.; Maillard, M. B.; Beaucher, E.; Henry, G.; Falentin, H.; Madec, M.-N.; Thierry, A.; Parayre, S.; Deutsch, S.-M. Adaptation of Propionibacterium freudenreichii to Long-Term Survival Under Gradual Nutritional Shortage. BMC Genomics 2016, 17(1), 1–13. DOI: 10.1186/s12864-016-3367-x.
- Lee, S. Y.; Ferdinand, V.; Siow, L. F. Effect of Drying Methods on Yield, Physicochemical Properties, and Total Polyphenol Content of Chamomile Extract Powder. Front. Pharmacol. 2022, 13, 1003209. DOI: 10.3389/fphar.2022.1003209.
- Bayili, R. G.; Abdoul-Latif, F.; Kone, O. H.; Diao, M.; Bassole, I. H.; Dicko, M. H. Phenolic Compounds and Antioxidant Activities in Some Fruits and Vegetables from Burkina Faso. Afr. J. Biotechnol. 2011, 10(62), 13543–13547. DOI: 10.5897/AJB10.2010.
- Madureira, J.; Severino, A.; Cojocaru, M.; Garofalide, S.; Santos, P. M. P.; Carolino, M. M.; Verde, S. C.; Cabo Verde, S. E-Beam Treatment to Guarantee the Safety and Quality of Cherry Tomatoes. Innova Food Sci & Emerg Tech. 2019, 55, 57–65. DOI: 10.1016/j.ifset.2019.05.013.
- Oussaief, O.; Jrad, Z.; Adt, I.; Dbara, M.; Khorchani, T.; El‐Hatmi, H. Antioxidant Activities of Enzymatic‐Hydrolysed Proteins of Dromedary (Camelus dromedarius) Colostrum. Int. J. Dairy Technol. 2020, 73(2), 333–340. DOI: 10.1111/1471-0307.12668.
- Zarban, A.; Taheri, F.; Chahkandi, T.; Sharifzadeh, G.; Khorashadizadeh, M. Antioxidant and Radical Scavenging Activity of Human Colostrum, Transitional and Mature Milk. J. Clin. Biochem. Nutr. 2009, 45(2), 150–154. DOI: 10.3164/jcbn.08-233.
- Salman, S.; Dinse, D.; Khol-Parisini, A.; Schafft, H.; Lahrssen-Wiederholt, M.; Schreiner, M.; Scharek-Tedin, L.; Zentek, J. Colostrum and Milk Selenium, Antioxidative Capacity and Immune Status of Dairy Cows Fed Sodium Selenite or Selenium Yeast. Arch. Animal Nutr. 2013, 67(1), 48–61. DOI: 10.1080/1745039X.2012.755327.
- E, I. S. O. Microbiology of the Food Chain–Horizontal Method for the Enumeration of Microorganisms–Part 1: Colony Count at 30 Degrees C by the Pour Plate Technique. ISO Norm. 2013, 4833, 1–2013.
- Meilgaard, M.; Vance Civille, G.; Carr, T. Sensory Evaluation Techniques, 4th ed.; CRC Press: Boca Raton, FL, 2007; pp. 7–8 & 173–179.
- Pecka, E.; Dobrzański, Z.; Zachwieja, A.; Szulc, T.; Czyż, K. Studies of Composition and Major Protein Level in Milk and Colostrum of Mares. Anim. Sci. J. 2012, 83(2), 162–168. DOI: 10.1111/j.1740-0929.2011.00930.x.
- Rashid, A. A.; Yousaf, M.; Salaryia, A. M.; Ali, S. Studies on the Nutritional Composition of Goat (Beetal) Colostrum and Its Mature Milk. Pak. J. Biochem. Mol. Biol 2012, 45(3), 113–116.
- Zahraddeen, D.; Butswat, I. S. R.; Mbap, S. T. Evaluation of Some Factors Affecting Milk Composition of Indigenous Goats in Nigeria. Livestock Res. Rural Dev. 2007, 19(11), 166.
- Addis, M.; Cabiddu, A.; Pinna, G.; Decandia, M.; Piredda, G.; Pirisi, A.; Molle, G. Milk and Cheese Fatty Acid Composition in Sheep Fed Mediterranean Forages with Reference to Conjugated Linoleic Acid Cis-9, Trans-11. J. Dairy Sci. 2005, 88(10), 3443–3454. DOI: 10.3168/jds.S0022-0302(05)73028-9.
- Habtegebriel, H.; Edward, D.; Wawire, M.; Sila, D.; Seifu, E. Effect of Operating Parameters on the Surface and Physico-Chemical Properties of Spray-Dried Camel Milk Powders. Food Bioprod. Process. 2018, 112, 137–149.
- Mistry, J.; Chuguransky, S.; Williams, L.; Qureshi, M.; Salazar, G. A.; Sonnhammer, E. L.; Bateman, A.; Paladin, L.; Raj, S.; Richardson, L. J. Pfam: The Protein Families Database in 2021. Nucleic Acids Res. 2021, 49(D1), D412–D419. DOI: 10.1093/nar/gkaa913.
- Vermeer, A. W.; Norde, W. The Thermal Stability of Immunoglobulin: Unfolding and Aggregation of a Multi-Domain Protein. Biophys. J. 2000, 78(1), 394–404. DOI: 10.1016/S0006-3495(00)76602-1.
- Mehra, R.; Singh, R.; Nayan, V.; Buttar, H. S.; Kumar, N.; Kumar, S.; Bhardwaj, A.; Kaushik, R.; Kumar, H. Nutritional Attributes of Bovine Colostrum Components in Human Health and Disease: A Comprehensive Review. Food Biosci. 2021, 40, 100907. DOI: 10.1016/j.fbio.2021.100907.
- Abd El-Fattah, A. M.; Abd Rabo, F. H.; El-Dieb, S. M.; El-Kashef, H. A. Effect of Pre-Weaning Concentrate Supplementation on Peripheral Distribution of Leukocytes, Functional Activity of Neutrophils, Acute Phase Protein and Behavioural Responses of Abruptly Weaned and Housed Beef Calves. BMC Vet. Res. 2012, 8, 1–7. DOI: 10.1186/1746-6148-8-1.
- Abdel-Ghany, A. S.; Zaki, D. A. Production of Novel Functional Yoghurt Fortified with Bovine Colostrum and Date Syrup for Children. Alexandria Sci. Exch. J 2018, 39(2018), 651–662. DOI: 10.21608/asejaiqjsae.2018.20475.
- Yilmaz-Ersan, L.; Ozcan, T.; Akpinar-Bayizit, A.; Sahin, S. Comparison of Antioxidant Capacity of Cow and Ewe Milk Kefirs. J. Dairy Sci. 2018, 101(5), 3788–3798.
- Qureshi, T. M.; Yaseen, M.; Nadeem, M.; Murtaza, M. A.; Munir, M. Physico–Chemical Composition and Antioxidant Potential of Buffalo Colostrum, Transition Milk, and Mature Milk. J. Food Process. Preserv. 2020, 44(10), e14763. DOI: 10.1111/jfpp.14763.
- Tu, Y.; Xu, Y.; Ren, F.; Zhang, H. Characteristics and Antioxidant Activity of Maillard Reaction Products from α-Lactalbumin and 2′-Fucosyllactose. Food Chem. 2020, 316, 126341. DOI: 10.1016/j.foodchem.2020.126341.
- Erel, O. A Novel Automated Direct Measurement Method for Total Antioxidant Capacity Using a New Generation, More Stable ABTS Radical Cation. Clin. Biochem. 2004, 37(4), 277–285. DOI: 10.1016/j.clinbiochem.2003.11.015.
- Suetsuna, K.; Ukeda, H.; Ochi, H. Isolation and Characterization of Free Radical Scavenging Activities Peptides Derived from Casein. J. Nutr. Biochem. 2000, 11(3), 128–131.
- Correia, I.; Nunes, A.; Duarte, I. F.; Barros, A.; Delgadillo, I. Sorghum Fermentation Followed by Spectroscopic Techniques. Food Chem. 2005, 90(4), 853–859. DOI: 10.1016/j.foodchem.2004.05.060.
- Johnson, J. L.; Godden, S. M.; Molitor, T.; Ames, T.; Hagman, D. Effects of Feeding Heat-Treated Colostrum on Passive Transfer of Immune and Nutritional Parameters in Neonatal Dairy Calves. J. Dairy Sci. 2007, 90(11), 5189–5198.
- Elizondo-Salazar, J. A.; Jayarao, B. M.; Heinrichs, A. J. Effect of Heat Treatment of Bovine Colostrum on Bacterial Counts, Viscosity, and Immunoglobulin G Concentration. J. Dairy Sci. 2010, 93(3), 961–967. DOI: 10.3168/jds.2009-2388.
- Sotudeh, S.; Rabbani Khorasgani, M.; Etemadifar, Z.; Zarkesh-Esfahani, S. H. Effects of Spray-Drying, Freeze-Drying and Pasteurization on Microbiological Quality and IgG Level of Bovine Colostrum. J. Food Qual. Hazards Control. 2018, 5(2), 54–60. DOI: 10.29252/jfqhc.5.2.5.
- Godden, S.; McMartin, S.; Feirtag, J.; Stabel, J.; Bey, R.; Goyal, S.; Metzger, L.; Fetrow, J.; Wells, S.; Chester-Jones, H. Heat-Treatment of Bovine Colostrum. II: Effects of Heating Duration on Pathogen Viability and Immunoglobulin G. J. Dairy Sci. 2006, 89(9), 3476–3483. DOI: 10.3168/jds.S0022-0302(06)72386-4.
- Borad, S. G.; Singh, A. K.; Meena, G. S.; Arora, S.; Raju, P. N.; Sabikhi, L. Optimization of Spray Drying of Colostrum Protein Ingredients–A Rheological Approach. J. Food Eng. 2021, 288, 110247. DOI: 10.1016/j.jfoodeng.2020.110247.
- Hesami, S.; Shahraki, A. D. F.; Zakian, A.; Ghalamkari, G. The Effect of Various Heat-Treatment Methods on Colostrum Quality, Health and Performance of Dairy Calves. Acta Scientiarum. Anim. Sci. 2020, 43, e49844. DOI: 10.4025/actascianimsci.v43i1.49844.
- Hayes, M. M.; Hughes, T. A.; Greene, A. K. Bacterial Diversity in Dried Colostrum and Whey Sold as Nutraceutical Products. J. Food Sci. 2012, 77(7), M359–M363. DOI: 10.1111/j.1750-3841.2012.02771.x.
- Goyal, G. K.; Ahuja, K. K.; Sumit, G. Manufacture of Paneer Tikka. Indian Dairyman. 2010, 62(9), 56–60.
- Nazir, T.; Pal, M. A.; Manzoor, A. Effect of Admixing Varying Levels of Whole Milk to the Colostrum on the Sensory Quality of Fermented Colostrum Product. Int. J. Adv. Res. Sci. Eng. Technol. 2018, 7(4), 156–161.
- Desai, N. T.; Shepard, L.; Drake, M. A. Sensory Properties and Drivers of Liking for Greek Yogurts. J. Dairy Sci. 2013, 96(12), 7454–7466.
- Yilmaz-Ersan, L.; Esra, T. Evaluation of Instrumental and Sensory Measurements Using Multivariate Analysis in Probiotic Yogurt Enriched with Almond Milk. J. Food Sci. Technol. 2022, 59(1), 133–143. DOI: 10.1007/s13197-021-04994-w.